DOI:
10.1039/C8RA07256F
(Paper)
RSC Adv., 2018,
8, 36392-36399
Synthesis of benzo[4,5]imidazo[1,2-a]pyrimidines and 2,3-dihydroquinazolin-4(1H)-ones under metal-free and solvent-free conditions for minimizing waste generation†
Received
31st August 2018
, Accepted 12th October 2018
First published on 26th October 2018
Abstract
Brønsted acidic ionic liquid was found to be an efficient and recyclable catalyst for the synthesis of benzo[4,5]imidazo[1,2-a]pyrimidines and 2,3-dihydroquinazolin-4(1H)-ones. The reactions proceeded smoothly with a broad scope of substrates providing the expected products in good to excellent yields under an atom-economical pathway. The low-cost recyclable catalyst, metal- and solvent-free conditions, and the ease of product isolation are the highlighted advantages in solving the issue of trace metal contamination in synthesized pharmaceuticals.
Introduction
The invention of new catalysts for the synthesis of biologically active compounds is essential to the advancement of green chemistry.1–3 Classical aqueous inorganic acids including H2SO4, HF, HCl, HNO3, and H3PO4 have been used for various organic transformations. Nevertheless, these homogeneous Brønsted acids are not suitable for widespread application due to separation and environmental issues.4 Ionic liquids have attracted considerable attention in many fields due to their structural diversity, low cost, and low toxicity.5–7 The use of ionic liquids as green catalysts for chemical reactions has presented many advantages in a sustainable synthesis.8–13 These homogeneous catalysts are thermally stable, highly active, and easily recyclable.14 The most popular Brønsted acidic ionic liquids have been easily prepared by the reaction of 1,4-butane sultone or 1,3-propane sultone with N-methylimidazole.15 Thus, these Brønsted acidic ionic liquids have been widely used as green catalysts for various organic transformations.16–24 Herein, we report the synthesis of benzo[4,5]imidazo[1,2-a]pyrimidines and 2,3-dihydroquinazolin-4(1H)-ones catalyzed by a Brønsted acidic ionic liquid under solvent-free conditions. The presented method is considered an essential solution for the environmental problems in industrial processes. The current synthesis scheme contributes to the sustainable and low-cost pathway for the preparation of benzo[4,5]imidazo[1,2-a]pyrimidines and 2,3-dihydroquinazolin-4(1H)-ones.
Results and discussion
Synthesis of benzo[4,5]imidazo[1,2-a]pyrimidines
Pyrimidine derivatives are backbones of various pharmaceutical drugs with various applications including anticancer,25–29 anti-inflammatory,30–32 antibacterial,31,33–35 and antifungal activities.35–37 Over the past decade, many approaches have been achieved for the preparation of pyrimidine derivatives.38–42 However, multi-step or low-yield procedures and harmful conditions could limit the widespread use of these approaches. Recently, multicomponent reactions have provided new prospects for the advancement in organic synthesis to eliminate these drawbacks.43,44 Herein, the Brønsted acidic ionic liquid was used for the first time to catalyze the synthesis of benzo[4,5]imidazo[1,2-a]pyrimidines via a one-pot multicomponent reaction. Initially, [(4-SO3H)BMIM]HSO4 ionic liquid was prepared as reported in the literature.22 The structure of [(4-SO3H)BMIM]HSO4 was confirmed by NMR, FT-IR, TGA, and HR-MS (ESI, Section S2†). The as-synthesized [(4-SO3H)BMIM]HSO4 was obtained in high yield after a simple purification step. Thermogravimetric analysis shows that [(4-SO3H)BMIM]HSO4 is thermally stable (up to 250 °C). Next, we investigated the model reaction between 2-aminobenzimidazole, ethyl acetoacetate, and benzaldehyde in the presence of different catalysts (10 mol%) at 100 °C for 120 min (Table 1). When the model reaction was performed using metal chlorides such as AlCl3, FeCl3, FeCl2, and ZnCl2 as the catalysts at 100 °C for 120 min, the expected product was obtained in 32% to 60% yield (Table 1, entries 1–4). These results encouraged us to test a series of Lewis acidic deep eutectic solvents with zinc chloride. However, the yields of the desired product drastically decreased (Table 1, entries 5–7). Other deep eutectic solvents were investigated by replacing ZnCl2 with ethylene glycol, oxalic acid or glucose (Table 1, entries 8–10), but the yields of the product were not satisfactory. Thus, we focused on ionic liquids. Three types of ionic liquids, namely, [EMIM]Cl, [BMIM]BF4, and [(4-SO3H)BMIM]HSO4, were tested for the model reaction. When the reactions were performed in ionic liquid media, the yields of the desired product drastically increased (Table 1, entries 11–13). Among these ionic liquids, [(4-SO3H)BMIM]HSO4 showed the best efficiency, allowing the reaction to afford 89% yield (Table 1, entry 13). As shown in Table 1, metal chlorides could be employed in the model reaction. However, these metal chlorides are moisture sensitive and easily decompose in the presence of a small amount of water. Furthermore, these metal chlorides could not be recovered and reused after the aqueous workup. The prominent feature of [(4-SO3H)BMIM]HSO4 is stability in water and in almost all cases, catalytic use, recovery, and reuse of [(4-SO3H)BMIM]HSO4 are possible. In our study, we tested different ratios of [(4-SO3H)BMIM]HSO4 with the aim of obtaining the desired product in high yield. The best yield was obtained in the presence of 10 mol% [(4-SO3H)BMIM]HSO4. When the amount of catalyst was increased to 15 mol%, the yield slightly improved. All attempts to reduce the amount of [(4-SO3H)BMIM]HSO4 loading led to diminished yields.
