DOI:
10.1039/C8RA06143B
(Paper)
RSC Adv., 2018,
8, 31028-31036
Nonisothermal decomposition and safety parameters of HNIW/TNT cocrystal
Received
20th July 2018
, Accepted 17th August 2018
First published on 4th September 2018
Abstract
To explore the thermal decomposition behavior and evaluate the thermal safety of the cocrystal 2,4,6,8,10,12-hexanitrohexaazaisowurtzitane (HNIW)/2,4,6-trinitrotoluene (TNT), its thermal and kinetic behaviors were studied by differential scanning calorimetry (DSC) technique. With the help of onset temperature (Te) and maximum peak temperature (Tp) from the non-isothermal DSC curves of HNIW/TNT cocrystal at different heating rates (β), the following were calculated: the value of specific heat capacity (Cp) and the standard molar enthalpy of formation
, the apparent activation energy (EK and EO) and pre-exponential constant (AK) of thermal decomposition reaction obtained by Kissinger's method and Ozawa's method, density (ρ) and thermal conductivity (λ), the decomposition heat (Qd, as half-explosion heat), Zhang–Hu–Xie–Li's formula, Smith's equation, Friedman's formula, Bruckman–Guillet's formula, Frank-Kamenetskii's formula and Wang–Du's formulas, the values (Te0 and Tp0) of Te and Tp corresponding to β → 0, thermal explosion temperature (Tbe and Tbp), adiabatic time-to-explosion (ttiad), 50% drop height (H50) for impact sensitivity, critical temperature of hot-spot initiation (Tcr), thermal sensitivity probability density function [S(T)] vs. temperature (T) relation curves with radius of 1 m and ambient temperature of 300 K, the peak temperature corresponding to the maximum value of S(T) vs. T relation curve (TS(T)max), safety degree (SD) and critical ambient temperature (Tacr) of thermal explosion. Results show that the kinetic equation describing the exothermic decomposition reaction of HNIW/TNT cocrystal is
The following thermal safety parameters for the HNIW/TNT cocrystal are obtained: Te0 = 464.45 K; Tp0 = 477.55 K; Tbe = 472.82 K; Tbp = 485.89 K; ttiad = 4.40 s, 4.42 s, and 4.43 s for n = 0, 1, and 2, respectively; Tcr = 531.90 K; H50 = 19.46 cm; and the values of Tacr, TS(T)max, SD and PTE are 469.69 K, 470.58 K, 78.57% and 21.43% for sphere; 465.70 K, 470.58 K, 78.17% and 21.83% for infinite cylinder; and 459.39 K, 464.26 K, 77.54% and 22.46% for infinite flat.
1 Introduction
High-density energetic materials (HDEMs) show great potential use in the military field, e.g., as propellants, pyrotechnics, explosives and gas generators.1–4 Along with the fast development of the energetic materials (EMs), the demand is increasing for the insensitive EMs; specifically, it is necessary to generate high-energy EMs with low sensitivity.5,6 As is known, the conflicts between increasing chemical energy and decreasing the sensitivity are severe. Many efforts have been made to improve the power and insensitivity of EMs, and synthesis of new compounds and modification of existing materials are the primary methods used.7–9 Co-crystallization is an effective and convenient means to improve the performance of materials, and it has drawn wide attention among researchers.10,11 Co-crystallization, combining two or more neutral molecules through non-covalent interactions, could compromise the merits of the individual substance, and one of the energetic compounds has shown the ability to alter undesirable material properties, such as high sensitivity or low density.12,13 2,4,6,8,10,12-Hexanitrohexaazaisowurtzitane (HNIW) is the most powerful explosive owing to its high density (>2.0 g cm−3), good oxygen balance (−10.94%) and high detonation velocity (9400 m s−1), but its high sensitivity toward external stimuli constrains its application.14 2,4,6-Trinitrotoluene (TNT) is an extensively used explosive with good stability. Bolton successfully prepared the HNIW·TNT cocrystal (in a 1
:
1 stoichiometry) in 2011,15 which featured insensitivity and high energy. The nonisothermal decomposition behaviors and thermal safety parameters of EMs play vital roles in theoretical studies and engineering applications, providing significant guidance to their further applications and industrial production. In recent years, reports of the HNIW·TNT cocrystal have been concentrated on its synthesis, characterization, atomistic simulation and reactive molecular dynamics,16–22 but there is little literature reported about the thermal behaviors and thermal safety parameters of HNIW·TNT cocrystal. Before HNIW·TNT cocrystal can reach practical application, much work should be done for a more comprehensive understanding of this material. To add to these studies, herein, the nonisothermal decomposition behaviors and detailed thermal safety parameters of HNIW·TNT cocrystal are described. The HNIW·TNT cocrystal was prepared and characterized. Its non-isothermal decomposition was studied by differential scanning calorimetry (DSC), and the thermal safety parameters of HNIW·TNT cocrystal, including the self-accelerating decomposition temperature (TSADT), the critical temperature of thermal explosion (Tb) and the adiabatic time-to-explosion (ttiad), were also calculated with the experimental data from non-isothermal decomposition. Moreover, the 50% drop height of impact sensitivity (H50), the critical temperature of hot-spot initiation caused by impact (Tcr), the safety degree (SD), the critical thermal explosion ambient temperature (Tacr) and thermal explosion probability (PTE) were also obtained.
