DOI:
10.1039/C8RA06819D
(Paper)
RSC Adv., 2018,
8, 38186-38195
Green synthesis of Au nanoparticles using an aqueous extract of Stachys lavandulifolia and their catalytic performance for alkyne/aldehyde/amine A3 coupling reactions†
Received
14th August 2018
, Accepted 31st October 2018
First published on 14th November 2018
Abstract
High reaction rate and easy availability make green synthesis of metal nanoparticles noticeable. In the present study, gold nanoparticles with wide applications in different fields were synthesized by an ecofriendly method at room temperature using Stachys lavandulifolia extract as the reducing agent. Properties of the synthesized gold nanoparticles (GNP) were identified by different analytical techniques including: UV-Vis absorption spectroscopy verified presence of Au NPs in the solution while functional groups of its extract and synthesized Au NPs were determined by FT-IR. Its crystalline analysis with a fcc plane was verified by X-ray diffraction (XRD) and energy dispersive spectroscopy (EDS) determined elements in the sample. Surface morphology, diverse shapes and sizes of the Au NPs were shown by scanning electron microscopy (SEM), atomic force microscopy (AFM), and transmission electron microscopy (TEM). Beginning and end destruction temperatures of the Au/S. lavandulifolia NPs were determined by thermal gravimetric analysis (TGA). The Au nanoparticles were capped with extracts, preventing them from oxidation and agglomeration and were used as an efficient heterogeneous nanocatalyst for a three-component reaction of amines, aldehydes, and alkynes (A3 coupling). A diverse range of propargylamines were obtained in good yields. Furthermore, the separation and recycling of Au/S. lavandulifolia NPs was very simple, effective, and economical.
1. Introduction
In general, propargylamines are useful in organic chemistry as precursors and versatile building blocks for preparing different nitrogen-comprising heterocyclic compounds as well as main intermediates for synthesizing biologically active medicines and natural products.1 Additionally, several propargylamines have been applied to treat neuropsychiatric diseases including Parkinson's and Alzheimer's disorders.2 Due to their importance, many synthetic methods have been developed.3 However, the most direct and efficient method for preparation of propargylamines is through transition metal-catalyzed three component coupling of an aldehyde, an amine, and a terminal alkyne, which is known as an A3 coupling reaction.4 In recent years, various homogeneous and heterogeneous catalysts have been employed in the synthesis of propargylamine via an A3-coupling reaction based on transition metals including Zr,5 Mn,6 Re,7 Fe,8 Ru,9 Co,10 Ir,11 Ni,12 Pd,13 Cu,14 Ag,15 Au,16 Zn,17 Cd,18 and Hg.19 Among the different transition metals, copper has been widely studied because of its abundance, low cost, low toxicity, and high reactivity. Although the majority of the catalytic systems were homogeneous, their loss at the end of a reaction reduces their utility especially for industrial applications. Nowadays, metal NPs are considered as heterogeneous catalysts which possess a high surface-to-volume ratio. Because of their enhanced activity and selectivity, they could be a competitive alternative to classical catalysis.20,21 Diverse models of traditional chemical22 and green chemistry23,24 approaches were applied to produce metal NPs. Amongst all metallic NPs, Au NPs have been widely used in different fields including medicine and gene delivery,27 imaging,28 heating,29 labeling, sensing,30 and catalysis31 because of their unique optical features.25,26 Applications of green chemistry comprising diverse parts of herbal extracts such as leaves,32 fruit,33 roots,34 and seeds35 as a reducing agent for synthesizing gold NPs makes them very useful because they have no hazardous materials and are very cheap. Amongst 34 Stachys spices, growing in many regions of Iran, Turkey, and Iraq,36,37 Stachys lavandulifolia (Fig. 1) is used as an herbal tea in Iran for gastrointestinal disorders and extracts of this plant were introduced to have an anxiolytic effect with a lower sedative activity than diazepam.38 Stachys lavandulifolia extracts fractions including flavonoids, phenylpropanoids, polyphenols, and terpenoids from the aerial parts of plants were considered to play an important role in anxiolytic effects.39 Acteoside, as one of the secondary metabolites isolated from S. lavandulifolia aerial part's extracts, is displayed in Fig. 1. Significantly, green preparation of NPs has up-surged as a new nanobiotechnology to produce eco-friendly and inexpensive synthetic procedures for extremely stable NPs and it has emerged as a safer and best alternative to conventional approaches. Due to our ongoing interest on the biosynthesis of metal NPs and heterogeneous catalysts,40,41 in the current study Au NPs were prepared for the first time using Stachys lavandulifolia through a green method and their morphological, structural, and catalytic applications were studied in detail.
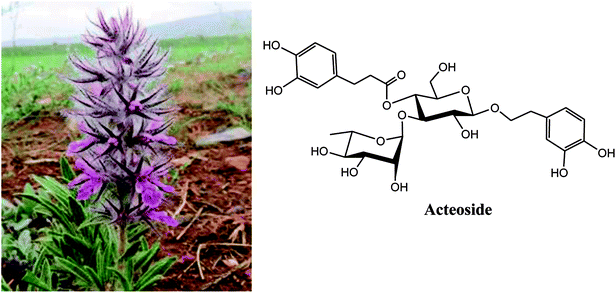 |
| Fig. 1 Stachys lavandulifolia image and acteoside structure. | |
2. Experimental
2.1. Materials and apparatus
All the reagents were purchased from Aldrich and Merck and were used without any purification. Crystalline structures of the samples were evaluated by X-ray diffraction (XRD) analysis on a Bruker D8 Advance Diffractometer with CuKα radiation at 40 kV and 20 mA. Fourier transform infrared (FT-IR) spectra were recorded using KBr pellets with a PerkinElmer 65 spectrophotometer in the range of 400–4000 cm−1. TEM images at an accelerating voltage of 80 KV were taken with a Zeiss-EM10C. Morphology and particle dispersion was investigated by field emission scanning electron microscopy (FESEM) (Cam Scan MV2300). Chemical composition of the prepared nanostructures was measured by EDS (Energy Dispersive X-ray Spectroscopy) performed in SEM. The UV-Vis absorbance spectra were recorded using a double beam UV-visible spectrophotometer (PG Instrument, T80+), equipped with 10 mm quartz cuvettes. The spectra were obtained using a ThermoFisher Scientific K-Alpha XPS spectrometer. Survey spectra were initially obtained at low energy resolution (pass energy −200 eV) followed by the main regions of interest at higher resolution (pass energy −25 eV). A monochromatic Al Kα X-ray was used, with a nominal spot size of 400 μm.