Table 1 Yields for reaction of 2-aminobenzimidazole, ethyl acetoacetate, and benzaldehyde with different catalystsa
Entry |
Catalyst |
Yieldb (%) |
Reaction condition: 2-aminobenzimidazole (1.0 mmol), ethyl acetoacetate (1.0 mmol), benzaldehyde (1.0 mmol), and catalyst (10 mol%) at 100 °C for 120 min. Isolated yield. |
1 |
AlCl3 |
57 |
2 |
FeCl3 |
60 |
3 |
FeCl2 |
32 |
4 |
ZnCl2 |
60 |
5 |
[CholineCl][ZnCl] |
0 |
6 |
[CholineCl][ZnCl]2 |
22 |
7 |
[CholineCl][ZnCl]3 |
12 |
8 |
[CholineCl][ethylene glycol]2 |
59 |
9 |
[CholineCl][glucose] |
48 |
10 |
[CholineCl][oxalic acid]2 |
52 |
11 |
[EMIM]Cl |
67 |
12 |
[BMIM]BF4 |
69 |
13 |
[(4-SO3H)BMIM]HSO4 |
89 |
With the optimized conditions in hand, we focused on using a variety of aromatic aldehydes. The results are presented in Scheme 1. A series of p-substituted benzaldehyde smoothly reacted with 2-aminobenzimidazole and ethyl acetoacetate to provide benzo[4,5]imidazo[1,2-a]pyrimidines in excellent yields. Electron-donating group on benzaldehyde, such as methoxybenzaldehyde, gave the expected products in excellent yield (90%), but longer reaction time was required (150 min). Electron-withdrawing groups on benzaldehyde, such as 2-nitrobenzaldehyde and 4-nitrobenzaldehyde, were all effectively reactive and provided the corresponding products in excellent yields within shorter reaction times (75 min). Benzaldehyde bearing halogen substituents could also react smoothly to attain the expected products in high yields (88–92%), and the substituent position did not significantly affect the reaction yield. In the interest of extending the scope of reaction, we examined acetonylacetone under the current method. To our delight, the reactions proceed smoothly to provide the desired products in high yields (88–93%) and shorter reaction times (60–90 min). Moreover, the method avoids the use of volatile organic solvents, harmful conditions, and additives making it greener than the previous reports. In addition, the pure products were obtained easily by recrystallization; thus, this method shows promise for application in industry.
