DOI:
10.1039/C8RA07491G
(Paper)
RSC Adv., 2018,
8, 40941-40949
Chemical constituents and antimicrobial activity of wood vinegars at different pyrolysis temperature ranges obtained from Eucommia ulmoides Olivers branches†
Received
8th September 2018
, Accepted 27th November 2018
First published on 6th December 2018
Abstract
Pyrolysis is a promising approach for converting biomass to solids, liquids, and gases. Herein wood vinegars (WVs) were produced from pyrolysis of Eucommia ulmoides Olivers (EUO) branches in different temperature ranges that were labeled as WV90∼120 and WV480∼510. The yield, the physicochemical characteristics, the chemical composition, and the antimicrobial activity of the WVs were comprehensively investigated. During the pyrolysis process, as the temperature increased, the yield of the WVs increased firstly and then decreased, and WV300∼330 achieved the maximum yield of 236.79 g, accounting for 23.26% of the total amount of raw WVs. However, both the lowest pH value (2.39) and the highest total acid content (12.70%) occurred for WV270∼300. GC-MS results demonstrated that six components of WVs for WV300∼330 were phenols, ketones, aldehydes, alcohols, organic acids, and benzenes, while no clear regularity between the contents of each component and the reaction temperature was found. Thereafter, the antimicrobial activity of WVs was tested, and the results showed that the longest inhibition circle diameter of 24.50 mm for Enterobacter aerogenes was obtained for WV300∼330 indicating an excellent antibacterial activity; the overall inhibitory effects of WVs on bacteria were superior to those of plant pathogens and fungus. Therefore, WV300∼330 showed a better antimicrobial activity than that of WV270∼300 in this study. The result could be a reference for the further utilization of WVs in the field of antimicrobial development.
1. Introduction
Biomass, with the characteristics of renewability, environment friendliness, and abundance, can be converted to combustible gas, bio-ethanol, liquid fuels, and chemicals by biochemical and thermo chemical approaches.1 However, one of the main concerns is the conversion technologies that convert biomass into high value-added products.
Eucommia ulmoides Olivers (EUO), also known as Du-zhong, Tuchong, Sizhong and Sixian with a long history in the Chinese medical classic Shen Nong Ben Cao Jing and Ben Cao Gang Mu, is not only a unique economic tree species, but a rare and precious medicinal plant in China.2 Several research studies have indicated that EUO has great application potential in the field of medicinal health and the materials industry. Among them, the aqueous extracts of EUO's leaves have significant anti-biomolecule oxidation effect, and show anti-aging and anticancer potential.3,4 Besides, it was reported that gutta percha extracted from leaves and barks have better performance in terms of the hydrophobicity, thermal stability, tensile strength, and resisting acids or alkalis compared with synthetic rubber, which could be used in packaging, electrical insulation or as filling materials in dentistry.5 However, using leaf model of Eucommia planting (ULMEP) makes it easily to obtain a large number of useful leaves and barks, while a large quantity of branches as biowaste were produced annually, which can affect the living and production environment.2 Relevant literature showed the annual production of branches reached 18.0–22.5 tons per ha in 2015, and the planting area is increasing due to the high value of secondary metabolites in the leaves and barks.6 Moreover, Eucommia ulmoides Olivers (EUO) is included in the National Reserve Forest Tree Species List in China, and the target of its planting area will reach 6.7 × 105 ha in 2025. Therefore, in order to prevent the accumulation of EUO biomass, appropriate measures are necessary to be taken to convert the branches to useful products.
Pyrolysis, as a promising technique for converting biomass to get commercial products such as bio-gas, charcoal, and wood vinegar, is a generation process of three-phase products including solids, liquids, and gases formed by the thermal decomposition of biomass occurring in the absence of oxygen.7 So far, properties or applications of bio-char and pyrolysis gas have been discussed comprehensively:8–10 the bio-char addition to anaerobic digestion and soil could significantly improve methane production, mitigate ammonia inhibition, and improve soil fertility characteristics, while it has a large potential of absorbing and storing carbon without converting it into greenhouse gases (carbon dioxide) that are emitted into the air.11–13 Moreover, the pyrolysis gas has higher heat value than conventional gasification gas, thus could be applied to the downstream gas turbine or other combustion engines for power generation or used as a substitute for civil gas for the purpose of cooking.14
However, regarding the pyrolysis liquid, especially the wood vinegars (WVs), the application research was rare due to the differences in yield and complicated constituent of WVs produced by different raw materials or pyrolysis parameters.15 WVs consists of many complex organic components and compounds, which are obtained from the condensation process of the volatiles of the flue gas, and mainly consist of organic acids, phenols, and ketones.16 In terms of the difficulty of traditional utilization of wood vinegar in incant Judaism, Islam and so on, in order to realize the high value-added utilization of the biomass resources, it is urgent to expand the application range and develop the new products of WVs. Recently, studies on the physicochemical or antimicrobial properties of WVs were quite intensive, mainly focusing on the effects of different pyrolysis parameters such as feedstock, heating rate, residence time, pyrolysis final temperature and so on.15,17–20 For example, Godfrey et al. studied the differences in the chemical composition of the banana waste including leaves, stem and peels generated vinegar, under the pyrolysis conditions as follows: final pyrolysis temperature of 550 °C, a heating rate of 10 °C min−1, and a residence time of 45–90 min.21 In addition, WVs obtained from pyrolysis of Litchi chinensis have antibacterial activity for a group of clinically antibiotic resistant isolates and all the bacterial strains with a range of disc inhibition zone between 15–19 mm.22 It was previously stated that WVs yield from pyrolysis at 450 °C have higher antifungal activity for Reticulitermes speratus and Coptotermes formosanus than those at 400 °C and 350 °C.23 Whereas, the attention paid to WVs from EUO branches remains blank. Various types of biomasses have different characteristics including composition and compounds, so the characteristics of WVs may be associated with the type of biomass. Therefore, it is necessary to investigate the antimicrobial activity of WVs from EUO branches and the effect of different organic ingredients.