2.2. Preparation of Stachys lavandulifolia extract
Freshly collected herbal tea (10 g) from the Kermanshah, Zagrous region, was washed, dried, and used for preparation of the Stachys lavandulifolia extract. The tea (10 g) was added to 100 mL of Milli-Q water and heated at 60 °C for 15 min. Then, the extract was filtered through Whatman no. 1 filter paper and centrifuged at 6000 rpm for 5 min to remove unwanted aggregates. The filtered extract was stored in a refrigerator at 4 °C for further use.
2.3. Green synthesis of Au NPs using Stachys lavandulifolia extract
Different amounts of the Stachys lavandulifolia extract (5, 10, 15, and 20 mL) were added to an aqueous solution of (1 mM) HAuCl4 × H2O (100 mL) at room temperature and stirred for the purpose of studying time effects and extract concentrations on synthesis of gold nanoparticles. The color of the solution turned red during 2 h and the high concentration of extract created a darker red (Fig. 1S†). Then, solutions containing the nanoparticles were centrifuged at 12
000 rpm for 15 min and the upper phase was removed. Obtained nanoparticles were washed for several times with deionized water and finally dried in an oven at 50 °C.
2.4. General procedure for the synthesis of propargylamine derivatives
The Au NPs catalyst (10 mol%) was added to a mixture of aldehyde (1 mmol), phenylacetylene (1 mmol), and amine (1.2 mmol) in toluene (5 mL) and the mixture was stirred at 100 °C. The progress of the reaction was monitored by TLC. After complete conversion of the aldehyde, the mixture was left to cool down to room temperature and then centrifuged. After evaporation of the solvent, the crude product was obtained. Purification was performed by silica gel column chromatography (70
:
30, hexane/EtOAc). All compounds were known and were characterized by spectral analysis or melting points.48–61
3. Results and discussion
A UV-Vis spectroscopy (PerkinElmer, Lambda 25) instrument at wavelengths of 300–700 nm was used to confirm dimensions and effective preparation of gold NPs. Fig. 2 shows the results from comparison of UV-Vis spectra of green synthesized of Au NPs using Stachys lavandulifolia extract with diverse levels of extracts at 5, 10, 15, and 20 mL. These results confirm the faster formation of Au NPs in the solution by enhancing contents of the extract. As observed, the amounts of NPs prepared were enhanced over time with all levels of extract.
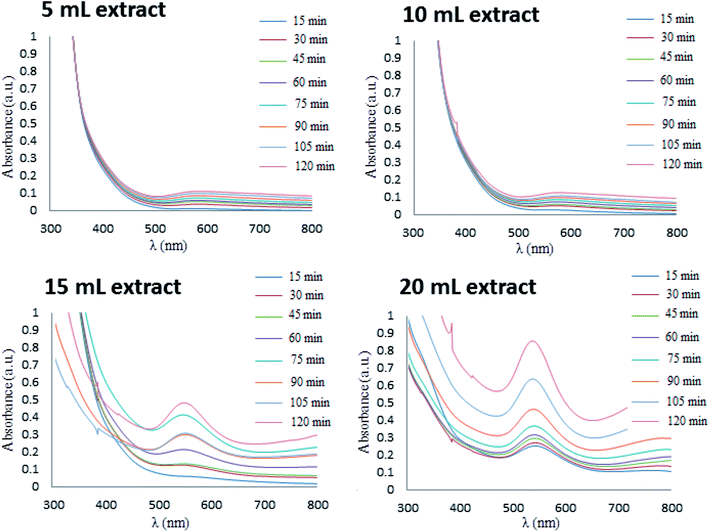 |
| Fig. 2 Extract concentrations and time effect on UV-Vis spectroscopy of synthesized gold nanoparticles. | |
FT-IR measurements ranging from 400 to 4000 cm−1 were applied to identify the responsible phytochemicals during green synthesis of the Au NPs. The FT-IR spectrum of the pure extract, (Fig. 2Sa†) showed several peaks at 3369 cm−1, 2923 cm−1, 1618 cm−1, and 1400 cm−1 corresponding to free OH and OH groups creating hydrogen bonds, saturated hydrocarbons (Csp3-H), carbonyl group (C
O), and stretching C
C aromatic ring, respectively. Due to existence of these functional groups in the structure of antioxidant polyphenolics, the spectrum proves the existence of phenolics in the herbal extract and supports the outcomes of relevant research.42 Moreover, an FT-IR spectrum corresponding to Au NPs is presented in Fig. 2Sb.† The peaks from Au/S. lavandulifolia NPs are nearly identical to Fig. 2Sa† and its representation of the functional groups of biomolecules adsorbed on NPs. The broad band at 3418 cm−1 can be attributed to a stretching bond of the hydroxyl functional group and the band near 1447 cm−1 is normally assigned to the bending vibration corresponding to sp2-carbon groups of aromatics and 1628 cm−1 for a carbonyl functional group.
The structure of prepared Au NPs also was analyzed with XRD by means pf a Bruker AXS-D8 Advance instrument working at a voltage of 40 kV and current of 30 mA with CuK radiation. The crystalline feature of Au NPs was confirmed with an X-ray intensity which reflected from crystals which are highest at certain angles. Diffraction peaks appeared at 38.067, 44.215, 64.291, and 77.592 in a 2θ range 100–80° relating to (111), (200), (220), and (311) facets of a face centered cubic crystal structure (JCPDS. no. 004-0784) and showed the crystalline structure of prepared Au NPs with Stachys lavandulifolia extract (Fig. 3).
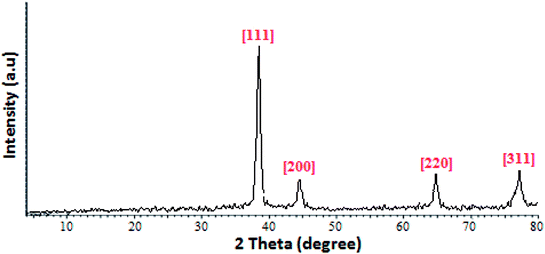 |
| Fig. 3 XRD spectrum of Au/S. lavandulifolia NPs. | |
Energy Dispersive X-ray Spectroscopy (TESCAN Vega, USA) verified the existence and purity of particles in the specimens. Fig. 4's EDX spectrum of prepared Au NPs shows a strong signal that reveals presence of pure metallic gold NPs.
