DOI:
10.1039/C8RA05165H
(Paper)
RSC Adv., 2018,
8, 27935-27945
Development of a high-throughput and sensitive assay of fusion genes in lung cancer by array-based MALDI-TOFMS†
Received
16th June 2018
, Accepted 7th July 2018
First published on 6th August 2018
Abstract
ALK (anaplastic lymphoma kinase gene), ROS1 (ros proto-oncogene 1) and RET (ret proto-oncogene) fusions are oncogenic drivers in non-small cell lung cancer (NSCLC). Methods like fluorescence in situ hybridization (FISH) and immunohistochemistry (IHC) are highly sensitive but subjectively analyzed, labor intensive, expensive and unsuitable for multiple fusion gene screening. This study aimed to establish a high-throughput, sensitive and cost-effective screening method (array-based matrix-assisted laser desorption/ionization time-of-flight mass spectrometry, array-based MALDI-TOFMS) for ALK, ROS1 and RET fusion detection. This method was established with three fusion gene positive cell lines (H2228, ALK positive; HCC78, ROS1 positive; LC-2/AD, RET positive) and negative samples. Then, 34 clinical samples were selected and detected by Sanger sequencing, next generation sequencing (NGS) and array-based MALDI-TOFMS. The results were compared and analyzed and Sanger sequencing was considered the standard. 7 cases showed ALK fusions, 1 case showed ROS1 fusions, no case showed RET fusions and 4 cases were both ALK and ROS1 fusions. Results showed that array-based MALDI-TOFMS was 100% concordant with Sanger sequencing and NGS 82.3%. In this study, we reported the utility of array-based MALDI-TOFMS in the assessment of ALK, ROS1 and RET fusions in routine lung biopsies of FFPE and fresh tissue specimens. Besides, this method may also be applied to the diagnosis, monitoring and prognosis of illness.
1. Introduction
Lung cancer is the leading cause of cancer-related mortality worldwide,1,2 with non-small cell lung cancer accounting for 85% of all lung cancer cases, and its prognosis remains very poor.3,4
The anaplastic lymphoma kinase gene (ALK) encodes a receptor tyrosine kinase that is aberrant in a variety of malignancies. Approximately 3–7% of NSCLC patients harbor ALK fusions and they are more commonly found in light smokers (<10 pack years) and/or never-smokers.5,6 DNA rearrangement leads to expression of a constitutively active protein kinase. Tumors harboring ALK rearrangements rely specifically on the chimeric oncoprotein for progression and are sensitive to ALK inhibitors, such as crizotinib, a drug approved by US Food and Drug Administration for the treatment of ALK fusion-positive non-small cell lung cancer (NSCLC).5,7
The ROS proto-oncogene 1, receptor tyrosine kinase (ROS1) is a member of the insulin receptor family. Approximately 2% of lung tumors harbor ROS1 fusions and, like ALK fusions, ROS1 fusions are more commonly found in light smokers (<10 pack years) and/or never-smokers.8 In an expansion cohort of a phase I study, 50 patients with ROS1-positive NSCLC demonstrated a 72% response rate and 19.2 month median progression-free survival interval when treated with crizotinib.9
The ret proto-oncogene (RET) gene, located on chromosome 10, encodes a receptor tyrosine kinase belonging to the RET family. Several recent cancer genome sequencing studies identified RET fusions in 1–2% of NSCLC, most frequently involving kinesin family member 5B (KIF5B) and coiled-coil domain containing 6 (CCDC6) as fusion partners.10 In addition, cells with RET fusions may be sensitive to multitargeted kinase inhibitors such as sunitinib, sorafenib and vandetanib.11
Currently, the gold standard method for gene fusion detection in lung cancer is fluorescence in situ hybridization (FISH).12 However, this technique is expensive, labor intensive and requires assessment by a pathologist meaning that the results are subjective.13 Other methods like immunohistochemistry (IHC) and reverse transcription polymerase chain reaction (RT-PCR) also have their disadvantages, like the false positive rate of RT-PCR14 and the low and partial expression of ALK oncoproteins in IHC.15
NanoString technology is a promising new method for detecting fusions. Adriane and co-workers reported the usefulness of NanoString in the assessment of ALK fusions in 43 lung biopsies of FFPE specimens, which were validated previously by FISH/IHC.16 However, a larger number of samples still need to be assessed for this to be validated.