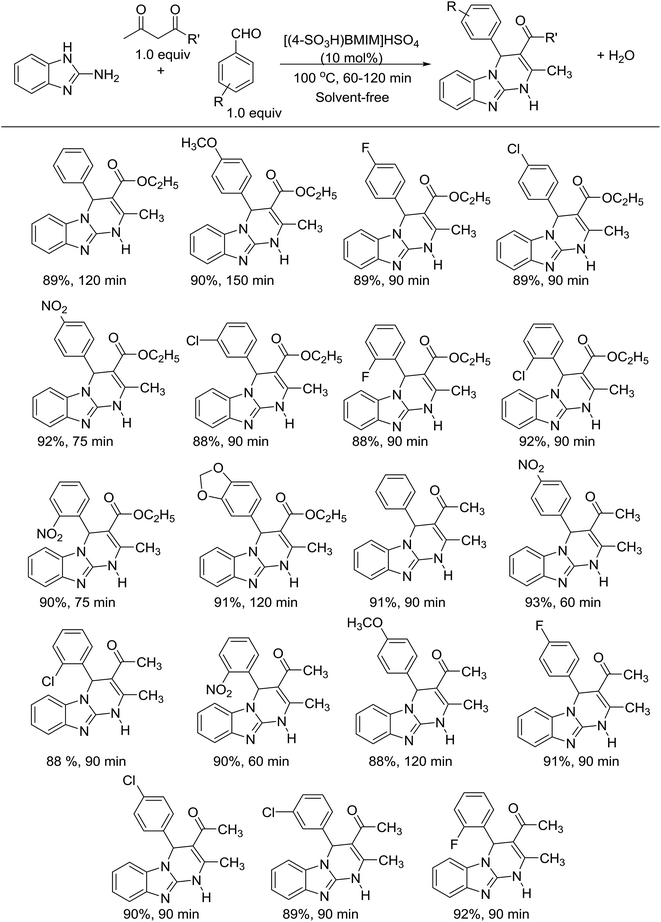 |
| Scheme 1 [(4-SO3H)BMIM]HSO4-catalyzed synthesis of benzo[4,5]imidazo[1,2-a]pyrimidines. | |
The comparison of the present method with previously reported literature is shown in Table 2. The multicomponent reaction of 2-aminobenzimidazole, ethyl acetoacetate, and benzaldehyde in the presence of [(4-SO3H)BMIM]HSO4 afforded the expected product in good yield within 2 h under metal-free and solvent-free conditions (Table 2, entry 6). The previous studies reported that the same reaction also achieved the target products in moderate to good yields, but some methods required longer reaction time and/or high temperature (Table 2, entries, 1–5). More importantly, the [(4-SO3H)BMIM]HSO4 could be recovered and reused without any considerable loss in catalytic activity in a test of six cycles of reuse.
Table 2 Comparison of the present method with previous literature for the one-pot multicomponent synthesis of benzo[4,5]imidazo[1,2-a]pyrimidine
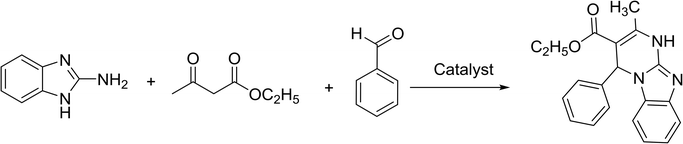
|
Entry |
Catalytic system |
Reaction conditions |
Yield (%) |
1 |
1,1,3,3-N,N,N′,N′-Tetramethylguanidinium trifluoroacetate (30 mol%) |
100 °C, 5 h |
73 (ref. 26) |
2 |
[bmim][BF4] (3 mL) |
90 °C, 7 h |
86 (ref. 36) |
3 |
L-Proline (20 mol%), H2O |
Reflux, 3 h |
91 (ref. 30) |
4 |
Thiamine hydrochloride (5 mol%), H2O |
Reflux, 3 h |
90 (ref. 41) |
5 |
α-Zirconium sulfophenylphosphonate (12 mol%) |
80 °C, 20 h |
73 (ref. 42) |
6 |
Current work: [(4-SO3H)BMIM]HSO4 (10 mol%), solvent-free |
100 °C, 2 h |
92 |
To get more information about the plausible mechanism, we performed three control experiments under the optimized conditions. 2-Benzylidene-3-oxobutanoate intermediate was detected as the major product (by GC-MS) when the model reaction was performed for 20 min. Thus, the reaction mainly followed Knoevenagel condensation between benzaldehyde and ethyl acetoacetate in the first step, where the [(4-SO3H)BMIM]HSO4 acted as a Brønsted acidic catalyst. The control experiments with only two components, i.e., the reaction of benzaldehyde with ethyl acetoacetate, also provided the desired product (2-benzylidene-3-oxobutanoate) in 70% yield, which was higher than the reaction yield of 2-aminobenzimidazole and benzaldehyde. The intermediate could completely convert into the desired product when 2-aminobenzimidazole was added. Based on the above control experiments and previous literature, the plausible mechanism is proposed, as presented in Scheme 2. The reaction first followed Knoevenagel condensation between benzaldehyde and ethyl acetoacetate to produce 2-benzylidene-3-oxobutanoate as intermediate, which could further react with 2-aminobenzimidazole through Michael reaction. Finally, the desired product was obtained through cyclization and dehydration. The [(4-SO3H)BMIM]HSO4 played a vital role in four steps of the reaction.