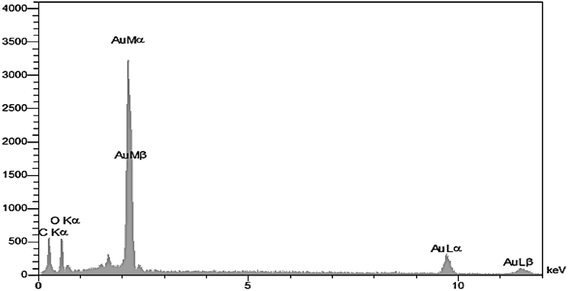 |
| Fig. 4 EDS image of Au/S. lavandulifolia NPs. | |
A scanning electron microscope (TESCAN Vega Model) was applied for identifying morphology and aggregates of NPs. According to SEM images of Au/S. lavandulifolia NPs presented in Fig. 3S,† nanoparticles were formed and their shape was somewhat spherical in nature.
Atomic force microscopy (AFM) (DME-95-50 E) was used to recognize topological appearance and sizes of NPs. Fig. 5 is an AFM image corresponding to GNP where height measurement indicates sizes ranging from 22 to 30 nm and also represents rough surface of NPs.
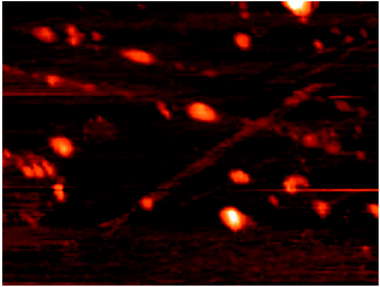 |
| Fig. 5 AFM images of Au/S. lavandulifolia NPs. | |
High resolution transmission electron microscopy (HR-TEM) was applied to determine the dimension and shape of NPs. Usual TEM images achieved for colloids are presented in Fig. 6. According to the image, it is obvious that gold NPs have a nearly spherical and triangular morphology with an excellent distribution of particles with sizes between 20 and 30 nm. Moreover, Fig. 6 shows a HR-TEM image corresponding to the Au NPs at 5 nm magnification. Based on this image, the Au NPs contained lattice fringes, which confirmed their great crystallinity. Meantime, a Fast Fourier Transform (FFT) image corresponding to the Au NPs (inset) shows bright diffraction spots and ring patterns that prove the crystalline nature of the Au atoms.
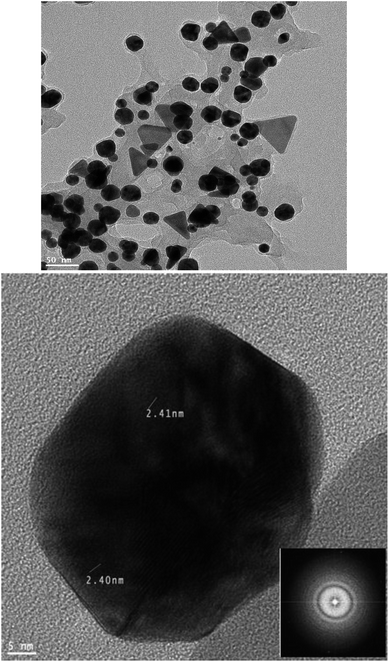 |
| Fig. 6 HR-TEM images of Au/S. lavandulifolia NPs and FFT image corresponding to Au NPs (inset). | |
Thermal gravimetric analysis (Netzsch-TGA 209 F1) is an important method to determine beginning and end destruction temperatures of nanoparticles (heat-resistance) and amounts of weight loss during heating at different temperatures. The beginning part of the graph that is straight (Fig. 7) shows that biosynthesized Au NPs are pure (free of impurities and moisture). The earliest weight loss for the synthesized gold nanoparticles occurred at 190 °C and shows a steady weight loss in the temperature range from 180–620 °C with a total weight loss up to 700 °C which is about 52.62% and occurred at 190 °C (4.01%), 390 °C, (16.89%), and 640 °C (31.71%).
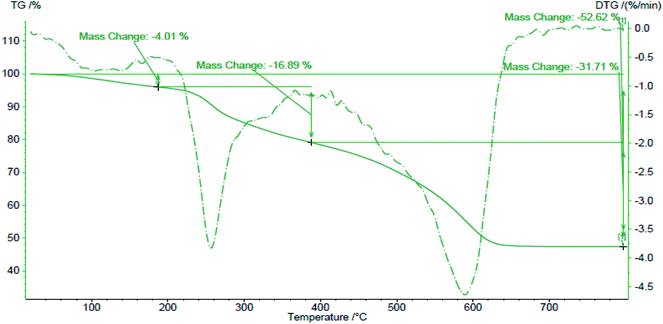 |
| Fig. 7 TGA analysis of Au/S. lavandulifolia NPs. | |
Polyphenolic compounds have been revealed as a reducing agent during the biological extract mediated synthesis of nanomaterials.44 Moreover, a rich source for polyphenolic compounds and flavonoid groups is the Stachys lavandulifolia extract.45–47 These polyphenolic compounds (such as acteoside) may play a role as reducing agents to reduce Au3+ ions. Scheme 1 is a schematic representation of possible pathways. In the first phase, as the extract combines with the metal salt solution, hydroxyl groups of the polyphenolic compounds could be reduced into Au atoms. Then, synthesized Au atoms nucleate followed by more growth which causes the NPs formation.
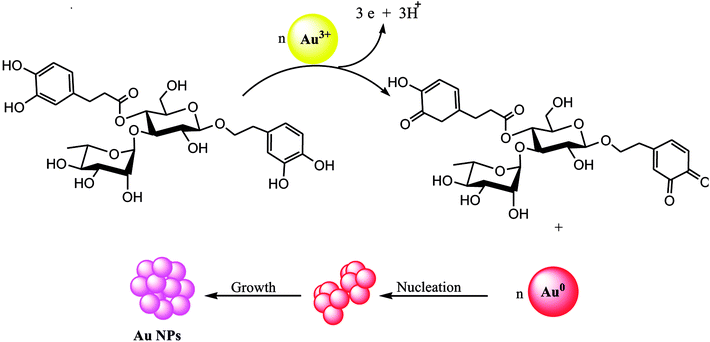 |
| Scheme 1 Probable mechanism for formation of Au NPs using Stachys lavandulifolia extract. | |
The catalytic behaviour of Au/S. lavandulifolia NPs was studied for the A3-coupling reaction of aldehydes, amines, and alkynes to produce propargylamines. Initially, we employed benzaldehyde (1.0 mmol), morpholine (1.0 mmol), and phenylacetylene (1.2 mmol) as model substrates for optimizing different factors including time, temperature, solvents, and catalyst loading. The results are presented in Table 1. First, influence of the catalyst was studied, and as expected, the desired product was not achieved without the catalyst, representing the essential role of Au/S. lavandulifolia NPs in the reaction mechanism (Table 1, entry 1). The reaction yield achieved 75% by utilizing a 10 mol% catalyst loading in toluene at 60 °C (Table 1, entry 2). Furthermore, the reaction occurred quantitatively when applying 10 mol% of catalyst at 100 °C (Table 1, entry 3). Lower yields were obtained by decreasing the catalyst level to 7 and 5 mol%, respectively (Table 1, entries 4 and 5). Increasing the catalyst level to 12 mol% did not improve the reaction yield or time (Table 1, entry 6). Applying 10 mol% of catalyst in other solvents including CH2Cl2, DMF, EtOH, H2O, CH3CN, and neat, resulted in lower reaction yields (Table 1, entries 7–12). Consequently, we chose toluene as the optimum solvent, 10 mol% catalyst loading and 100 °C reaction temperature as the most effective and optimum reaction conditions for studying the scope of this A3 coupling.