2 Experimental
2.1 Caution
General caution: title compound is a hazardous material, explosions of which may occur in certain conditions. Although we had no difficulties during the experiments and in handling the compounds, appropriate safety precautions such as the use of safety glasses, face shields and horn spoons should be taken, especially when the compounds are prepared at a large scale.
2.2 Preparation
HNIW/TNT cocrystal was prepared and purified according to the literature method.15 It was kept in a vacuum desiccator before use. The structure of HNIW/TNT cocrystal was characterized by infrared spectroscopy (IR) and elemental analysis. IR (KBr): 3111, 3024 (CH), 1599, 1339, 1348, 1259, 943, 885 (NO2), 1088, 1059 (N–N), 831, 752, 721 (skeleton ring) cm−1. Anal. calcd (%) for C13H11N15O18: C 23.47, N 31.58, H 1.67; found (%) C 23.55, N 32.01, H 1.59. High-performance liquid chromatography (HPLC; LC-20AD, Shimadzu Corporation, Japan) was used to confirm the content of HNIW and TNT in the HNIW/TNT cocrystal, with a Phenomenex C18 analytical column (5.0 μm, 150 mm × 4.6 mm) at 298.15 K column temperature and the detection wavelength of 254 nm. The results demonstrate that the mole ratio of HNIW to TNT is 1
:
1 in the above prepared HNIW/TNT cocrystal.
2.3 Differential scanning calorimetry
The DSC experiments for the title compound were performed using a Q2000 apparatus (TA, USA) under nitrogen atmosphere at a flow rate of 50 ml min−1. About 0.6 mg of sample was sealed in an aluminum pan, and the heating rates used were 2.5, 5.0, 10.0, 15.0 and 20.0 K min−1 from ambient temperature to 773.15 K. DSC curves obtained under the same conditions overlapped with each other, indicating that the reproducibility of the tests was satisfactory.
3 Results and discussion
3.1 Standard enthalpy of combustion
The standard combustion enthalpy of the title compound,
(HNIW/TNT cocrystal, s, 298.15 K) was derived from the combustion enthalpy change of the following ideal combustion reaction (1) at 298.15 K and 100 kPa, |
C13H11N15O18(s) + 15.75O2(g) → 13CO2(g) + 5.5H2O(l) + 7.5N2(g),
| (1) |
and calculated by Hess's law according to the thermochemical eqn (2), |
 | (2) |
where
,23 and
determined through a thermochemical cycle, as reported elsewhere; the result obtained is −(7012.14 ± 3.42) kJ mol−1.
3.2 Standard energy of combustion
The standard energy of combustion of the title compound ΔcU (HNIW/TNT cocrystal, s, 298.15 K) was calculated by the following eqn (4): |
 | (3) |
|
Δn = ng (products) − ng (reactants)
| (4) |
where
ng is the total amount in mole of gases present as products or as reactants, Δn = 13 + 7.5 − 15.75 = 4.75, R = 8.314 J K−1 mol−1, and T = 298.15 K. The result is −(7023.914 ± 3.42) kJ mol−1.
3.3 The nonisothermal decomposition behavior of HNIW/TNT cocrystal
A typical DSC curve at a heating rate of 5.0 K min−1 for HNIW/TNT cocrystal is shown in Fig. 1. The curve consists of one endothermic peak and one exothermic peak. The endothermic peak at 413.52 K is the phase change from solid to liquid. The exothermic peak is the decomposition reaction of HNIW/TNT cocrystal; the Tp is 490.61 K, and the Te is 479.32 K.
 |
| Fig. 1 DSC curve for HNIW/TNT cocrystal at a heating rate of 5.0 K min−1. | |
3.4 Thermal conductivity (λ)
According to the relationship between specific heat capacity at constant pressure (Cp) and specific heat capacity at constant volume (Cv) as Cp = 0.83Cv24 and substituting the values of M of 665.32 and a, b, c and d in eqn (5) into eqn (6), |
CaHbOcNd = C13H11O18N15
| (5) |
|
 | (6) |
we obtain Cp = 1.01 J g−1 K−1, which is in accordance with our experiment results, determined by a continuous Cp mode of Micro-DSC III and will be reported elsewhere.
By replacing the values of Cp of 1.01 J g−1 K−1, ρ of 1.844 g cm−3, Tm of 413.52 K and M of 665.32 into eqn (7),25
|
 | (7) |
the value of
λ of 0.168 W (m K)
−1 was obtained.
3.5 Explosion properties
By substituting the values of a, b, c and d in CaHbOcNd = C13H11O18N15, ρ = 1.844 g cm−3,
into Kamlet–Jacobs eqn (8)–(12),26 the values of D of 8.624 km s−1, P of 33.89 GPa, N of 0.0289 mol g−1, Mg of 30.34 g mol−1, and Q of 6184.71 J g−1 are obtained. |
D = 1.01(NM1/2Q1/2)1/2(1 + 1.30ρ)
| (8) |
|
 | (10) |
|
 | (11) |
|
 | (12) |
where D is detonation velocity (km s−1), P detonation pressure (GPa), N moles of gas detonation products per gram of explosive, M average molecular weight of gaseous products, Q chemical energy of detonation (J g−1), ρ density of explosives (g cm−3), and ΔfHθ standard enthalpy of formation.