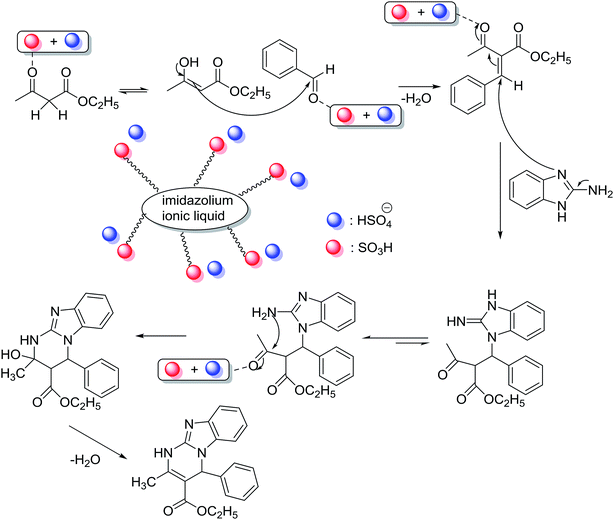 |
| Scheme 2 Proposed mechanism for the preparation of benzo[4,5]imidazo[1,2-a]pyrimidines. | |
Synthesis of 2,3-dihydroquinazolin-4(1H)-ones
Quinazolinones are valuable bioactive compounds possessing antitumor,45–47 antiviral,48,49 antibacterial,50,51 and anti-inflammatory activities.52,53 Traditionally, both homogeneous and heterogeneous acidic catalysts can catalyze the synthesis of 2,3-dihydroquinazolin-4(1H)-ones.54–56 Recently, various protocols have been reported for the preparation of 2,3-dihydroquinazolin-4(1H)-ones with the aid of improving the reaction efficiency.57–61 Moreover, 2,3-dihydroquinazolin-4(1H)-ones have been successfully synthesized using ionic liquids including [BMIM]BF4,62 poly(4-vinylpyridine) supported acidic ionic liquids,63 and imidazolium triflate.64 However, methods that involved these ionic liquids presented some drawbacks, including high temperature, prolonged reaction time, and the use of volatile organic solvents. In this study, we report that [(4-SO3H)BMIM]HSO4 acidic ionic liquid could be used as an active catalyst for the preparation of 2,3-dihydroquinazolin-4(1H)-ones presumably due to strong effect of Brønsted acidic groups on the ionic liquid. Interestingly, various aromatic aldehydes reacted successfully under the optimized conditions, and the expected product was obtained in excellent yields in the presence of [(4-SO3H)BMIM]HSO4 (Scheme 3). The substituent on the benzene did not affect the reaction rate. In addition, pure products were obtained easily by recrystallization. Remarkably, the condensation between anthranilamide and benzaldehyde was successfully performed on a 50 mmol scale, and the expected yield was obtained equally with 1 mmol scale. This method avoids the use of volatile organic solvents, harmful conditions, and metal catalysts, thus showing promise for application in industrial processes.
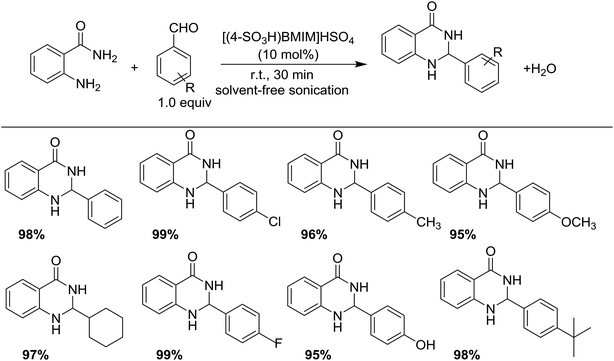 |
| Scheme 3 Reaction scope with aldehydes. | |
The comparison of the presented method with previously reported literature is shown in Table 3. The reaction of anthranilamide and benzaldehyde in the presence of [(4-SO3H)BMIM]HSO4 afforded the expected product in excellent yield at room temperature for 30 min under metal-free and solvent-free conditions (Table 3, entry 6). The previous studies reported that the same reaction also achieved the target products in good to excellent yields, but some methods required the use of a solvent, high temperature and/or longer reaction time (Table 3, entries, 1–5). Additionally, the [(4-SO3H)BMIM]HSO4 could be recovered and reused without any considerable loss of catalytic activity in a test of six cycles.
Table 3 Comparison of the presented method with previous literature for the synthesis of 2-phenyl-2,3-dihydroquinazolin-4(1H)-one

|
Entry |
Catalytic system |
Reaction conditions |
Yield (%) |
1 |
[BMIM]PF6 (3.0 mL) |
75 °C, 35 min |
89 (ref. 62) |
2 |
Cp2TiCl2 (1 mol%), ethanol (0.5 mL) |
30 °C, 8 min |
96 (ref. 65) |
3 |
Fe3O4@SiO2@GMSI-VB1 (10 mg), H2O (1.0 mL) |
80 °C, 80 min |
95 (ref. 66) |
4 |
MNPs/DETA-SA (15 mg), H2O (2.0 mL) |
90 °C, 40 min |
94 (ref. 67) |
5 |
p-Sulfonic acid calix[4]arene(1.0 mol%), H2O (1.0 mL) |
r.t., 18 min |
94 (ref. 68) |
6 |
Current work: [(4-SO3H)BMIM]HSO4 (10 mol%), solvent-free |
r.t., 30 min |
98 |
On the basis of our experiments and the literature, we proposed the reaction mechanism for the cyclocondensation between anthranilamide and aromatic aldehydes (Scheme 4).56,69 The first step could be the formation of imine as a critical intermediate under the presence of [(4-SO3H)BMIM]HSO4. Next, the imine would be activated by the Brønsted acidic ionic liquid and the desired product could be obtained from the intermediate by the intramolecular nucleophilic attack of the nitrogen (–NH2) on the amide group to imine carbon.