Table 1 Optimization of various conditions in the model reaction using Au/S. lavandulifolia NPs catalysta
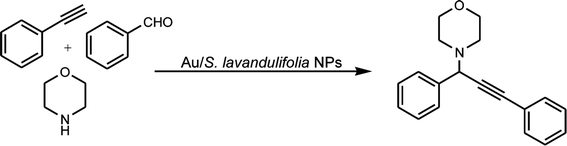
|
Entry |
Catalyst (mol%) |
Solvent |
T (°C) |
Yieldb (%) |
Reaction conditions: benzaldehyde (1.0 mmol), morpholine (1 mmol), phenylacetylene (1.2 mmol), Au/S. lavandulifolia NPs, solvent (5.0 mL) for 8 h. Yields are based on 1H NMR. |
1 |
— |
Toluene |
60 |
— |
2 |
10 |
Toluene |
60 |
75 |
3 |
10 |
Toluene |
100 |
92 |
4 |
7 |
Toluene |
100 |
65 |
5 |
5 |
Toluene |
100 |
50 |
6 |
12 |
Toluene |
100 |
92 |
7 |
10 |
CH2Cl2 |
70 |
30 |
8 |
10 |
DMF |
100 |
70 |
9 |
10 |
EtOH |
80 |
65 |
10 |
10 |
H2O |
100 |
40 |
11 |
10 |
CH3CN |
70 |
65 |
12 |
10 |
— |
100 |
30 |
Under the optimal reaction conditions, other aldehydes were reacted with different aliphatic amines and phenylacetylene and resulted A3 coupling products with good yields (Table 2). In the primary study of the aldehyde substrate's scope, morpholine, and phenylacetylene were utilized as model substrates and different aldehydes were studied for the A3-coupling reactions (Table 2, entries 1–20). Results showed that aromatic aldehyde's behaviors with functional groups like –Cl, –Br, –OH, –Me, or –OMe could impact the A3-coupling. Moreover, a minor electronic effect was found which is related to reaction of aryl aldehydes with electron-withdrawing groups (Table 2, entries 2–4) and generation of relevant products in excellent yields, while replacement of electron rich groups (Table 2, entries 5–7) on the benzene ring reduced performance and caused lesser yields. Additionally, reactions with challenging heterocyclic compounds such as thiophene-2-carbaldehyde or furan-2-carboxaldehyde with morpholine and phenylacetylene proceeded efficiently and the corresponding propargylamines were obtained in good yields (Table 2, entries 8 and 9). On the other hand, an aliphatic aldehyde (i.e., cyclohexanecarbaldehyde or butyraldehyde) also showed good yields under this optimum condition (Table 2, entries 10 and 11). To expand the scope of amine substrates a mixture of benzaldehyde–phenylacetylene–amine was selected and different amines were studied (Table 2, entries 1 and 12–16). The results indicate that cyclic, heterocyclic (i.e., piperidine, pyrrolidine, or morpholine), and acyclic (i.e., diethyl or dibenzyl) secondary amines also provided high yields of products under the optimal reaction conditions (Table 2, entries 1 and 12–15). However, no product was found when using aniline as a substrate (Table 2, entry 16). Remarkably, reaction of the aliphatic alkyne 1-octyne, a substrate which is usually hard to activate, also worked well and resulted in a product with a good yield under optimal conditions (Table 2, entries 17–20).
Table 2 The reactions of aldehydes, amines, and alkynes in the presence of Au/S. lavandulifolia NPs catalysta
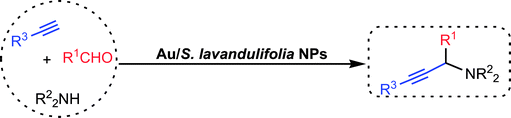
|
Entry |
R1 |
Amine |
R3 |
Yieldb (%) |
Ref.c |
Reaction conditions: aldehyde (1.0 mmol), amine (1 mmol), alkyne (1.2 mmol), and Au/S. lavandulifolia NPs (10 mol%) were stirred in toluene (5.0 mL) at 100 °C for 8 h. Yields are based on 1H NMR. Earlier reference of the corresponding product. |
1 |
Ph |
Morpholine |
Ph |
95 |
48 |
2 |
4-ClC6H4 |
Morpholine |
Ph |
96 |
59 |
3 |
3-ClC6H4 |
Morpholine |
Ph |
95 |
61 |
4 |
4-BrC6H4 |
Morpholine |
Ph |
96 |
51 |
5 |
4-OHC6H4 |
Morpholine |
Ph |
90 |
59 |
6 |
4-MeC6H4 |
Morpholine |
Ph |
88 |
48 |
7 |
4-OMeC6H4 |
Morpholine |
Ph |
85 |
59 |
8 |
2-Thiophenyl |
Morpholine |
Ph |
92 |
43 |
9 |
2-Furfuryl |
Morpholine |
Ph |
90 |
48 |
10 |
Cyclohexyl |
Morpholine |
Ph |
90 |
59 |
11 |
C3H7 |
Morpholine |
Ph |
85 |
59 |
12 |
Ph |
Piperidine |
Ph |
95 |
59 |
13 |
Ph |
Pyrrolidine |
Ph |
95 |
60 |
14 |
Ph |
Diethyl |
Ph |
90 |
59 |
15 |
Ph |
Dibenzyl |
Ph |
90 |
59 |
16 |
Ph |
Aniline |
Ph |
0 |
59 |
17 |
Ph |
Morpholine |
n-C6H13 |
82 |
49 |
18 |
Ph |
Piperidine |
n-C6H13 |
80 |
59 |
19 |
4-ClC6H4 |
Morpholine |
n-C6H13 |
85 |
59 |
20 |
4-OMeC6H4 |
Morpholine |
n-C6H13 |
70 |
59 |
According to the above outcomes, a suggested reaction pathway for the A3 coupling reaction catalyzed by Au/S. lavandulifolia NPs under heating conditions is presented in Scheme 2. The first phase involves activating the terminal alkyne using the Au NPs catalyst under heating to result in the related Au–alkylidine complex on the surface of NPs. Next, the reaction between an Au–acetylide intermediate with iminium ion (formed in situ from the related aldehyde and amine) occurs to result in the desired propargylamine, with the Au/S. lavandulifolia NPs catalyst being reformed for a further cycle of reactions.48
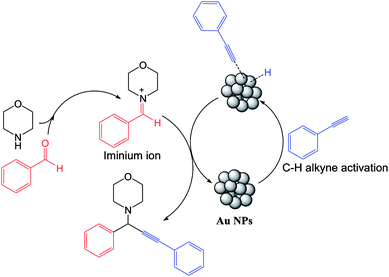 |
| Scheme 2 Proposed mechanism for the A3 coupling reaction catalyzed by Au/S. lavandulifolia NPs. | |
Recovering heterogeneous new catalysts is extremely vital in terms of economic and sustainable chemistry views. Consequently, we investigated recovery of the Au/S. lavandulifolia NPs catalyst for the reaction of benzaldehyde, morpholine, and phenylacetylene under optimum reaction conditions. For this goal, at the end of the reaction, the catalyst was isolated from the reaction solution through centrifugation and rinsed with water and ethanol twice, and then was recycled for seven times with no significant change in catalytic performance (Fig. 8). This reusability proves the excellent stability and turnover of the catalyst under working conditions.