Based on the above, the main aim of this study was to investigate the physicochemical properties and antimicrobial activity of the WVs obtained by pyrolysis of EUO branches at different temperature ranges. In addition, the specific substances in WVs which played a decisive role in enhancing the antimicrobial activity was identified.
2. Material and methods
2.1. Materials
EUO wood cultivated via LUMEP was harvested from an experimental field in the Northwest A&F University, Yangling, Shaanxi, China. After barks and leaves were peel off, the EUO branches were dried and cut into chips with an average size around 15 mm (diameter) × 200 mm (length). The contents of cellulose, hemicelluloses, and lignin in raw materials were 32.54 wt%, 21.73 wt%, and 41.66 wt%, respectively. The contents of cellulose, hemicelluloses, and lignin in raw materials were calculated after measurement using a semi-automatic fiber analyzer (ANKOM A200i). Results of the proximate analysis and ultimate analysis of EUO branches were shown in Table 1.
Table 1 Chemical properties of Eucommia ulmoides Olivers branchesa
Proximate analysis |
Content (wt%) |
Ultimate analysis |
Content (wt%) |
M = moisture; FC = fixed carbon; V = volatile; A = ash. The content of oxygen was calculated by difference. |
M |
1.10 |
C |
47.53 |
FC |
14.47 |
H |
6.51 |
V |
82.77 |
Ob |
44.54 |
A |
1.66 |
N |
1.42 |
2.2. Wood vinegars preparation from EUO branches
A vertical pyrolytic reactor with height of 400 mm and internal diameter of 200 mm was used for the pyrolysis. A pipe with pyrolytic smoke was connected to the water-cooling device and applied to collect WVs at different temperature ranges by means of a separating funnel. For each run, reactor was heated from room temperature to the target temperature with a rate of 1 °C min−1 and maintained for 30 min to ensure complete pyrolysis. 600 g of biomass loading for each condition based on dry weight. With the temperature interval of 30 °C from 90 °C to 510 °C, the collected WVs collected were placed in brown bottles and kept away from light. For the sake of obtaining enough WVs, each condition was repeated three times.
After 40 days, the crude WVs was divided into three distinct layers. The upper layer was thin oil, the middle was clear liquid, and the bottom was sticky wood tar with other substances. The clear liquid, as effective WVs, was separated through the siphon method, and the refined WVs were labeled as WV90∼120 and WV480∼510 based on their preparation temperatures. WV90∼120 means the WVs was obtained at the temperature ranges from 90 °C to 120 °C, and 480 to 510 °C for WV480∼510. The yield of WVs were calculated based on the weight of feed.
2.3. Physiochemical properties measurement of wood vinegars
The proximate analysis was performed for the contents of moisture, fixed carbon, volatile matter, and ash based on the ASTM D1762-84 methods. The ultimate analysis was conducted using an elemental analyser (Vario Macro Cube, Elementar, Germany) under CHNS mode. pH was measured by a pH meter. Pycnometer method was adopted to determine the density. The refractive index was measured by a sugar meter (MASTER-20α, Japan Atago) that convert the refractive index into sugar content, expressed in the form of a sugar meter readings. Total organic acids were determined by titrating using the standard solution of 0.1 mol L−1 NaOH and were calculated based on the mass fraction of acetic acid.
2.4. GC-MS analysis of wood vinegars
In order to obtain the organic components contained in the refined WVs, GC-MS analysis was carried out. The gas chromatographic conditions were as follows: DB-WAX column with a size of 30.00 m × 0.25 mm × 0.25 μm was used. The injection port temperature was 220 °C and the injection volume was 1.00 μL. The split injection was adopted at a split rate of 80
:
1. N2 was used as carrier gas with a stable flow rate of 1.00 mL min−1. The column temperature was maintained at 60 °C for 3 min, and the temperature was raised to 240 °C at a heating rate of 5 °C min−1 and kept for 10 min. The mass spectrometry conditions were: electron impact (EI) source, electron energy 70 eV, source temperature 230 °C, scanning range was 35–400 amu s−1. And the mass spectrometry library was NIST library. The corresponding peak areas were used to determine the relative content of compounds in the refined WVs.