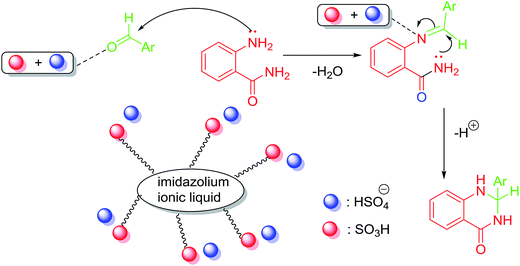 |
| Scheme 4 A plausible mechanism for the synthesis of 2,3-dihydroquinazolin-4(1H)-one. | |
Recyclability of the catalyst is a necessary feature for the application in industrial processes. The recyclability of [(4-SO3H)BMIM]HSO4 in this study was investigated in the model reactions with benzaldehyde under optimal conditions (Fig. 1). After completion of the reaction, the reaction mixture was diluted with ethanol, and the desired product was obtained by filtration. The catalyst was recovered, dried under vacuum for 6 h and reused for next cycles. The yields of the product slightly decreased after the sixth cycle. The FT-IR spectra confirmed that there was no change in functional groups of [(4-SO3H)BMIM]HSO4 (Fig. 2).
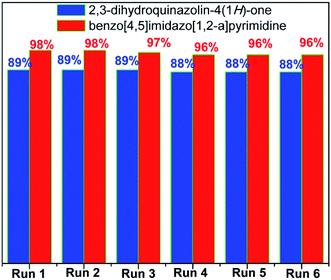 |
| Fig. 1 Recycling test. | |
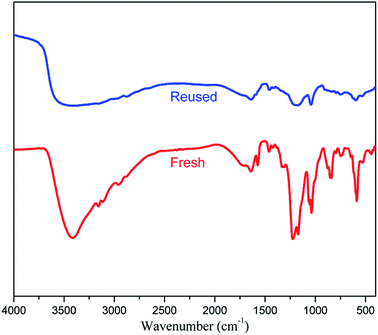 |
| Fig. 2 FT-IR spectra of the fresh and the six-times reused catalyst. | |
Conclusions
We have developed a facile, efficient, and atom-economic method for preparing benzo[4,5]imidazo[1,2-a]pyrimidines and 2,3-dihydroquinazolin-4(1H)-ones under metal- and solvent-free conditions. The presented method provides a straightforward and green approach for the preparation of biologically nitrogen-heterocyclic compounds from the readily available starting materials in good to excellent yields within short reaction time. Remarkably, [(4-SO3H)BMIM]HSO4 Brønsted acidic ionic liquid showed to be particularly suitable for these transformations as highlighted by its environmental friendliness, low-cost, recyclability, and simplicity of operation.
Experimental
Materials and instrumentation
All the reagents were purchased from Merck, Acros, and Sigma-Aldrich and used without further purification. Ultrasonic irradiation was performed in Elma sonic S30H. FT-IR spectra were analyzed using a Bruker Vertex 70 (KBr pellets). Thermogravimetric analysis was performed on Analyzer TGA Q5000. 1H and 13C NMR spectra were performed on a Bruker Advance 500. High-resolution electrospray ionization mass spectrometry (HRMS-ESI) was performed on a Bruker micrOTOF-QII MS at 80 eV.
Synthesis of [(4-SO3H)BMIM]HSO4 under solvent-free sonication
[(4-SO3H)BMIM]HSO4 was synthesized via a one-pot two-step procedure under solvent-free sonication according to our previous literature report.22
General procedure for the synthesis of benzo[4,5]imidazo[1,2-a]pyrimidines
A mixture of benzaldehyde (106 mg, 1 mmol), ethyl acetoacetate (130 mg, 1 mmol), 2-aminobenzimidazole (133 mg, 1 mmol) and [(4-SO3H)BMIM]HSO4 (31.6 mg, 0.1 mmol) was heated 100 °C and the progress of the reaction was monitored by TLC. After completion of the conversion, the reaction mixture was quenched with cold ethanol (10 mL). The crude product was filtered and washed with petroleum ether (10 mL), and then purified by recrystallization from ethanol to obtain the desired pure product.