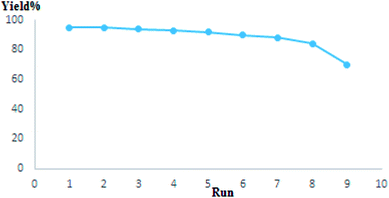 |
| Fig. 8 Recycling of catalyst for the reaction of benzaldehyde, morpholine, and phenylacetylene. | |
Several chosen procedures in the literature and current procedures were compared in Table 3, which indicates that Au/S. lavandulifolia NPs are a similar or a more effective catalyst in terms of reaction time and yield than earlier reported ones.
Table 3 Comparison efficiency of Au/S. lavandulifolia NPs with some reported methods for the A3 coupling reaction between benzaldehyde and piperidine with phenylacetylene
Entry |
Reaction conditions |
Time (h) |
Yield % |
Ref. |
1 |
Au/S. lavandulifolia NPs, toluene, 100 °C |
8 |
95 |
This work |
2 |
AgI, H2O, 100 °C, N2 |
14 |
70 |
49 |
3 |
CuSBA-15, toluene, 90 °C |
6 |
80 |
50 |
4 |
Au@PMO-IL, CHCl3, 60 °C |
12 |
87 |
51 |
5 |
FeCl3, 70 °C |
14 |
34 |
52 |
6 |
Ag NPs, PEG, 100 °C |
20 |
77 |
53 |
7 |
Nano Co3O4, 130 °C |
15 |
87 |
54 |
8 |
Fe3O4, toluene, 80 °C |
16 |
75 |
55 |
9 |
Fe3O4@SBA-15, toluene, 110 °C |
8 |
76 |
56 |
10 |
NiCl2, toluene, 111 °C |
9 |
87 |
57 |
11 |
Ag–NaY, neat, 100 °C |
15 |
73 |
58 |
12 |
Ag NPs/plant extract, PEG, 90 °C |
18 |
83 |
24 |
13 |
MNP@Au/NNN-pincer, H2O, 85 °C |
7 |
90 |
59 |
14 |
[CuCl{2,5-bis(2-thienyl)-1-phenylphosphole}2], 100 °C |
5 |
92 |
60 |
15 |
InBr3, toluene, 80 °C |
6 |
80 |
61 |
4. Conclusion
In conclusion, a green, effective, simple, and cost-effective technique has been developed for synthesizing Au NPs with Stachys lavandulifolia extract as a reducer and stabilizer. Characterization of the prepared Au/S. lavandulifolia NPs was carried out using SEM, HR-TEM, FFT, XRD, EDS, TGA, FT-IR, and UV-visible methods. It was found that Au/S. lavandulifolia NPs are cost-effective, air stable, and an effective catalyst for synthesis of propargylamines through a one-pot three-component A3-coupling reaction of aldehydes, amines, and alkynes. The products were achieved in good to high yields and the catalyst can be reused up to seven cycles with nearly no change in its performance. The important properties of the presented approach are: (i) high catalytic activity; (ii) excellent yield of the products; (iii) application of the plant extract as a fast and clean synthetic pathway for synthesis of Au NPs; (iv) no surfactant, capping agent, and/or template were utilized in the process; and (v) the Au NPs can be simply retained and reused.
Conflicts of interest
There are no conflicts to declare.
Acknowledgements
We are thankful to Payame Noor University (PNU) for financial supports.
References
-
(a) Y. Liu, ARKIVOC, 2014, 1, 1 Search PubMed;
(b) E. Vessally, RSC Adv., 2016, 6, 18619 RSC;
(c) E. Vessally, L. Edjlali, A. Hosseinian, A. Bekhradnia and M. D. Esrafili, RSC Adv., 2016, 6, 49730 RSC;
(d) I. Matsuda, J. Sakakibara and H. Nagashima, Tetrahedron Lett., 1991, 32, 7431 CrossRef CAS;
(e) C. P. T. Sar, T. Kalai, J. Jeko and K. Hideg, ARKIVOC, 1991, 32, 47 Search PubMed.
-
(a) P. H. Yu, B. A. Davis and A. A. Boulton, J. Med. Chem., 1992, 35, 3705 CrossRef CAS PubMed;
(b) J. J. Chen, D. M. Swope and K. Dashtipour, Clin. Ther., 2007, 29, 1825 CrossRef CAS PubMed;
(c) M. Baranyi, P. F. Porceddu, F. Goloncser, S. Kulcsar, L. Otrokocsi, A. Kittel, A. Pinna, L. Frau, P. B. Huleatt, M. L. Khoo, C. L. L. Chai, P. Dunkel, P. Matyus, M. Morelli and B. Sperlagh, Mol. Neurodegener., 2016, 11, 2 CrossRef PubMed.