The refined WVs were treated with ether extraction after modification.24 3 mL ether was added to 10 mL WVs at different temperature ranges for a total of six times. In order to remove the remaining ether, the combined ether extract was loaded into a reagent bottle, which was heated by a water bath at 40 °C. The concentrated WVs was then analyzed as the GC-MS sample.
2.5. Antimicrobial activity test
The method of agar plate diffusion was adopted to investigate the antimicrobial activity of WVs.25 Under sterile condition, the test spawns were made into 106 mL−1 of spore suspension, then 0.1 mL of spore suspension was taken and uniformly painted to the mediums. Filter papers with 6 mm diameter, after sterilization, were soaked in WVs about 10 s, then stay on the wall of the container until there was no liquid dripping. Finally, the filter papers were placed on corresponding medium, and three filter papers was placed on the medium of each Petri-dish with equidistant. Each treatment was repeated three times and the sterile water was used as a control. Bacteria and fungus were incubated at 28 °C for 24 h and 30 °C for 48 h, respectively. After the cultivation, the diameter of the inhibition circle on the Petri-dish was measured by the cross intersect method with a Vernier caliper. The antimicrobial activity was represented by the diameter of inhibition circle. The greater of the diameter, the more obvious of the antimicrobial activity of WVs.26
2.6. Statistical analysis of the data
Data was organized using of Excel software. Variance analysis and significance test of each index were performed using SPSS software (version 17.0).
3. Results and discussion
3.1. The process performance of wood vinegars yield
The WVs were collected with an interval of 30 °C from 90 °C to 510 °C, and 14 samples were obtained. Fig. 1 shows the yields of WVs along with the increase of temperature. In general, with the increase of pyrolysis temperature, the yield of WVs showed a trend of decrease and then increase followed by a continuous decrease. Sample for WV300∼330 reached the maximum yield of 236.79 g, accounting for 23.26% of the total amount of raw WVs, followed by 179.25 g at 270–300 °C. What we should pay special attention to was that the yield from sample WV90∼120 to WV150∼180 slightly decreased. The same phenomenon also appeared in the article,16 while the only difference was that the temperature of the decline in output was varied from 130 °C to 170 °C for WVs from walnut tree branches. The results may be due to the variance of moisture contents in different raw materials, which could be proved by the DSC curves of pyrolysis of three components. The reactions occurred at 100 °C were all endothermic, which was mainly attributed to the dehumidification when the biomass was heated up.27 In addition, the minimum yield of WVs was 6.18 g, which was obtained from WV480∼510 and account for 26.10% of the maximum yield of WVs. As we know, the pyrolysis of hemicellulose and cellulose occurred quickly, with the weight loss of hemicellulose mainly happened at 220–315 °C and that of cellulose at 315–400 °C. However, lignin was more difficult to decompose, as its weight loss happened in a wide temperature range (from 160 °C to 900 °C) and the current pyrolytic temperature was not sufficient to further promote the cleavage of the bonds to produce more liquid products.27
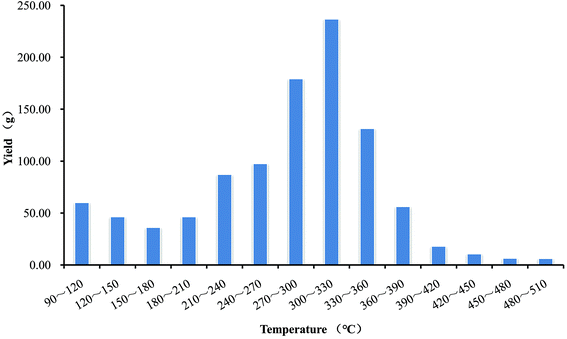 |
| Fig. 1 Yield of wood vinegars at different pyrolysis temperature ranges. | |
All the WVs produced at different temperature ranges were refined by the method mentioned in Section 2.2. The physical properties of WVs collected at different temperature ranges can be seen in Table 2. Both the density and color of the WVs obtained at different pyrolysis temperature ranges showed no significant difference, except for the color of the first five samples which were slightly lighter than others. The reason may be that, at low pyrolysis temperature, the decomposition of cellulose and hemicellulose generated light-colored and transparent water-phase substance.28 In addition, the difference in moisture content of the feedstock may also contribute to the difference in color of WVs.28,29 More importantly, it was found that there was a negative correlation between pH value and total organic acid content, thus both the minimum pH value (2.39) and the maximum total organic acid content (12.70%) was achieved from WV270∼300.