By substituting the values of xN2 = 7.5, xH2O = 5, xCO2 = 6, xCO = 1, xH2 = 0.5, xO2 = 0, xC = 6, and M = 665.32 g mol−1 from reaction formula (13) and ρ = 1.884 g cm−3 into empirical nitrogen equivalent eqn (14) and (15) (ref. 24) for predicting the D and P of C–H–N–O explosives, the values of D of 8.646 km s−1 and P of 33.76 GPa are obtained.
|
 | (13) |
|
 | (14) |
where 695 and 1150 are constants; 1.00, 0.64, 1.34, 0.72, 0.18, 0.50, and 0.12 are the nitrogen equivalent coefficients of gaseous detonation products N
2, H
2O, CO
2, CO, H
2, O
2, C of the explosive.
Furthermore,
|
 | (15) |
where 1.060 and 0.619 are constants. The abovementioned calculation results show that HNIW/TNT cocrystal has an explosion performance level approaching those of HMX and RDX [
D (HMX) = 8.92 km s
−1 (
β = 1.844 g cm
−3),
P (HMX) = 35.72 GPa and
D (RDX) = 8.67 km s
−1 (
β = 1.765 g cm
−3),
P (RDX) = 32.97 GPa], obtained using Kamlet–Jacobs equations.
3.6 Analysis of kinetic data for the main exothermic decomposition reaction of HNIW/TNT cocrystal
Two isoconversional methods [eqn (16) and (17)] were used to obtain the kinetic parameters (the apparent activation energy (Ea) and pre-exponential constant (A)) of the main exothermic decomposition reaction for the title compound:
Differential method. Kissinger equation27 |
 | (16) |
Integral method. Ozawa equation28 |
 | (17) |
where α is the degree of reaction, T the absolute temperature, E the apparent activation energy, β the heating rate, R the gas constant, Tp the DSC curve peak temperature, and A the pre-exponential factor.From the original data in Table 1, EK obtained by Kissinger's method27 is determined to be 239.40 kJ mol−1. The pre-exponential constant (A) is 1023.50 s−1. The linear correlation coefficient (rK) is 0.9969. The value of Eo obtained by Ozawa's method28 is 235.45 kJ mol−1. The value of r0 is 0.9971. The value of Eeo obtained by Tei vs. βi relation is 222.08 kJ mol−1. The value of reo is 0.9946.
Table 1 Maximum peak temperature (Tp) and onset temperature (Te) of the exothermic decomposition reaction for HNIW/TNT cocrystal determined by the DSC curves at various heating rates (β)
β/(K min−1) |
2.5 |
5.0 |
10.0 |
15.0 |
20.0 |
Tp/K |
484.83 |
490.61 |
495.40 |
498.75 |
502.39 |
Te/K |
472.65 |
479.32 |
483.50 |
486.82 |
490.59 |
By substituting the experimental data, βi, Ti and αi, i = 1, 2, … n, listed in Table 2 from DSC curves at differential heating rates into eqn (18), the values of Eα for any given value of α were obtained and are shown in Fig. 2. The average value of Eα in the α range of 0.10 to 0.90 in Fig. 2 is in good agreement with the calculated values obtained by Kissinger's method and Ozawa's method. The E values calculated using eqn (18)29 are used to check the validity of activation energy by the other methods.
|
 | (18) |
where the
I(
Eα,
Tα) integral is obtained taking the Senum–Yang approximation calculation for approximation of 3
rd degree:

where
u =
E/
RT.
Table 2 Data for HNIW/TNT cocrystal determined by DSC at different heating rates and apparent activation energies (Eα) of thermal decomposition obtained by integral isoconversional non-linear (NL-INT) methoda
α/(%) |
Ti/(K) |
Eα/kJ mol−1 |
min |
2.5 |
5.0 |
10.0 |
15.0 |
20.0 |
α is the degree of reaction; Ti is the corresponding temperature at different heating rates for different α; min is the minimum value of eqn (18) for any given value of α. |
0.0 |
452.38 |