General procedure for the synthesis 2,3-dihydroquinazolin-4(1H)-one
A mixture of anthranilamide (136 mg, 1 mmol), benzaldehyde (106 mg, 1 mmol), and [(4-SO3H)BMIM]HSO4 (31.6 mg, 0.1 mmol) was sonicated for 30 min at room temperature and the progress of the reaction was monitored by TLC or GC-MS. After completion of the conversion, the reaction mixture was quenched with ethanol (10 mL). The crude product was filtered and washed with petroleum ether (2 × 5 mL), and then purified by recrystallization from ethanol to afford the desired pure product.
Conflicts of interest
There are no conflicts to declare.
Acknowledgements
This research was funded by Viet Nam National University, Ho Chi Minh City (VNU-HCM) under grant number 562-2018-18-03.
References
- V. I. Parvulescu and C. Hardacre, Chem. Rev., 2007, 107, 2615–2665 CrossRef CAS PubMed.
- M. Zhang, R. Ettelaie, T. Yan, S. Zhang, F. Cheng, B. P. Binks and H. Yang, J. Am. Chem. Soc., 2017, 139, 17387–17396 CrossRef CAS PubMed.
- Q. Hou, M. Zhen, L. Liu, Y. Chen, F. Huang, S. Zhang, W. Li and M. Ju, Appl. Catal., B, 2018, 224, 183–193 CrossRef CAS.
- T. Akiyama and K. Mori, Chem. Rev., 2015, 115, 9277–9306 CrossRef CAS PubMed.
- M. A. Martins, C. P. Frizzo, D. N. Moreira, N. Zanatta and H. G. Bonacorso, Chem. Rev., 2008, 108, 2015–2050 CrossRef CAS PubMed.
- J. C. Schleicher and A. M. Scurto, Green Chem., 2009, 11, 694 RSC.
- H. Olivier-Bourbigou, L. Magna and D. Morvan, Appl. Catal., A, 2010, 373, 1–56 CrossRef CAS.
- J. P. Hallett and T. Welton, Chem. Rev., 2011, 111, 3508–3576 CrossRef CAS PubMed.
- R. L. Vekariya, J. Mol. Liq., 2017, 227, 44–60 CrossRef CAS.
- A.-O. Diallo, G. Fayet, C. Len and G. Marlair, Ind. Eng. Chem. Res., 2012, 51, 3149–3156 CrossRef CAS.
- A. O. Diallo, C. Len, A. B. Morgan and G. Marlair, Sep. Purif. Technol., 2012, 97, 228–234 CrossRef CAS.
- A.-O. Diallo, A. B. Morgan, C. Len and G. Marlair, Energy Environ. Sci., 2013, 6, 699–710 RSC.
- A. Bado-Nilles, A. O. Diallo, G. Marlair, P. Pandard, L. Chabot, A. Geffard, C. Len, J. M. Porcher and W. Sanchez, J. Hazard. Mater., 2015, 283, 202–210 CrossRef CAS PubMed.
- M. A. Martins, C. P. Frizzo, A. Z. Tier, D. N. Moreira, N. Zanatta and H. G. Bonacorso, Chem. Rev., 2014, 114, PR1–PR70 CrossRef PubMed.
- A. S. Amarasekara, Chem. Rev., 2016, 116, 6133–6183 CrossRef CAS PubMed.
- A. C. Cole, J. L. Jensen, I. Ntai, K. L. T. Tran, K. J. Weaver, D. C. Forbes and J. H. Davis, J. Am. Chem. Soc., 2002, 124, 5962–5963 CrossRef CAS PubMed.
- H. Zhang, F. Xu, X. Zhou, G. Zhang and C. Wang, Green Chem., 2007, 9, 1208–1211 RSC.
- J. Yang, H. Zhou, X. Lu and Y. Yuan, Catal. Commun., 2010, 11, 1200–1204 CrossRef CAS.
- J. Long, W. Lou, L. Wang, B. Yin and X. Li, Chem. Eng. Sci., 2015, 122, 24–33 CrossRef CAS.
- A. Wang, X. Zheng, Z. Zhao, C. Li, Y. Cui, X. Zheng, J. Yin and G. Yang, Appl. Catal., A, 2014, 482, 198–204 CrossRef CAS.
- Q. Peng, K. Mahmood, Y. Wu, Z. Liu, L. Wei, H. Yuan and R. Yang, Mol. Catal., 2017, 434, 140–145 CrossRef CAS.
- P. H. Tran, A.-T. Duy Nguyen, H. T. Nguyen and T. N. Le, RSC Adv., 2017, 7, 54399–54406 RSC.