Table 2 The Physical properties of refined wood vinegar of Eucommia ulmoides Olivers branches collected at different temperature ranges
Wood vinegar |
pH |
Density (g cm−3) |
Refractive index (%) |
Total organic acid (%) |
Color |
WV90∼120 |
2.81 |
1.0193 |
9.50 |
1.80 |
Red brown |
WV120∼150 |
2.80 |
1.0098 |
5.80 |
1.35 |
Red wine |
WV150∼180 |
2.81 |
1.0043 |
5.00 |
0.85 |
Light brown |
WV180∼210 |
2.60 |
1.0136 |
10.00 |
3.65 |
Red wine |
WV210∼240 |
2.47 |
1.0365 |
21.00 |
7.85 |
Red brown |
WV240∼270 |
2.41 |
1.0555 |
29.20 |
12.30 |
Black brown |
WV270∼300 |
2.39 |
1.0752 |
37.00 |
12.70 |
Black brown |
WV300∼330 |
2.47 |
1.0744 |
45.00 |
6.95 |
Black brown |
WV330∼360 |
2.64 |
1.0826 |
44.00 |
4.90 |
Black brown |
WV360∼390 |
3.08 |
1.0642 |
44.00 |
5.45 |
Black brown |
WV390∼420 |
3.00 |
1.0652 |
34.00 |
4.25 |
Black brown |
WV420∼450 |
3.03 |
1.0477 |
24.00 |
3.35 |
Black brown |
WV450∼480 |
3.26 |
1.0254 |
20.00 |
3.10 |
Black brown |
WV480∼510 |
3.32 |
1.0185 |
18.50 |
3.15 |
Black brown |
3.2. GC-MS analysis of wood vinegars
The GC-MS analysis was employed to further explain the compositional distinction amongst the WVs produced under different conditions. The relative contents of main components for the WVs are shown in Fig. 2. Six main organic ingredients of the WVs were found, including phenols, ketones, aldehydes, alcohols, organic acids, and benzenes. However, except for benzenes and aldehydes, there was no clear relationship between the relative content and the changes in preparation temperatures. Phenols were the dominant component of WVs, followed by ketones, aldehydes, alcohols, organic acids, and benzenes. Moreover, for WV480∼510, the relative content of phenols varied from 30.90% to 48.80%, accounting for almost half the weight, and reached the highest relative content. The relative content of ketones varied from 15.72% to 25.93%. Furthermore, the maximum relative contents of organic acids (8.30%) and alcohols (8.22%) were obtained for WV240∼270 and WV360∼390, respectively. The maximum relative contents of aldehydes (16.27%) and benzenes (17.43%) were achieved for WV180∼210 and WV390∼420, respectively. In terms of benzenes, when temperature increased to more than 270 °C, the relative content of benzenes (6.84–17.43%) increased quickly and exceeded that of aldehydes (0.09–9.37%), alcohols (3.59–8.22%), and organic acids (1.62–8.12%). However, when the temperature reached 330 °C and even after, the relative content of aldehydes sharply decreased from 5.01% to 0.09%. The reason may be that the water-phase products including alcohols, organic acids, and aldehydes resulted from the decomposition of hemicelluloses and cellulose were converted into benzene and other oil-phase products with the increase of temperature.27
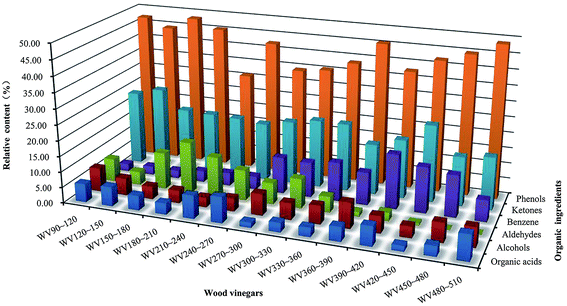 |
| Fig. 2 Relative contents of six major compositions of wood vinegars produced at different temperature ranges. | |
3.3. Antibacterial activity of wood vinegars
Antibacterial activity test of WVs produced at different temperature ranges on the inhibition of five kinds of bacteria including Enterobacter aerogenes, Escherichia coli, Staphylococcus aureus, Bacillus subtilis, and Bacillus cereus. The inhibition circle diameters of different bacteria are showed in Fig. 3 and S1.† All the WVs samples have showed antibacterial activity for the five bacteria. On one hand, WV300∼330 had the greatest antibacterial activity for three of the five kinds of bacteria in the order of Enterobacter aerogenes (24.50 mm) > Escherichia coli (21.00 mm) > Bacillus subtilis (19.67 mm). For Staphylococcus aureus and Bacillus cereus, the greatest antibacterial activity appeared for WV240∼270, and the maximum inhibition circle diameters were 32.00 mm and 23.50 mm, respectively. Moreover, the antibacterial activity of WV240∼270 for Staphylococcus aureus was more pronounced than that of the other four bacteria, and the inhibition circle diameters (32.00 mm) was much bigger than the diameter of tetracycline (17.09 mm). On the other hand, all the pictures showed an overall trend of first increase followed by decrease.