461.70 |
464.54 |
467.25 |
470.47 |
203.64 |
0.6861 |
2.5 |
465.87 |
472.91 |
478.00 |
481.57 |
484.98 |
202.94 |
0.0659 |
5.0 |
469.08 |
475.84 |
481.08 |
484.73 |
488.22 |
205.02 |
0.0518 |
7.5 |
471.12 |
477.79 |
483.11 |
486.79 |
490.32 |
205.97 |
0.0479 |
10.0 |
472.67 |
479.29 |
484.65 |
488.34 |
491.87 |
207.17 |
0.0454 |
12.5 |
473.93 |
480.52 |
485.88 |
489.57 |
493.12 |
208.40 |
0.0461 |
15.0 |
474.99 |
481.57 |
486.91 |
490.60 |
494.14 |
209.78 |
0.0464 |
17.5 |
475.91 |
482.49 |
487.79 |
491.48 |
495.01 |
211.20 |
0.0479 |
20.0 |
476.72 |
483.30 |
488.57 |
492.25 |
495.77 |
212.53 |
0.0489 |
22.5 |
477.46 |
484.03 |
489.25 |
492.93 |
496.44 |
214.05 |
0.0508 |
25.0 |
478.13 |
484.68 |
489.87 |
493.54 |
497.05 |
215.40 |
0.0522 |
27.5 |
478.74 |
485.28 |
490.44 |
494.11 |
497.62 |
216.46 |
0.0537 |
30.0 |
479.31 |
485.84 |
490.97 |
494.63 |
498.14 |
217.63 |
0.0552 |
32.5 |
479.84 |
486.35 |
491.46 |
495.13 |
498.63 |
218.55 |
0.0555 |
35.0 |
480.35 |
486.83 |
491.93 |
495.59 |
499.10 |
219.51 |
0.0563 |
37.5 |
480.82 |
487.28 |
492.38 |
496.04 |
499.56 |
220.07 |
0.0568 |
40.0 |
481.27 |
487.71 |
492.81 |
496.48 |
500.00 |
220.56 |
0.0567 |
42.5 |
481.69 |
488.12 |
493.23 |
496.91 |
500.44 |
220.68 |
0.0567 |
45.0 |
482.11 |
488.51 |
493.64 |
497.34 |
500.87 |
220.83 |
0.0556 |
47.5 |
482.50 |
488.89 |
494.04 |
497.76 |
501.30 |
220.65 |
0.0553 |
50.0 |
482.88 |
489.25 |
494.44 |
498.18 |
501.72 |
220.38 |
0.0533 |
52.5 |
483.25 |
489.62 |
494.84 |
498.59 |
502.15 |
219.98 |
0.0531 |
55.0 |
483.61 |
489.98 |
495.23 |
499.01 |
502.57 |
219.50 |
0.0520 |
57.5 |
483.97 |
490.34 |
495.63 |
499.42 |
502.99 |
219.05 |
0.0507 |
60.0 |
484.32 |
490.70 |
496.02 |
499.84 |
503.42 |
218.37 |
0.0502 |
62.5 |
484.67 |
491.05 |
496.42 |
500.26 |
503.85 |
217.65 |
0.0487 |
65.0 |
485.01 |
491.42 |
496.82 |
500.68 |
504.28 |
216.91 |
0.0481 |
67.5 |
485.36 |
491.78 |
497.23 |
501.11 |
504.72 |
216.10 |
0.0466 |
70.0 |
485.71 |
492.16 |
497.65 |
501.54 |
505.17 |
215.29 |
0.0460 |
72.5 |
486.06 |
492.55 |
498.08 |
501.99 |
505.63 |
214.35 |
0.0449 |
75.0 |
486.43 |
492.95 |
498.53 |
502.45 |
506.11 |
213.45 |
0.0438 |
77.5 |
486.82 |
493.38 |
498.99 |
502.93 |
506.60 |
212.26 |
0.0432 |
80.0 |
487.24 |
493.83 |
499.48 |
503.44 |
507.12 |
211.94 |
0.0422 |
82.5 |
487.69 |
494.31 |
500.00 |
503.98 |
507.68 |
211.14 |
0.0418 |
85.0 |
488.18 |
494.84 |
500.56 |
504.56 |
508.28 |
210.41 |
0.0418 |
87.5 |
488.73 |
495.44 |
501.19 |
505.21 |
508.94 |
209.74 |
0.0412 |
90.0 |
489.38 |
496.14 |
501.92 |
505.97 |
509.70 |
209.12 |
0.0402 |
92.5 |
490.18 |
496.99 |
502.82 |
506.88 |
510.62 |
208.54 |
0.0387 |
95.0 |
491.29 |
498.14 |
504.02 |
508.10 |
511.81 |
208.50 |
0.0355 |
97.5 |
493.12 |
500.07 |
506.03 |
510.06 |
513.64 |
209.70 |
0.0271 |
100 |
502.27 |
509.77 |
519.78 |
524.06 |
523.51 |
183.13 |
0.4347 |
 |
| Fig. 2 The Eα–α curve obtained by Ozawa's method. | |
The data (β, Ti, αi, i = 1, 2, …) in the range of α = 0.10–0.90 and 47 kinetic model functions29 were input to following eqn (19)–(23) to obtain the values of E and A from a single non-isothermal DSC curve.
Ordinary-integral equation30
|
 | (19) |
Universal integral equation31
|
 | (20) |
MacCall–Tanner equation32
|
 | (21) |
Satava–Sestak equation33
|
 | (22) |
Agrawal equation34
|
 | (23) |
where
G(
α) is the integral model function, respectively,
T the temperature (K) at time
t,
α the degree of reaction, and
R the gas constant.