Consequently, a high-throughput, sensitive and cost-effective method that detects and evaluates ALK, ROS1 and RET fusion gene is urgently needed. MALDI-TOF was widely applied in the detection of protein and nucleic acid. Based on the conservation protein peak, this method can distinguish different species of microorganism.17 David and his co-workers made a proof of principle of their MALDI-TOFMS approach in the clinical setting using recently isolated Fusarium strains demonstrated its validity.18 Joyner utilized MALDI-TOFMS to monitor the kinetics and products of RNA cleavage and proved the method is a rapid, accurate, highly-detailed and semi-quantitative analysis of RNA cleavage.19 In this study, we combined a transcriptomic platform to handle RNA extracted from samples and array to perform high-throughput. Then we validated this method with three fusion gene positive cell lines and negative samples and tested this method with 34 formalin-fixed paraffin-embedded (FFPE) and fresh tissue samples from Chinese population and compared the results with Sanger sequencing and NGS.
2. Experimental
2.1 Reagents and instruments
The following materials were used in this study: lung cancer ALK/ROS1/RET gene Fusion Detection Kit (Guangzhou Darui Biotechnology Co. Ltd, Guangzhou, China), Recover All™ Total Nucleic Acid Isolation Kit (Thermo, MA, USA), Qiagen RNeasy Mini kit (Qiagen, Valencia, Germany), Invitrogen SuperScript® VILO cDNA synthesis kit (Thermo, MA, USA), H2O (Sigma-Aldrich, USA), Qubit 3.0™ fluorimeter (Thermo, MA, USA). Veriti Dx Thermal 96 well Cycler (Life Technologies, New York, USA), time of flight mass spectrometry detection system (DR MassARRAY) (Guangzhou Darui Biotechnology Co. Ltd, Guangzhou, China). All processes were followed by the manufacturer recommendations (Fig. 1).
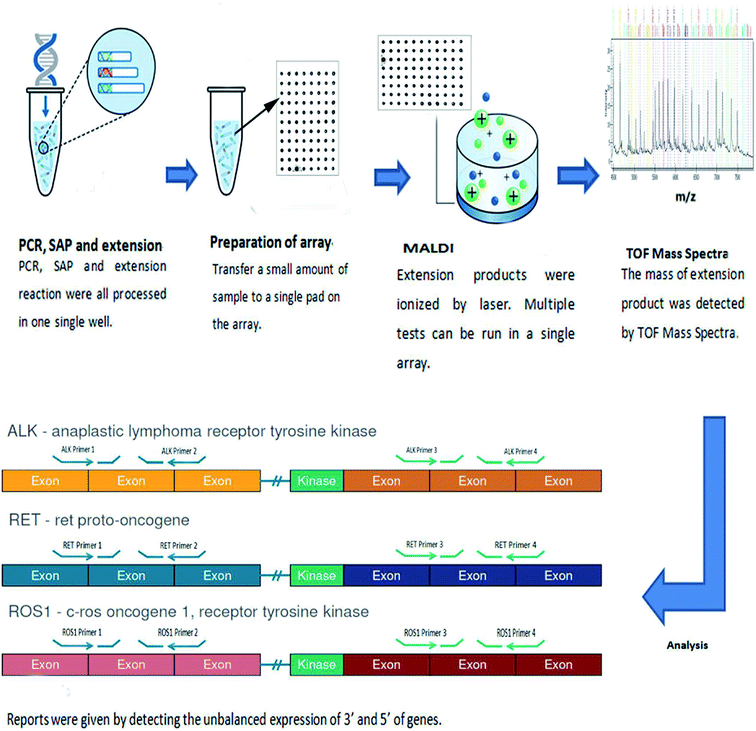 |
| Fig. 1 Workflow of array-based MALDI-TOFMS. RNA was extracted from samples and converted to cDNA, then PCR, SAP and extension were performed, extension primers were extended one base and ionized by laser. The mass of extended primers was detected by MALDI-TOFMS. By detecting and comparing the expression of 3′ and 5′ of fusion gene, a Z score would be given to evaluate fusion. | |
2.2 Preparation of samples
2.2.1 Samples. Three cell lines obtained from Cobioer Biotechnology Company (H2228, ALK positive20; HCC78, ROS1 positive21; LC-2/AD, RET positive21) cultured in 37 °C, 5% CO2 for two days and negative samples that had been previously tested by Sanger sequencing. A total of 34 surgically resected NSCLC samples which were not randomly collected but partly validated by FISH previously and 128 negative samples form DaAn Gene Co. Ltd. of Sun Yat-sen University were collected and maintained in −20 °C.