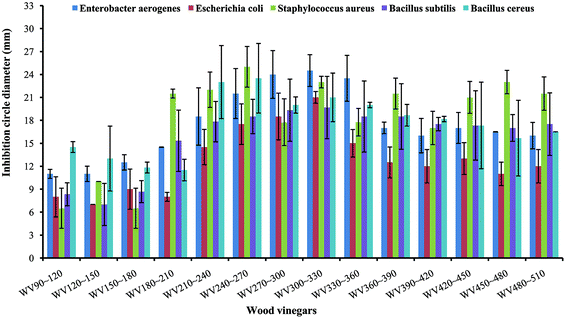 |
| Fig. 3 Antibacterial activity of refined wood vinegar of Eucommia ulmoides Olivers branches collected at different temperature ranges. | |
The WVs can be categorized into three types based on their preparation temperatures hence: low temperature (90–210 °C) WVs, medium temperature (210–360 °C), and high temperature (360–510 °C). The antibacterial activity for the other three bacteria was ranked as: medium temperature WVs > high temperature WVs > low temperature WVs, while for Staphylococcus aureus and Bacillus subtilis, the antibacterial activity under medium temperature WVs and high temperature WVs were obviously better than that of low temperature except for the WV180∼210. For Bacillus subtilis, the differences of the antibacterial activity between medium temperature WVs and high temperature WVs were not significant. However, for Staphylococcus aureus, an alternating trend appeared under medium temperature and high temperature. The inhibition circle diameters of WV240∼270 was up to 32.00 mm, which was not only significantly higher than the remaining WVs samples, but also preceded that of the antibacterial activity of tetracycline (7.5 mg mL−1) as mentioned above.
All in all, WV300∼330 had the greatest antimicrobial activity for Enterobacter aerogenes, Escherichia coli, and Bacillus subtilis, while WV240∼270 had the greatest antimicrobial activity on Staphylococcus aureus and Bacillus cereus. According to the result of GC-MS analysis, compared with the other thirteen WVs samples, the relative contents of the six mainly organic components in WV300∼330 were not the highest, but the antibacterial activity was the best for the three bacteria. In addition, WV240∼270 with the highest organic acids (8.30%) played a leading role in antimicrobial activity for Staphylococcus aureus and Bacillus cereus. Therefore, the antimicrobial activity of WVs was not only determined by the components, but also closely related to the characteristics of the tested bacteria and the interaction of multi-substances with different content in WVs. In a word, the optimum pyrolysis temperature range of WVs should be selected based on the characteristics of bacteria.
3.4. Antifungal activity of wood vinegars
As shown in Fig. 4 and S2,† unlike bacteria, not all of the WVs samples showed antifungal activity for Penicillium, Aspergillus, and Rhizopus, and the overall antifungal activity was not significant. First of all, as showed in Fig. 4(a), the antifungal activity of WVs could be divided into three levels based on the strength such as no effect (NE), unobvious effect (UE), and obvious effect (OE). NE consists of the first three WVs samples; UE were constitutive of WV270∼300 to WV330∼360, and the remaining WVs samples with inhibition circle diameters of more than 9.00 mm belonged to OE. WV450∼480 obtained the longest inhibition circle diameter (12.50 mm), while the difference of which was not significant compared with other WVs belonged to OE.
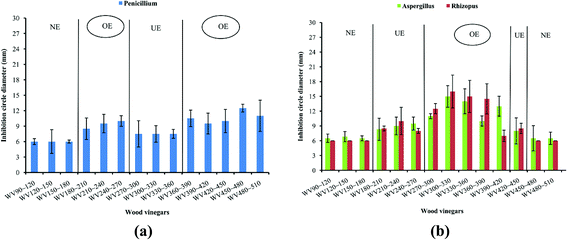 |
| Fig. 4 Antifungal activity of refined wood vinegar of Eucommia ulmoides Olivers branches collected at different temperature ranges. ((a) Penicillium, (b) Aspergillus and Rhizopus). NE: no effect, UE: unobvious effect, OE: obvious effect. | |
As shown in Fig. 4(b), except for the first three and the last two WVs with inhibition circle diameters less than 7.00 mm, the inhibition circle diameters of the remaining WVs for Aspergillus and Rhizopus showed a general trend of first increase followed by decrease along with the increase of temperature. The antifungal activity of WV300∼330 was stronger than others. That may indicate that WVs with complex compositions at different temperature ranges selectively changed the permeability of cell membrane, inhibited the speed of fungus division or the synthesis of protein, which result in the exudation of protein, carbohydrate, Na+, and K+, leading to metabolism disorder, thus showing various inhibitory effects.30
Above all, the antifungal activity of WVs was not promising as that for bacteria.