The kinetic parameters obtained by the logical choice method31 are presented in Table 3. Their values for E are very close to each other. The values of Ea and A obtained from a single non-isothermal DSC curve are in good agreement with the calculated values obtained by Kissinger's method and Ozawa's method. Therefore, we conclude that the reaction mechanism of the main exothermic decomposition process of the title compound is classified as G(α) = [1 − ln(1 − α)]2/3,
Substituting f(α)with
E with 235.44 kJ mol−1 and A with 1023.50 s−1 in eqn (24):
|
 | (24) |
the kinetic equation of the exothermic decomposition reaction of HNIW/TNT cocrystal may be described as:
|
 | (25) |
Table 3 Thermokinetic parameters for thermal decomposition process of HNIW/TNT cocrystal for G(α) = [1 − ln(1 − α)]2/3,
a
Method |
β/K min−1 |
E/kJ mol−1 |
lg(A0/s−1) |
r |
Q |
β is the heating rate; E is the activation energy; A0 is the pre-exponential constant; r is the linear correlation coefficient; Q is the variance. |
Ordinary-integral equation30 |
2.5 |
231.87 |
22.69 |
0.9998 |
0.0028 |
5.0 |
238.46 |
23.37 |
0.9998 |
0.0045 |
10.0 |
236.01 |
23.13 |
0.9991 |
0.0162 |
15.0 |
233.85 |
22.87 |
0.9986 |
0.0256 |
20.0 |
234.05 |
22.83 |
0.9982 |
0.0336 |
Universal integral method31 |
2.5 |
235.87 |
21.35 |
0.9998 |
0.0043 |
5.0 |
242.52 |
22.02 |
0.9998 |
0.0045 |
10.0 |
240.20 |
21.79 |
0.9992 |
0.0161 |
15.0 |
237.98 |
21.53 |
0.9987 |
0.0255 |
20.0 |
238.21 |
21.50 |
0.9983 |
0.0335 |
Maccall–Tanner32 |
2.5 |
232.93 |
22.80 |
0.9998 |
0.0008 |
5.0 |
239.68 |
23.50 |
0.9998 |
0.0008 |
10.0 |
238.68 |
23.49 |
0.9998 |
0.0008 |
15.0 |
235.19 |
23.01 |
0.9987 |
0.0048 |
20.0 |
235.45 |
22.98 |
0.9983 |
0.0063 |
Satava–Sestak equation33 |
2.5 |
228.11 |
22.34 |
0.9998 |
0.0008 |
5.0 |
234.48 |
23.00 |
0.9998 |
0.0008 |
10.0 |
237.39 |
23.26 |
0.9992 |
0.0030 |
15.0 |
230.24 |
22.54 |
0.9987 |
0.0048 |
20.0 |
230.49 |
22.51 |
0.9983 |
0.0063 |
Agrawal34 |
2.5 |
231.87 |
22.69 |
0.9998 |
0.0043 |
5.0 |
238.46 |
23.36 |
0.9998 |
0.0045 |
10.0 |
236.10 |
23.13 |
0.9991 |
0.0162 |
15.0 |
233.85 |
22.87 |
0.9986 |
0.0256 |
20.0 |
234.05 |
22.83 |
0.9982 |
0.0336 |
Mean |
|
235.44 |
22.70 |
|
|
Kissinger27 |
|
239.40 |
23.50 |
0.9969 |
|
Flynn–Wall Ozawa28 |
|
235.45 |
|
0.9971 |
|
3.7 Heat-temperature quotient for the exothermic decomposition of HNIW/TNT cocrystal
The exothermic peak in Fig. 1 is caused by the decomposition reaction. Heat-temperature quotient ΔSd of the process equals to: |
ΔSd = Qd/Tp0 = −(−2057.41 kJ mol−1)/477.55 K = 4.308 kJ mol−1 K−1
| (26) |
In eqn (26), Qd is the heat of decomposition reaction, which is defined as Qd = 0.5Q, i.e., Qd is the half-chemical energy (heat) of detonation. For HNIW/TNT cocrystal, Qd = 0.5Q = 3092.36 J g−1, and Tp0 is the peak temperature Tp corresponding to β → 0.
3.8 Self-accelerating decomposition temperature TSADT
Setting Te as the onset temperature and Tp as the peak temperature, and defining Te0 or p0 as the value of T(e0 or p0)i corresponding to β → 0 and Te0 as the self-accelerating decomposition temperature TSADT, we have: |
Te or p = Te0 or p0 + bβi + cβi2 + dβi3, i = 1, 2, …, L
| (27) |
and
Replacing the experimental data: βi, Tei, Tpi, i = 1, 2, …, 5 in Table 1 into eqn (27), the values of Te0 of 464.45 K and Tp0 of 477.55 K are obtained. The TSADT value of 464.45 K is obtained by eqn (28).
3.9 The critical temperature of thermal explosion (Tb)
The critical temperature of thermal explosion (Tb) is an important parameter in evaluating the safety and elucidating transition tendency from thermal decomposition to thermal explosion for small-scale EMs.
For HNIW/TNT cocrystal, the values of Tb obtained by the Zhang–Hu–Xie–Li equation [eqn (29)] taken from ref. 35 using the values of Te0 (464.45 K) and Tp0 (477.55 K) and the values of Eoe (222.08 kJ mol−1) and Eop (235.45 kJ mol−1) are 472.82 and 485.89 K, respectively:
|
 | (29) |
In comparison with RDX for Tbp = 454.08 K,25 the high value of Tb shows that it is difficult for the transition from thermal decomposition to thermal explosion to take place.
3.10 Thermodynamic parameters of activation reaction
The entropy of activation (ΔS≠), enthalpy of activation (ΔH≠), and Gibbs free energy of activation (ΔG≠) corresponding to T = Tp0 = 477.55 K, E = EK = 239 400 J mol−1, and A = AK = 1023.50 obtained by eqn (30)–(32) are 192.74 J mol−1 K−1, 235.43 kJ mol−1 and 143.39 kJ mol−1, respectively. The positive value of ΔG≠ indicates that the exothermic decomposition reaction for HNIW/TNT cocrystal must proceed under the heating condition. |
 | (30) |
where kB is the Boltzmann constant (1.3807 × 10−23 J K−1) and h is the Planck constant (6.626 × 10−34 J s).