-
(a) G. Magueur, B. Crousse and D. Bonnet-Delpon, Tetrahedron Lett., 2005, 46, 2219 CrossRef CAS;
(b) P. Kaur, G. Shakya, H. Sun, Y. Pan and G. Li, Org. Biomol. Chem., 2010, 8, 1091 RSC;
(c) F. Colombo, M. Benaglia, S. Orlandi, F. Usuelli and G. Celentano, J. Org. Chem., 2006, 71, 2064 CrossRef CAS PubMed;
(d) C. Wei and C. J. Li, J. Am. Chem. Soc., 2002, 124, 5638 CrossRef CAS PubMed.
-
(a) R. P. Herrera and E. Marques-Lopez, Multicomponent Reactions: Concepts and Applications for Design and Synthesis, John Wiley & Sons., 2015, p. 94 Search PubMed;
(b) V. A. Peshkov, O. P. Pereshivko and E. V. Van der Eycken, Chem. Soc. Rev., 2012, 41, 3790 RSC.
- L. C. Akullian, M. L. Snapper and A. H. Hoveyda, Angew. Chem., Int. Ed., 2003, 42, 4244 CrossRef CAS PubMed.
- S. N. Afraj, C. Chen and G. H. Lee, RSC Adv., 2014, 4, 26301 RSC.
- Y. Kuninobu, Y. Inoue and K. Takai, Chem. Lett., 2006, 35, 1376 CrossRef CAS.
-
(a) D. A. Kotadia and S. S. Soni, Appl. Catal., A, 2014, 488, 231 CrossRef CAS;
(b) T. Zeng, W. W. Chen, C. M. Cirtiu, A. Moores, G. Song and C. J. Li, Green Chem., 2010, 12, 570 RSC;
(c) B. Sreedhar, A. S. Kumar and P. S. Reddy, Tetrahedron Lett., 2010, 51, 1891 CrossRef CAS;
(d) W. W. Chen, R. V. Nguyen and C. J. Li, Tetrahedron Lett., 2009, 2895 CrossRef CAS;
(e) P. Li, Y. Zhang and L. Wang, Chem.–Eur. J., 2009, 15, 2045 CrossRef CAS PubMed;
(f) R. Sharma, S. Sharma and G. Gaba, RSC Adv., 2014, 4, 49198 RSC.
-
(a) C. J. Li and C. Wei, Chem. Commun., 2002, 3, 268 RSC;
(b) E. R. Bonfield, Org. Biomol. Chem., 2007, 5, 435 RSC.
- W. W. Chen, H. P. Bi and C. J. Li, Synlett, 2010, 475 CAS.
-
(a) S. Sakaguchi, T. Kubo and Y. Ishii, Angew. Chem., Int. Ed., 2001, 40, 2534 CrossRef CAS;
(b) S. Sakaguchi, T. Mizuta, M. Furuwan, T. Kubo and Y. Ishii, Chem. Commun., 2004, 1638 RSC;
(c) C. Fischer and E. M. Carreira, Org. Lett., 2001, 3, 4319 CrossRef CAS PubMed.
-
(a) S. Samai, G. C. Nandi and M. Singh, Tetrahedron Lett., 2010, 51, 5555 CrossRef CAS;
(b) K. Namitharan and K. Pitchumani, Eur. J. Org. Chem., 2010, 411 CrossRef CAS.
- R. Manikandana, P. Anithaa, P. Viswanathamurthia and J. G. Malecki, Polyhedron, 2016, 119, 300 CrossRef.
- For some examples see:
(a) S. Cheng, N. Shang, C. Feng, S. Gao, C. Wang and Z. Wang, Catal. Commun., 2017, 89, 91 CrossRef CAS;
(b) M. Varyani, P. K. Khatri and S. L. Jain, Catal. Commun., 2016, 77, 113 CrossRef CAS;
(c) M. M. Islam, A. S. Roy and S. M. Islam, Catal. Lett., 2016, 146, 1128 CrossRef CAS;
(d) S. Kumari, A. Shekhar and D. D. Pathak, RSC Adv., 2016, 6, 15340 RSC;
(e) M. Gholinejad, F. Saadati, S. Shaybanizadeh and B. Pullithadathilc, RSC Adv., 2016, 6, 4983 RSC;
(f) M. Abdolia, H. Saeidian and A. Kakanejadifard, Synlett, 2016, 27, 2473 CrossRef;
(g) P. Li, S. Regati, H. C. Huang, H. D. Arman, B.-L. Chen and J. C.-G. Zhao, Chin. Chem. Lett., 2015, 26, 6 CrossRef CAS;
(h) B. Kodicherla, P. C. Perumgani and M. R. Mandapati, Appl. Organomet. Chem., 2014, 28, 756 CrossRef CAS;
(i) M. Abdollahi-Alibeik and A. Moaddeli, RSC Adv., 2014, 4, 39759 RSC;
(j) S. Nakamura, M. Ohara, Y. Nakamura, N. Shibata and T. Toru, Chem.–Eur. J., 2010, 16, 2360 CrossRef CAS PubMed;
(k) M. K. Patil, M. Keller, B. M. Reddy, P. Pale and J. Sommer, Eur. J. Org. Chem., 2008, 4440 CrossRef CAS;
(l) J. B. Bariwal, D. S. Ermolat’ev and E. V. Van der Eycken, Chem.–Eur. J., 2010, 16, 3281 CrossRef CAS PubMed;
(m) A. Fodor, A. Kiss, N. Debreczeni, Z. Hell and I. Gresits, Org. Biomol. Chem., 2010, 8, 4575 RSC;
(n) H. B. Chen, Y. Zhao and Y. Liao, RSC Adv., 2015, 5, 37737 RSC;
(o) B. M. Choudary, C. Sridhar, M. L. Kantam and B. Screedhar, Tetrahedron Lett., 2004, 45, 7319 CrossRef CAS;
(p) L. Shi, Y.-Q. Tu, F.-M. Zhang and C. A. Fan, Org. Lett., 2004, 6, 1001 CrossRef CAS PubMed;
(q) S. B. Park and H. Alper, Chem. Commun., 2005, 1315 RSC;
(r) H. Naeimi and M. Moradian, Appl. Catal., A, 2013, 467, 400 CrossRef CAS;
(s) M. Wang, P. Li and L. Wang, Eur. J. Org. Chem., 2008, 2255 CrossRef CAS;
(t) M. J. Aliaga, D. J. Ramón and M. Yus, Org. Biomol. Chem., 2010, 8, 43 RSC.