3.5. Anti-plant pathogens activity of wood vinegars
Plant pathogens including buckwheat leaf spot, wheat root rot, eggplant wilt, watermelon wilt, and root rot were selected to study the anti-plant pathogens activity of WVs at different temperature ranges. The anti-plant pathogens activity of wood vinegars collect at different temperature ranges were showed in Fig. 5 and S3.† First, as shown in Fig. 5(a), the difference of the inhibition circle diameters of all the WVs were not significant for root rot, indicating that the anti-plant pathogens activities of WVs for root rot were weak. The longest inhibition circle diameter appears for WV270∼300 and was only reaches 11.00 mm.
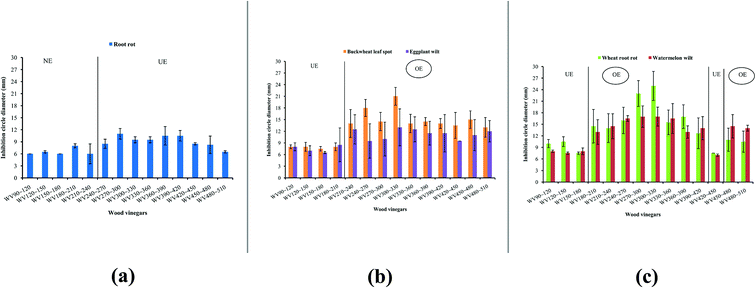 |
| Fig. 5 Anti-plant pathogens activity of refined wood vinegar of Eucommia ulmoides Olivers branches collected at different temperature ranges. ((a) root rot, (b) buckwheat leaf spot and eggplant wilt, (c) wheat root rot and watermelon wilt). NE: no effect, UE: unobvious effect, OE: obvious effect. | |
For buckwheat leaf spot and eggplant wilt, it showed similar trend of the inhibition circle diameter was found in Fig. 5(b). The inhibition circle diameter of buckwheat leaf spot was larger than or equal to eggplant wilt for all WVs. For the sake of illustration, the first four WVs those inhibition circle diameters were less than 8.50 mm and poor in anti-plant pathogens activity belonged to UE. While the remaining ten WVs those inhibition circle diameters of buckwheat leaf spot and eggplant wilt were varied from 13.00 mm to 21.00 mm, and 9.50 mm to 13.00 mm, respectively. WV300∼330 reached the maximum. As demonstrated in Fig. 5(c), except for the first three WVs and WV420∼450 that belonged to UE, the inhibition circle diameter of the majority of remaining samples for wheat root rot and watermelon wilt exceeded 10.50 mm, which exhibited excellent anti-plant pathogens activity. The maximum value for wheat root rot and watermelon wilt appeared for WV300∼330 and were 25.00 mm and 17.00 mm, respectively. The anti-plant pathogens activity of WV270∼300 and WV300∼330 for wheat root rot was significantly better than that of watermelon wilt.
Above all, for the five plant pathogens, the anti-plant pathogens activity of WVs for wheat root rot was the strongest of followed by buckwheat leaf spot, watermelon wilt, eggplant wilt, and root rot. Therefore, WV300∼330 had great potential to be a bio-pesticide for wheat root rot.
3.6. Summary about antimicrobial activities and chemical compositions of different wood vinegars
A graphical summary about the chemical compositions of different WVs and their inhibition effects on various microorganisms were exhibited in Fig. 6. WVs that showed promising antimicrobial activity for different microorganisms were WV240∼270, WV270∼300, WV300∼330, and WV450∼480, suggesting that the optimal pyrolysis temperature was mainly in the medium temperature range.