3.11 The determination of the adiabatic time-to-explosion
The adiabatic time-to-explosion (ttiad, s) of energetic materials is the time of energetic material thermal decomposition transitioning to explosion under adiabatic conditions, and it is an important parameter for assessing the thermal stability and the safety of energetic materials. The estimation formulae used to calculate the adiabatic time-to-explosion (t) of energetic materials are Smith's eqn (33)–(35) taken from ref. 36. |
 | (33) |
|
 | (34) |
|
 | (35) |
where Cp is the specific heat capacity (J g−1 K−1); f(α) is the differential mechanism function; E is the apparent activation energy; A is the pre-exponential constant; Q is the heat of decomposition; R is the gas constant (8.314 J mol−1 K−1); and α is the conversion degree.
By substituting the original data of Cp = 1.01 J g−1 K−1, f(α) = (1 − α)n, E = 239 400 J mol−1, A = 1023.50 s−1, Qd = 3092.36 J g−1, and R = 8.314 J mol−1 K−1, the integral upper limit T = Tb = 472.82 K and the lower limit T0 = Te0 = 464.45 K into eqn (35), the values of t of 4.40 s for n = 0, 4.42 s for n = 1, and 4.43 s for n = 2 are obtained.
3.12 The critical temperature of hot-spot initiation (Tcr)
The interior of energetic materials (EMs) could create a local thermal point when the EMs accept energy, and the EMs will explode from thermal decomposition when the hot-spot temperature reaches the explosive temperature. The critical temperature of hot-spot initiation (Tcr) is an important parameter for evaluating the thermal safety of EMs.
By submitting the density ρ = 1.844 g cm−3 of HNIW/TNT cocrystal, specific heat capacity Cp = 1.01 J g−1 K−1, thermal conductivity λ = 16.8 × 10−4 J cm−1 s−1 K−1, heat of decomposition Qd = 3092.36 J g−1, kinetic parameters E = 239 400 J mol−1 and A = 1023.50 s−1, Troom = 293.15 K, R = 8.314 J mol−1 K−1, hot-spot critical radius a = 10−3 cm (ref. 36) and time interval t − t0 = 10−4 s (ref. 37) to Bruckman–Guillet's first-order estimation equation [eqn (36)],38,39 the value of Tcr,hotspot of 258.75 °C (531.90 K) is obtained.
|
 | (36) |
3.13 50% drop height (H50) impact sensitivity
Impact sensitivity is an important parameter for evaluating the security and reliability of EMs and can be characterized by 50% drop height (H50). Using data of λ = 16.80 × 10−4 J cm−1 s−1 K−1, ρ = 1.844 g cm−3, Qd = 3146.89 J g−1, E = 239 400 J mol−1, and A = 1023.50 s−1, the H50 of HNIW/TNT cocrystal was estimated as 19.46 cm by Friedman's formula (eqn (37)).40,41 This value shows that it has impact sensitivity level approaching those of PETN (15.6 cm) and tetryl (17.6 cm)37 |
 | (37) |
3.14 Critical thermal explosion ambient temperature Tacr, thermal sensitivity probability density function S(T), safety degree SD and thermal explosion probability PTE
To explore the heat resistance of HNIW/TNT cocrystal, the values of Tacr, S(T) vs. T relation, SD and PTE are calculated by Frank-Kamenetskii (38)42 and Wang–Du's formulas (39)–(42).43 In formulas (38)–(44), Tacr is the critical thermal explosion ambient temperature in K; E is the activation energy in J mol−1; A is the pre-exponential constant in s−1; R is the gas constant in 8.314 J K−1 mol−1; λ is the thermal conductivity in W m−1 K−1; δ is the Frank-Kamenetskii parameter (FK); δcr is the criticality of thermal explosion of exothermic system; r is characteristic measurement of reactant in m; Qd is decomposition heat in J kg−1; ρ is density in kg m−3; μT is the average value of temperature, K; σδ is the standard deviation of FK parameter; σT is the standard deviation of ambient temperature; T is surrounding temperature; S(T) is the thermal sensitivity probability density function; SD is safety degree; and PTE is thermal explosion probability. |
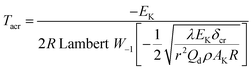 | (38) |
|
 | (39) |
where: |
 | (40) |
|
 | (41) |
|
 | (42) |
and σδ is the standard derivation of the Frank-Kamenetskii parameter, σT is the standard derivation of the measured surrounding temperature, and μT is the mean value of T.
SD was obtained by eqn (43):
|
 | (43) |
The thermal explosion probability (PTE) is expressed as eqn (44):
By substituting the values of r = 1 m, Qd = 3.09236 × 106 J kg−1, E = 239 400 J mol−1, A = 1023.50 s−1, ρ = 1.844 × 103 kg m−3, λ = 0.168 W m−1 K−1, ambient temperature Ta = 400 K, σδ = 10 K into eqn (39)–(42), the S(T) vs. T relation curves for infinite flat, infinite cylindrical and spheroid HNIW/TNT cocrystal (Fig. 3) and thermal sensitivity of the HNIW/TNT cocrystal (Table 4) are obtained, showing that the accelerating tendency from adiabatic decomposition to explosion of large-scale HNIW/TNT cocrystal and the thermal safety of HNIW/TNT cocrystal with different shapes decreases in the order: sphere > infinite cylinder > infinite flat.