- For some examples see:
(a) C. Wei, Z. Li and C. J. Li, Org. Lett., 2003, 5, 4473 CrossRef CAS PubMed;
(b) P. Li, L. Wang, Y. Zhang and M. Wang, Tetrahedron Lett., 2008, 49, 6650 CrossRef CAS;
(c) Z. Li, C. Wei, L. Chen, R. S. Varma and C. J. Li, Tetrahedron Lett., 2004, 45, 2443 CrossRef CAS;
(d) K. M. Reddy, N. S. Babu, I. Suryanarayana, P. S. Sai Prasad and N. Lingaiah, Tetrahedron Lett., 2006, 47, 7563 CrossRef CAS;
(e) M. Trose, M. Dell'Acqua, T. Pedrazzini, V. Pirovano, E. Gallo, E. Rossi, A. Caselli and G. Abbiati, J. Org. Chem., 2014, 79, 7311 CrossRef CAS PubMed;
(f) Y. He, M. F. Lv and C. Cai, Dalton Trans., 2012, 1248 Search PubMed;
(g) Y. Zhao, X. Zhou, T. A. Okamura, M. Chen, Y. Lu, W. Y. Sun and J. Q. Yu, Dalton Trans., 2012, 5889 RSC;
(h) X. Zhou, Y. Lu, L. L. Zhai, Y. Zhao, Q. Liu and W. Y. Sun, RSC Adv., 2013, 3, 1732 RSC;
(i) Y. Li, X. Chan, Y. Song, L. Fang and G. Zou, Dalton Trans., 2011, 40, 2046 RSC.
- For some example see:
(a) M. Gholinejad, F. Hamed and C. Nájera, Synlett, 2016, 27, 1193 CrossRef CAS;
(b) C. Wetzel, P. C. Kunz, I. Thiel and B. Spingler, Inorg. Chem., 2011, 50, 7863 CrossRef CAS PubMed;
(c) C. Wei and C.-J. Li, J. Am. Chem. Soc., 2003, 125, 9584 CrossRef CAS PubMed;
(d) G. Villaverde, A. Corma, M. Iglesias and F. Sánchez, ACS Catal., 2012, 2, 399 CrossRef CAS;
(e) G. A. Price, A. K. Brisdon, K. R. Flower, R. G. Pritchard and P. Quayle, Tetrahedron Lett., 2014, 55, 151 CrossRef CAS;
(f) P. Oña-Burgos, I. Fernández, L. Roces, L. Torre Ferenández, S. García-Granda and F. López Ortiz, Organometallics, 2009, 28, 1739 CrossRef;
(g) L. Lili, Z. Xin, R. Shumin, Y. Ying, D. Xiaoping, G. Jinsen, X. Chunminga and H. Jingb, RSC Adv., 2014, 4, 13093 RSC;
(h) F. M. Moghaddam, S. E. Ayati, S. H. Hosseinib and A. Pourjavadi, RSC Adv., 2015, 5, 34502 RSC;
(i) J.-L. Huang, D. G. Gray and C.-J. Li, Beilstein J. Org. Chem., 2013, 9, 1388 CrossRef PubMed;
(j) V. Kar-Yan Lo, K. Ka-Yan Kung, M.-K. Wong and C.-M. Che, J. Organomet. Chem., 2009, 694, 583 CrossRef;
(k) M. Kidwai, V. Bansal, A. Kumarb and S. Mozumdarb, Green Chem., 2007, 9, 742 RSC.
-
(a) Y. Qiu, Y. Qin, Z. Ma and W. Xia, Chem. Lett., 2014, 43, 1284 CrossRef CAS;
(b) N. P. Eagalapatia, A. Rajacka and Y. L. N. Murthy, J. Mol. Catal. A: Chem., 2014, 381, 126 CrossRef;
(c) K. V. V. Satyanarayana, P. A. Ramaiah, Y. L. N. Murty, M. R. Chandra and S. V. N. Pammi, Catal. Commun., 2012, 25, 50 CrossRef CAS;
(d) C. Mukhopadhyay and S. Rana, Catal. Commun., 2009, 11, 28 CrossRef;
(e) E. Ramu, R. Varala, N. Sreelatha and S. R. Adapa, Tetrahedron Lett., 2007, 48, 7184 CrossRef CAS;
(f) M. Periasamy, P. O. Reddy, A. Edukondalu, M. Dalai, L. M. Alakonda and B. Udaykumar, Eur. J. Org. Chem., 2014, 6067 CrossRef CAS.
- D. S. Raghuvanshi and K. N. Singh, Synlett, 2011, 373 CAS.
- P. H. Li and L. Wang, Chin. J. Chem., 2005, 23, 1076 CrossRef CAS.
- D. Astruc, Nanoparticles and Catalysis, Wiley-VCH, Weinheim, 2008 Search PubMed.
- V. Polshettiwar and R. S. Varma, Green Chem., 2010, 12, 743 RSC.
- Y. Sun and Y. Xia, Science, 2002, 298, 2176 CrossRef CAS PubMed.
- S. Iravani, Green Chem., 2011, 13, 2638 RSC.
- S. A. Aromal and D. Philip, Spectrochim. Acta, Part A, 2012, 97, 1 CrossRef PubMed.
- W. Rechberger, A. Hohenau, A. Leitner, J. R. Krenn, B. Lamprecht and F. R. Aussenegg, Opt. Commun., 2003, 220, 137 CrossRef CAS.
- C. L. Nehl, H. Liao and J. H. Hafner, Nano Lett., 2006, 6, 683 CrossRef CAS PubMed.
-
(a) D. Pissuwan, T. Niidome and M. B. Cortie, J. Controlled Release, 2011, 149, 65 CrossRef CAS PubMed;
(b) G. Han, P. Ghosh, M. De and V. M. Rotello, NanoBiotechnology, 2007, 3, 40 CrossRef CAS.
- E. Boisselier and D. Astruc, Chem. Soc. Rev., 2009, 38, 1759 RSC.
- D. Pissuwan, S. M. Valenzuela and M. B. Cortie, Trends Biotechnol., 2006, 24, 62 CrossRef CAS PubMed.
- K. Saha, S. S Agasti, C. Kim, X. Li and V. M. Rotello, Chem. Rev., 2012, 112, 2739 CrossRef CAS PubMed.
-
(a) G. Li and R. Jin, Nanotechnol. Rev., 2013, 5, 529 Search PubMed;
(b) Y. Chen, C. Liu, H. Abroshan, Z. Li, J. Wang, G. Li and M. Haruta, J. Catal., 2016, 340, 287 CrossRef CAS.
- S. P. Dubey, M. Lahtinen and M. Sillanpaa, Colloids Surf., A, 2010, 364, 34 CrossRef CAS.
- M. V. Sujitha and S. Kannan, Spectrochim. Acta, Part A, 2013, 102, 15 CrossRef CAS PubMed.