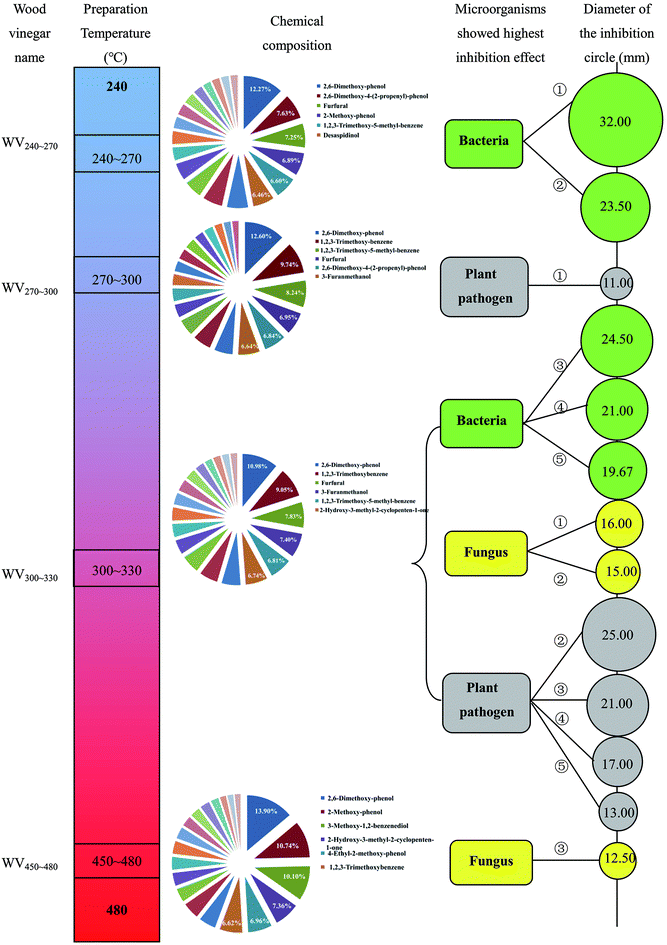 |
| Fig. 6 Graphical summary: chemical compositions of different wood vinegars and their inhibition effects on various microorganisms. (The name of the microorganisms shown in the figure were: (1) bacteria: ① Staphylococcus aureus, ② Bacillus cereus, ③ Enterobacter aerogenes, ④ Escherichia coli, ⑤ Bacillus subtilis; (2) fungus: ① Rhizopus, ② Aspergillus, ③ Penicillium; (3) plant pathogens: ① root rot, ② wheat root rot, ③ buckwheat leaf spot, ④ watermelon wilt, ⑤ eggplant wilt.) | |
It was clear that WV300∼330 showed excellent antimicrobial activity for the three types of microbes (③ Enterobacter aerogenes, ④ Escherichia coli, and ⑤ Bacillus subtilis), two fungus (① Rhizopus and ② Aspergillus), and four plant pathogens (② wheat root rot, ③ buckwheat leaf spot, ④ watermelon wilt and ⑤ eggplant wilt), which covers about 70% of the tested strains. In comparison, WV240∼270 only showed good antibacterial activity for two types of bacteria namely Staphylococcus aureus and Bacillus cereus. In addition, both WV270∼300 and WV450∼480 showed good antimicrobial activity for root rot and Penicillium. Moreover, the antibacterial activity of WVs was generally superior to that of plant pathogens followed by fungus. Therefore, for practical applications, the preparation temperature of WVs should be selected based on the specific types of microorganisms.
In brief, difference in chemical composition of WVs caused by different preparation temperatures resulted in a diversity of antimicrobial activity. Some previous investigations demonstrated that the antimicrobial activity of WVs was directly related to the content of organic acids.16,23 It has been reported that the pH value of WVs (2.20–3.01) was much lower than the optimal value of the bacteria growth (7.0), thereby inhibited or prevented bacterial growth.20 While others insisted that the active components responsible for antimicrobial activity were phenols.22,31,32 Thereinto, some phenolic compounds such as phenol and cresols have been well known as an antimicrobial agent.33 All in all, the antimicrobial activity of WVs seems not to be determined by a specific substance, but multi-substances interaction. In this study, WV300∼330 showed the best antimicrobial activity for most of the tested microorganisms, and the relative contents of phenols, ketones, organic acids, aldehydes, alcohols, and benzenes presented in this sample were 35.50%, 21.72%, 2.95%,9.37%, 5.34%, and 11.23%, respectively.
4. Conclusions
With the increase in pyrolysis temperature, the yield of WVs increased first followed by decrease. WV300∼330 reached the maximum yield of 236.79 g. GC-MS analysis of WVs showed that there was no clear correlation between the relative content of specific organic component and temperature. WVs collected at different temperature ranges had antimicrobial activity for bacteria, fungus, and plant pathogens, but the antimicrobial activity varies obviously. The longest inhibition circle diameters of 24.50 mm of Enterobacter aerogenes was obtained for WV300∼330, showing the greatest antibacterial activity. As a result, WV300∼330 showed a great potential for being an antimicrobial agent.
Conflicts of interest
There are no conflicts to declare.
Acknowledgements
The research was supported by the National Natural Science Foundation of China (No. 51576167).
References
- A. Demirbas, Energy Convers. Manage., 2001, 42, 1357–1378 CrossRef CAS.
- M.-Q. Zhu, J.-L. Wen, Y.-H. Zhu, Y.-Q. Su and R.-C. Sun, Ind. Crops Prod., 2016, 83, 124–132 CrossRef CAS.
- G. C. Yen and C. L. Hsieh, J. Agric. Food Chem., 1998, 46, 3952–3957 CrossRef CAS.
- X. P. Dai, Q. Huang, B. T. Zhou, Z. C. Gong, Z. Q. Liu and S. Y. Shi, Food Chem., 2013, 139, 563–570 CrossRef CAS PubMed.
- J. Xu, B. Liu, J. Hu and H. Hou, Ind. Crops Prod., 2017, 108, 140–148 CrossRef CAS.
- M. Q. Zhu, J. L. Wen, Z. W. Wang, Y. Q. Su, Q. Wei and R. C. Sun, Appl. Energy, 2015, 158, 233–242 CrossRef CAS.
- A. Demirbas and G. Arin, Energy Sources, 2002, 24, 471–482 CrossRef CAS.