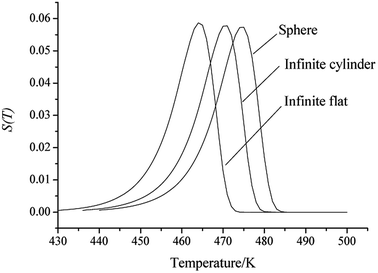 |
| Fig. 3 The S(T) vs. T relation curves for infinite flat like, infinite cylindrical and spherical HNIW/TNT cocrystal. | |
Table 4 Results for the determination of thermal sensitivity for the HNIW/TNT cocrystala
Shape types of reactant |
W/K2 |
Tarc/K |
σT/K |
TS(T)max/K |
S(X)TP |
SD/% |
PTE/% |
1 is the sphere; 2 is the infinite cylinder; 3 is the infinite flat. |
1 |
3.091 × 1032 |
469.69 |
4.19 |
470.58 |
0.0581 |
78.57 |
21.43 |
2 |
3.091 × 1032 |
465.70 |
2.57 |
470.58 |
0.0581 |
78.17 |
21.83 |
3 |
3.091 × 1032 |
459.39 |
1.16 |
464.26 |
0.0581 |
77.54 |
22.46 |
4 Conclusions
[1] The thermodynamic data describing the energetic characteristics of HNIW/TNT cocrystal are presented: (a)
(b) ΔcU (HNIW/TNT cocrystal, s, 298.15 K) = −(7023.91 ± 3.40) kJ mol−1; (c) chemical energy of detonation Q = 6184.71 J g−1.
[2] The nonisothermal thermal safety data used to evaluate the heat resistance and the transition resistance from thermal decomposition to thermal explosion of small-scale HNIW/TNT cocrystal and faster select samples are presented: Te0 = 464.45 K, Tp0 = 477.55 K, Tbe = 472.82 K, Tbp = 485.89 K, ttiad = 4.40 s for n = 0, ttiad = 4.42 s for n = 1, ttiad = 4.43 s for n = 2, Tcr = 531.90 K, H50 = 19.46 cm.
[3] The exothermic decomposition reaction of HNIW/TNT cocrystal can be described by the kinetic equation: 
[4] HNIW/TNT cocrystal is sensitive to impact; the value of H50 is 19.46 cm, which is better than PETN (H50 = 15.6 cm) and tetryl (H50 = 17.6 cm).
[5] The values of Tacr, TS(T)max, SD and PTE are 469.69 K, 470.58 K, 78.57% and 21.43% for sphere; 465.70 K, 470.58 K, 78.17% and 21.83% for infinite cylinder; and 459.39 K, 464.26 K, 77.54% and 22.46% for infinite flat. The thermal safety of large-scale HNIW/TNT cocrystal with different shapes decreases in the order: sphere > infinite cylinder > infinite flat.
[6] HNIW/TNT cocrystal has a high standard molar enthalpy of formation, high standard combustion energy, and high chemical energy (heat) of detonation, better thermal safety, an explosion performance level approaching those of HMX and RDX, and impact sensitivity level approaching those of PETN and tetryl and can be used as a main ingredient of composite explosive.
Conflicts of interest
There are no conflicts to declare.
Acknowledgements
We gratefully acknowledge the financial support from the National Natural Science Foundation of China (Grant No. 21673182, and 21703168).
References
- Q. H. Zhang and J. N. M. Shreeve, Angew. Chem., Int. Ed., 2014, 53(10), 2540–2542 CrossRef PubMed.
- S. B. Kim, K. J. Kim, M. H. Cho, J. H. Kim, K. T. Kim and S. H. Kim, ACS Appl. Mater. Interfaces, 2016, 8(14), 9405–9412 CrossRef PubMed.
- Q. H. Lin, Y. C. Li, C. Qi, W. Liu, Y. Wang and S. P. Pang, J. Mater. Chem. A, 2013, 1(23), 6776–6785 RSC.
- X. X. Zhao, S. H. Li, Y. Wang, Y. C. Li, F. Q. Zhao and S. P. P ang, J. Mater. Chem. A, 2016, 4(15), 5495–5504 RSC.
- C. He, J. Zhang, D. A. Parrish and J. N. M. Shreeve, J. Mater. Chem. A, 2013, 1(8), 2863–2868 RSC.
- V. Thottempudi, J. H. Zhang, C. L. He and J. N. M. Shreeve, RSC Adv., 2014, 4(92), 50361–50364 RSC.
- Y. R. Yao, X. Chen, Y. P. Hu, B. Yan, Y. L. Guan, J. R. Song and H. X. Ma, J. Chem. Thermodyn., 2017, 104, 67–72 CrossRef.
- C. An, J. Wang, W. Xu and F. Li, Propellants, Explos., Pyrotech., 2010, 35(4), 365–372 CrossRef.
- X. Wang, K. Xu, Q. Sun, B. Wang, C. Zhou and F. Zhao, Propellants, Explos., Pyrotech., 2015, 40(1), 9–12 CrossRef.
- F. Laraochoa and G. Espinosapérez, Supramol. Chem., 2007, 19(8), 553–557 CrossRef.
- C. Hong, Y. Xie, Y. Yao, G. Li, X. Yuan and H. Shen, Pharm. Res., 2015, 32(1), 47–60 CrossRef PubMed.