- T. Y. Suman, S. R. Rajasree, R. Ramkumar, C. Rajthilak and P. Perumal, Spectrochim. Acta, Part A, 2014, 118, 11 CrossRef CAS PubMed.
- C. Jayaseelan, R. Ramkumar, A. Rahuman and P. Perumal, Ind. Crops Prod., 2013, 45, 423 CrossRef CAS.
- V. Mozaffarian, Latin, English, Persian, Farhang Mo'aser, 1996 Search PubMed.
- A. A. Basaran, I. Calis, C. Anklin, S. Nishibe and O. Sticher, Chim. Acta, 1988, 71, 1483 CrossRef CAS.
- G. H. Amin, Popular medicinal plants of Iran, Ministry of Health., 1991, p. 40 Search PubMed.
- M. Rabbani, S. E. Sajjadi and H. R. Zarei, J. Ethnopharmacol., 2003, 89, 271 CrossRef CAS PubMed.
-
(a) H. Veisi, S. Taheri and S. Hemmati, Green Chem., 2016, 18, 6337 RSC;
(b) F. Bonyasi, M. Hekmati and H. Veisi, J. Colloid Interface Sci., 2017, 496, 177 CrossRef CAS PubMed;
(c) E. Farzad and H. Veisi, J. Ind. Eng. Chem., 2018, 60, 114–124 CrossRef CAS;
(d) H. Veisi, S. Najafi and S. Hemmati, Int. J. Biol. Macromol., 2018, 113, 186–194 CrossRef CAS PubMed;
(e) H. Veisi, A. Sedrpoushan and S. Hemmati, Appl. Organomet. Chem., 2015, 29, 825 CrossRef CAS;
(f) M. Pirhayati, H. Veisi and A. Kakanejadifard, RSC Adv., 2016, 6, 27252 RSC;
(g) H. Veisi, S. Azizi and P. Mohamadi, J. Cleaner Prod., 2018, 170, 1536–1543 CrossRef CAS;
(h) F. Heidari, M. Hekmati and H. Veisi, J. Colloid Interface Sci., 2017, 501, 175–184 CrossRef CAS PubMed;
(i) H. Veisi, S. A. Mirshokraie and H. Ahmadian, Int. J. Biol. Macromol., 2018, 108, 419–425 CrossRef CAS PubMed;
(j) S. Lebaschi, M. Hekmati and H. Veisi, J. Colloid Interface Sci., 2017, 485, 223 CrossRef CAS PubMed;
(k) H. Veisi, M. Pirhayati, A. Kakanejadifard, P. Mohammadi, M. R. Abdi, J. Gholami and S. Hemmati, ChemistrySelect, 2018, 3, 1820–1826 CrossRef CAS;
(l) H. Veisi, M. Ghadermazi and A. Naderi, Appl. Organomet. Chem., 2016, 30, 341 CrossRef CAS.
-
(a) H. Veisi, A. Rashtiani and andV. Barjasteh, Appl. Organomet. Chem., 2016, 30, 231 CrossRef CAS;
(b) H. Veisi, S. Hemmati, H. Shirvani and H. Veisi, Appl. Organomet. Chem., 2016, 30, 387 CrossRef CAS.
- M. Nasrollahzadeh and S. M. Sajadi, RSC Adv., 2015, 5, 46240 RSC.
- M. Nasrollahzadeh, S. M. Sajadi and M. Maham, RSC Adv., 2015, 5, 40628 RSC.
- V. Kumar, S. Mohan, D. K. Singh, D. K. Verma, V. K. Singh and S. H. Hasan, Mater. Sci. Eng., C, 2017, 71, 1004 CrossRef CAS PubMed.
- H. J. D. Dorman and S. G Deans, J. Appl. Microbiol., 2000, 88, 308 CrossRef CAS PubMed.
- A. Shtayeh, Z. Yaniv and J. Mahajna, J. Ethnopharmacol., 2000, 73, 221 CrossRef.
- G. Tumen, N. Ermin, T. Ozek, M. Kurkcuoglu and K. H. C Baser, J. Essent. Oil Res., 1994, 6, 463 CrossRef CAS.
- X. Xiong, H. Chen and R. Zhu, J. Catal., 2014, 35, 2006 CrossRef CAS.
- C. Wei, Z. Li and C. J. Li, Org. Lett., 2003, 5, 4473 CrossRef CAS PubMed.
- M. Srinivasa, P. Srinivasua, S. K. Bhargava and M. Lakshmi Kantam, Catal. Today, 2013, 208, 66 CrossRef.
- B. Karimi, M. Gholinejad and M. Khorasani, Chem. Commun., 2012, 48, 8961 RSC.
- W. Chen, R. V. Nguyen and C. J. Li, Tetrahedron Lett., 2009, 50, 2895 CrossRef CAS.
- W. Yan, R. Wang, Z. Xua, X. u Jiangke, L. Lin, Z. Shen, Y. Zhou and J. Mol, J. Mol. Catal. A: Chem., 2006, 255, 81 CrossRef CAS.
- K. D. Bhatte, D. N. Sawant, K. M. Deshmukh and B. M. Bhanage, Catal. Commun., 2011, 16, 114 CrossRef CAS.
- B. Sreedhar, A. S. Kumar and P. S. Reddy, Tetrahedron Lett., 2010, 51, 1891 CrossRef CAS.
- M. Lakshmi Kantam, S. Laha, J. Yadav and S. Bhargava, Tetrahedron Lett., 2008, 49, 3083 CrossRef.
- S. Samai, G. C. Nandi and M. S. Singh, Tetrahedron Lett., 2010, 51, 5555 CrossRef CAS.
- R. Maggi, A. Bello, C. Oro, G. Sartori and L. Soldi, Tetrahedron Lett., 2008, 64, 1435 CrossRef CAS.
- N. Zohreh, S. H. Hosseini, M. Jahani, M. S. Xaba and R. Meijboom, J. Catal., 2017, 356, 255 CrossRef CAS.
- J. R. Cammarata, R. Rivera, F. Fuentes, Y. Otero, E. Ocando-Mavárez, A. Arce and J. M Garcia, Tetrahedron Lett., 2017, 58, 4078 CrossRef CAS.
- J. S. Yadav, B. V. Subba Reddy, A. V. Hara Gopal and K. S. Patil, Tetrahedron Lett., 2009, 50, 3493 CrossRef CAS.
Footnote |
† Electronic supplementary information (ESI) available. See DOI: 10.1039/c8ra06819d |
|
This journal is © The Royal Society of Chemistry 2018 |
Click here to see how this site uses Cookies. View our privacy policy here.