- J. Wang, Z. Xiong and Y. Kuzyakov, GCB Bioenergy, 2016, 8, 512–523 CrossRef CAS.
- X. F. Tan, Y. G. Liu, Y. L. Gu, Y. Xu, G. M. Zeng, X. J. Hu, S. B. Liu, X. Wang, S. M. Liu and J. Li, Bioresour. Technol., 2016, 212, 318–333 CrossRef CAS PubMed.
- B. F. Zhao, X. D. Zhang, L. Chen, L. Z. Sun, H. Y. Si and G. Y. Chen, Bioresour. Technol., 2014, 156, 78–83 CrossRef CAS PubMed.
- J. M. Novak, W. J. Busscher, D. L. Laird, M. Ahmedna, D. W. Watts and M. A. S. Niandou, Soil Sci., 2009, 174, 105–112 CrossRef CAS.
- D. Woolf, J. E. Amonette, F. A. Street-Perrott, J. Lehmann and S. Joseph, Nat. Commun., 2010, 1, 56 Search PubMed.
- J. Mumme, F. Srocke, K. Heeg and M. Werner, Bioresour. Technol., 2014, 164, 189–197 CrossRef CAS PubMed.
- G. Chen, J. Andries, Z. Luo and H. Spliethoff, Energy Convers. Manage., 2003, 44, 1875–1884 CrossRef CAS.
- A. Sharma, V. Pareek and D. Zhang, Renewable Sustainable Energy Rev., 2015, 50, 1081–1096 CrossRef CAS.
- Q. Wei, X. Ma and J. Dong, J. Anal. Appl. Pyrolysis, 2010, 87, 24–28 CrossRef CAS.
- Q. Wu, S. Zhang, B. Hou, H. Zheng, W. Deng, D. Liu and W. Tang, Bioresour. Technol., 2015, 179, 98–103 CrossRef CAS PubMed.
- M. Garcia-Perez, X. S. Wang, J. Shen, M. J. Rhodes, F. J. Tian, W. J. Lee, H. W. Wu and C. Z. Li, Ind. Eng. Chem. Res., 2008, 47, 1846–1854 CrossRef CAS.
- S. Mathew, Z. A. Zakaria and N. F. Musa, Process Biochem., 2015, 50, 1985–1992 CrossRef CAS.
- T. Jin, Y. X. Wu and Q. Wang, Adv Intel Soft Compu, 2012, 134, pp. 451–457 Search PubMed.
- G. Omulo, S. Willett, J. Seay, N. Banadda, I. Kabenge, A. Zziwa and N. Kiggundu, J. Sustain. Dev., 2017, 10, 81 CrossRef.
- J. F. Yang, C. H. Yang, M. T. Liang, Z. J. Gao, Y. W. Wu and L. Y. Chuang, Molecules, 2016, 21, 1150 CrossRef PubMed.
- H. A. Oramahi and T. Yoshimura, J. Wood Sci., 2013, 59, 344–350 CrossRef CAS.
- Y. M. Xu, L. L. Gao, Y. Z. Piao, J. T. Liu, Z. X. Song and T. Z. Sun, Advances In Energy Science And Equipment Engineering, 2015, pp. 2795–2799 Search PubMed.
- P. Luber, E. Bartelt, E. Genschow, J. Wagner and H. Hahn, J. Clin. Microbiol., 2003, 41, 1062–1068 CrossRef CAS PubMed.
- X. Ma, Q. Wei, S. Zhang, L. Shi and Z. Zhao, J. Anal. Appl. Pyrolysis, 2011, 91, 338–343 CrossRef CAS.
- H. Yang, R. Yan, H. Chen, D. H. Lee and C. Zheng, Fuel, 2007, 86, 1781–1788 CrossRef CAS.
- S. I. Yang, M. S. Wu and C. Y. Wu, Energy, 2014, 66, 162–171 CrossRef CAS.
- E. K. Kai Sipila, L. Fagernas and A. Oasmaa, Biomass Bioenergy, 1998, 14, 103–113 CrossRef.
- S. E. Walsh, J. Y. Maillard, A. D. Russell, C. E. Catrenich, D. L. Charbonneau and R. G. Bartolo, J. Appl. Microbiol., 2003, 94, 240–247 CrossRef CAS PubMed.
- A. Y. Loo, K. Jain and I. Darah, Food Chem., 2007, 104, 300–307 CrossRef CAS.
- Y. Baimark and N. Niamsa, Biomass Bioenergy, 2009, 33, 994–998 CrossRef CAS.
- Y. H. Hwang, Y. I. Matsushita, K. Sugamoto and T. Matsui, J. Microbiol. Biotechnol., 2005, 15, 1106–1109 CAS.
Footnote |
† Electronic supplementary information (ESI) available. See DOI: 10.1039/c8ra07491g |
|
This journal is © The Royal Society of Chemistry 2018 |