- Q. T. Nguyen, A.-H. Thi Hang, T.-L. Ho Nguyen, D.-K. Nguyen Chau and P. H. Tran, RSC Adv., 2018, 8, 11834–11842 RSC.
- P. H. Tran, X.-T. T. Nguyen and D.-K. N. Chau, Asian J. Org. Chem., 2018, 7, 232–239 CrossRef CAS.
- T. Raj, H. Sharma, Mayank, A. Singh, T. Aree, N. Kaur, N. Singh and D. O. Jang, ACS Sustainable Chem. Eng., 2017, 5, 1468–1475 CrossRef CAS.
- A. Shaabani, A. Rahmati and S. Naderi, Bioorg. Med. Chem. Lett., 2005, 15, 5553–5557 CrossRef CAS PubMed.
- W. Kong, Y. Zhou and Q. Song, Adv. Synth. Catal., 2018, 360, 1943–1948 CrossRef CAS.
- S. Tardy, A. Orsato, L. Mologni, W. H. Bisson, C. Donadoni, C. Gambacorti-Passerini, L. Scapozza, D. Gueyrard and P. G. Goekjian, Bioorg. Med. Chem., 2014, 22, 1303–1312 CrossRef CAS PubMed.
- R. Garamvolgyi, J. Dobos, A. Sipos, S. Boros, E. Illyes, F. Baska, L. Kekesi, I. Szabadkai, C. Szantai-Kis, G. Keri and L. Orfi, Eur. J. Med. Chem., 2016, 108, 623–643 CrossRef CAS PubMed.
- S. J. Kalita, D. Chandra Deka and H. Mecadon, RSC Adv., 2016, 6, 91320–91324 RSC.
- K. B. Puttaraju, K. Shivashankar, Chandra, M. Mahendra, V. P. Rasal, P. N. Venkata Vivek, K. Rai and M. B. Chanu, Eur. J. Med. Chem., 2013, 69, 316–322 CrossRef CAS PubMed.
- T. H. Al-Tel and R. A. Al-Qawasmeh, Eur. J. Med. Chem., 2010, 45, 5848–5855 CrossRef CAS PubMed.
- M. Abedini, F. Shirini, M. Mousapour and O. Goli Jolodar, Res. Chem. Intermed., 2016, 42, 6221–6229 CrossRef CAS.
- I. M. Abbas, M. A. Abdallah, S. M. Gomha and M. S. H. Kazem, J. Heterocycl. Chem., 2017, 54, 3447–3457 CrossRef CAS.
- F. Bellina, R. Rossi, M. Lessi, C. Manzini and L. Perego, Synthesis, 2014, 46, 2833–2883 CrossRef.
- C. Yao, S. Lei, C. Wang, T. Li, C. Yu, X. Wang and S. Tu, J. Heterocycl. Chem., 2009, 47, 26–32 Search PubMed.
- N. S. El-Gohary and M. I. Shaaban, Eur. J. Med. Chem., 2013, 63, 185–195 CrossRef CAS PubMed.
- M. Rawat and D. S. Rawat, Tetrahedron Lett., 2018, 59, 2341–2346 CrossRef CAS.
- M. V. Reddy, A. V. S. Reddy and Y. T. Jeong, Res. Chem. Intermed., 2016, 42, 4893–4906 CrossRef CAS.
- H. R. Shaterian, N. Fahimi and K. Azizi, Res. Chem. Intermed., 2013, 40, 1879–1898 CrossRef.
- J. Liu, M. Lei and L. Hu, Green Chem., 2012, 14, 840–846 RSC.
- O. Rosati, M. Curini, F. Montanari, M. Nocchetti and S. Genovese, Catal. Lett., 2011, 141, 850–853 CrossRef CAS.
- A. M. Jadhav, Y. I. Kim, K. T. Lim and Y. T. Jeong, Tetrahedron Lett., 2018, 59, 554–557 CrossRef CAS.
- T. A. Shah, W. Raza, M. Muneer, N. A. Mir and M. Ahmad, ChemistrySelect, 2018, 3, 946–950 CrossRef CAS.
- A. Kamal, E. V. Bharathi, J. S. Reddy, M. J. Ramaiah, D. Dastagiri, M. K. Reddy, A. Viswanath, T. L. Reddy, T. B. Shaik, S. N. Pushpavalli and M. P. Bhadra, Eur. J. Med. Chem., 2011, 46, 691–703 CrossRef CAS PubMed.
- M. Rueping, A. P. Antonchick, E. Sugiono and K. Grenader, Angew. Chem., Int. Ed. Engl., 2009, 48, 908–910 CrossRef CAS PubMed.