- J. C. Bennion, Z. R. Siddiqi and A. J. Matzger, Chem. Commun., 2017, 53(45), 6065–6068 RSC.
- J. Tao, B. Jin, S. Chu, R. Peng, Y. Shang and B. Tan, RSC Adv., 2018, 8(4), 1784–1790 RSC.
- Y. Wang, Z. Yang, H. Li, X. Zhou, Q. Zhang, J. Wang and Y. Liu, Propellants, Explos., Pyrotech., 2014, 39(4), 590–596 CrossRef.
- O. Bolton and A. J. Matzger, Angew. Chem., 2011, 123, 9122–9125 CrossRef.
- H. Lin, J. F. Chen, S. G. Zhu, H. Z. Li and Y. Huang, J. Energ. Mater., 2016, 35(1), 95–108 CrossRef.
- H. Liu, Z. Yang and Y. H. He, Chin. J. Explos. Propellants, 2017, 40(1), 14–20 Search PubMed.
- Z. W. Yang, H. Huang, H. Z. Li, X. Q. Zhou, J. S. Li and F. D. Nie, Chin. J. Explos. Propellants, 2012, 20(2), 256–257 Search PubMed.
- H. Li, Y. Shu, S. Gao, L. Chen, Q. Ma and X. Ju, J. Mol. Model., 2013, 19(11), 4909–4917 CrossRef PubMed.
- C. L. Wu, S. H. Zhang, R. J. Gou, F. D. Ren, G. Han and S. F. Zhu, Comput. Theor. Chem., 2018, 1127, 22–30 CrossRef.
- D. Guo, Q. An, S. V. Zybin, W. A. Goddard III, F. L. Huang and B. Tang, J. Mater. Chem. A, 2015, 3(10), 5409–5419 RSC.
- D. Z. Guo, Q. An, W. A. Goddard III, S. V. Zybin and F. L. Huang, J. Phys. Chem. C, 2014, 118(51), 30202–30208 CrossRef.
- J. D. Cox, D. D. Wagman and V. A. Medvedev, CODATA Key Values for Thermodynamics, Hemisphere Pub. Corp., 1989 Search PubMed.
- R. Z. Hu, E. G. Yao, H. X. Ma, H. Zhang, H. X. Gao, L. Han, F. Q. Zhao, Y. Luo and H. A. Zhao, Chin. J. Energ. Mater., 2015, 23(12), 1243–1244 Search PubMed.
- R. Z. Hu, F. Q. Zhao, H. X. Gao, H. X. Ma, H. Zhang, K. Z. Xu, H. A. Zhao and E. G. Yao, Acta Phys.–Chim. Sin., 2013, 29(10), 2071–2078 Search PubMed.
- M. J. Kamlet and S. J. Jacobs, J. Chem. Phys., 1968, 48(1), 23–35 CrossRef.
- H. E. Kissinger, Anal. Chem., 1957, 29(11), 1702–1706 CrossRef.
- T. Ozawa, Bull. Chem. Soc. Jpn., 1965, 38(11), 1881–1886 CrossRef.
- R. Z. Hu, S. L. Gao, F. Q. Zhao, Q. Z. Shi, T. L. Zhang and J. J. Zhang, Thermal Analysis Kinetics, Science Press, Beijing, 2008 Search PubMed.
- R. Z. Hu, and Q. Z. Shi, Thermal Analysis Kinetics, Science Press, Beijing, 2001 Search PubMed.
- R. Z. Hu, Z. Q. Yang and Y. J. Liang, Thermochim. Acta, 1988, 123(88), 135–151 Search PubMed.
- J. R. Maccallum and J. Tanner, Eur. Polym. J., 1970, 6(6), 907–917 CrossRef.
- F. Škvára and J. Šesták, J. Therm. Anal., 1975, 8(3), 477–489 CrossRef.
- R. K. Agrawal, J. Therm. Anal., 1987, 32(1), 149–156 CrossRef.
- T. L. Zhang, R. Z. Hu, Y. Xie and F. P. Li, Thermochim. Acta, 1994, 244(244), 171–176 Search PubMed.
- L. C. Smith, Thermochim. Acta, 1975, 13(1), 1–6 CrossRef.
- R. Z. Hu, F. Q. Zhao, H. X. Gao and J. R. Song, Fundamentals and Application of Calorimetry, Science Press, Beijing, 2010 Search PubMed.
- H. J. Bruckman Jr and J. E. Guillet, Can. J. Chem., 1968, 46(20), 3221–3228 CrossRef.
- M. H. Friedman, Trans. Faraday Soc., 1963, 59, 1865–1873 RSC.
- M. H. Friedman, Symp. Combust., 1963, 9(1), 294–302 CrossRef.
- R. Z. Hu, F. Q. Zhao, H. X. Gao, H. Zhao, H. A. Zhao, X. J. Wang, X. L. Zhang, F. Yu and H. X. Ma, Chin. J. Energ. Mater., 2009, 17(3), 252–254 Search PubMed.
- D. A. Frank-Kamenetskii, J. Phys. Chem., 1939, 13(6), 738–755 Search PubMed.
- W. Peng and Z. M. Du, Chin. J. Energ. Mater., 2007, 15(6), 633–636 Search PubMed.
|
This journal is © The Royal Society of Chemistry 2018 |
Click here to see how this site uses Cookies. View our privacy policy here.