- G. H. Zhang, J. M. Yuan, G. Qian, C. X. Gu, K. Wei, D. L. Mo, J. K. Qin, Y. Peng, Z. P. Zhou, C. X. Pan and G. F. Su, J. Med. Chem., 2017, 60, 6853–6866 CrossRef CAS PubMed.
- S. Y. Abbas, K. A. M. El-Bayouki, W. M. Basyouni and E. A. Mostafa, Med. Chem. Res., 2017, 27, 571–582 CrossRef.
- D. Kumar, G. Mariappan, A. Husain, J. Monga and S. Kumar, Arabian J. Chem., 2017, 10, 344–350 CrossRef CAS.
- H. K. Kumara, R. Suhas, D. M. Suyoga Vardhan, M. Shobha and D. Channe Gowda, RSC Adv., 2018, 8, 10644–10653 RSC.
- S. Poudapally, V. Gurram, R. Garlapati, C. Tulluri, U. Addepally, K. Vidya, S. Sharma, S. Sen and N. Pottabathini, J. Heterocycl. Chem., 2017, 54, 2272–2286 CrossRef CAS.
- A. A. Abdel-Aziz, L. A. Abou-Zeid, K. E. H. ElTahir, R. R. Ayyad, M. A. El-Sayed and A. S. El-Azab, Eur. J. Med. Chem., 2016, 121, 410–421 CrossRef CAS PubMed.
- A. A. Abdel-Aziz, L. A. Abou-Zeid, K. E. ElTahir, M. A. Mohamed, M. A. Abu El-Enin and A. S. El-Azab, Bioorg. Med. Chem., 2016, 24, 3818–3828 CrossRef CAS PubMed.
- M. Badolato, F. Aiello and N. Neamati, RSC Adv., 2018, 8, 20894–20921 RSC.
- M. Dabiri, P. Salehi, M. Baghbanzadeh, M. A. Zolfigol, M. Agheb and S. Heydari, Catal. Commun., 2008, 9, 785–788 CrossRef CAS.
- M. Sharma, S. Pandey, K. Chauhan, D. Sharma, B. Kumar and P. M. Chauhan, J. Org. Chem., 2012, 77, 929–937 CrossRef CAS PubMed.
- V. F. Vavsari and G. M. Ziarani, Chem. Heterocycl. Compd., 2018, 54, 317–319 CrossRef CAS.
- D. He, M. Wang, S. Zhao, Y. Shu, H. Zeng, C. Xiao, C. Lu and Y. Liu, Fitoterapia, 2017, 119, 136–149 CrossRef CAS PubMed.
- U. Kshirsagar and R. Rohokale, Synthesis, 2016, 48, 1253–1268 CrossRef.
- I. Khan, S. Zaib, S. Batool, N. Abbas, Z. Ashraf, J. Iqbal and A. Saeed, Bioorg. Med. Chem., 2016, 24, 2361–2381 CrossRef CAS PubMed.
- M. Zahedifard, F. L. Faraj, M. Paydar, C. Yeng Looi, M. Hajrezaei, M. Hasanpourghadi, B. Kamalidehghan, N. Abdul Majid, H. Mohd Ali and M. Ameen Abdulla, Sci. Rep., 2015, 5, 11544–11560 CrossRef PubMed.
- J. Chen, W. Su, H. Wu, M. Liu and C. Jin, Green Chem., 2007, 9, 972–975 RSC.
- J. Wang, Y. Zong, R. Fu, Y. Niu, G. Yue, Z. Quan, X. Wang and Y. Pan, Ultrason. Sonochem., 2014, 21, 29–34 CrossRef CAS PubMed.
- S. Nagarajan, T. M. Shaikh and E. Kandasamy, New J. Chem., 2015, 39, 9693–9699 RSC.
- Y. Luo, Y. Wu, Y. Wang, H. Sun, Z. Xie, W. Zhang and Z. Gao, RSC Adv., 2016, 6, 66074–66077 RSC.
- N. Azizi, F. Abbasi and M. Abdoli-Senejani, Mater. Chem. Phys., 2017, 196, 118–125 CrossRef CAS.
- L. Shiri, H. Narimani and M. Kazemi, Appl. Organomet. Chem., 2018, 32, e3999 CrossRef.
- M. Rahman, I. Ling, N. Abdullah, R. Hashim and A. Hajra, RSC Adv., 2015, 5, 7755–7760 RSC.
- N. Y. Kim and C.-H. Cheon, Tetrahedron Lett., 2014, 55, 2340–2344 CrossRef CAS.
Footnote |
† Electronic supplementary information (ESI) available. See DOI: 10.1039/c8ra07256f |
|
This journal is © The Royal Society of Chemistry 2018 |