2.2.2 Ethical approval. Informed consent was obtained from each patient and the study was conducted in accordance with the Declaration of Helsinki and was approved by the Local Ethics Committee of Southern Medical University.
2.3 Establishment of array-based MALDI-TOFMS
2.3.1 RNA extraction. For FFPE samples, 2 to 10 sections (7-μm thickness) were used to isolate RNA and 30 mg for fresh tissue samples, and all of the samples used Recover All™ Total Nucleic Acid Isolation Kit. For the cell lines total RNA was isolated using the Qiagen RNeasy Mini kit. RNA concentration was assessed with Qubit.
2.3.2 Conversion of cDNA. Total RNA was converted to cDNA using an Invitrogen SuperScript® VILO cDNA synthesis kit with random hexamer priming according to manufacturer's recommendations and RNA was maintained in −80 °C.
2.3.3 Array-based MALDI-TOFMS. Multiplex screening of the samples was performed using the array-based MALDI-TOFMS LungFusion panel. The 16 extension primers were designed as listed in Table 1. Each extension primer was companied with 250 copies competitive fragments, and they were designed to evaluate the amount of fusion gene expression. By comparing the expression quantity of 3′ and 5′ section of fusion genes, the status was determined. The primers designed for EML4 were to ensure the samples obtained from lung tissues and the primers designed for GAPDH were to evaluate the quantity of gDNA.
Table 1 Number and position of extension primersa
Primers for 3′ and 5′ fusion genes were to ensure fusion types. Primers for EML4 were to ensure the samples resource and the primers designed for GAPDH were to evaluate gDNA quantity. |
Primer position |
ALK |
ROS1 |
RET |
EML4 |
GAPDH |
3′ |
5′ |
3′ |
5′ |
3′ |
5′ |
Primer number |
2 |
3 |
2 |
2 |
2 |
2 |
2 |
2 |
This method contained 5 steps, PCR, Shrimp alkaline phosphatase reaction (SAP), extension reaction, preparation of array and analysis. The thermocycling cocktail and condition are listed at Tables S1–S3.†
Array was maintained at room temperature. After liquid handling, 40 μL resin was added into extension product to adsorb saline ions, then it was sealed and spotted six dots a time on the array by dispenser. And 15 nL extension product was spotted on the array. While finishing spotting, array was sent into MALDI-TOFMS. The intensity of laser is 500 and the whole process was maintained at 4 °C.
The results were analyzed by Type 4.0, the report was given by comparing the unbalanced expression of 3′ and 5′ of genes.
2.3.4 Sanger sequencing. For the fusion gene variants of ALK, ROS1 and RET fusions, PCR primers were designed using Primer Premier 5.0 (http://www.bio-soft.net). PCR was performed by Veriti Dx Thermal 96 well Cycler. cDNA was converted from RNA, then cDNA was quantified using Qubit 3.0. The thermocycling cocktail and conditions were as follows. The sequencing process was followed to Sanger Sequencing Protocol and thermocycling cocktail and conditions are listed at Tables S4 and S5.† After that, we analyzed the results with Chromas V1.62 (http://www.sangon.com).
2.3.5 Next-generation sequencing. We used the ThermoFisher NGS Fusion Assay for fusion gene sequencing including AmpliSeq assay technology and Ion Torrent sequencing for the targeted sequencing of known and de novo fusion junctions for ALK, ROS1 and RET. The concentration of RNA was evaluated by Qubit followed by reverse transcription by multiplex PCR using a single primer pool. Briefly, libraries were prepared and barcoded sequencing adapters ligated to the resulting amplicons. Libraries were pooled, amplified in emulsion PCR and sequenced on the Ion Torrent PGM instrument. Samples were batched on a single 318 PGM chip to give >150
000 reads per sample.Torrent Suite software server for alignment was used to transfer sample data and Ion Reporter software v4.4 for variant calling. After sequencing, reads are aligned and for samples with more than 34
000 mapped reads, fusions were detected when the sequence of one gene was found relocated to that of another gene at the breakpoint. A fusion was called when more than 40 fusion reads supported the breakpoint. Fusions were also inferred qualitatively from differential expression data, where expression of the 5′ and 3′ ends of a transcript were significantly imbalanced in fusion samples compared with normal samples.
2.4 Establishment of baseline
128 negative samples were collected to establish baseline. Every extend primer was along with 250 copies competitive sequences which were synthesized to evaluate the expression of fusion gene. After calculating the median and variance of 128 samples, Z scores of each fusion type were deduced by following formula and the median and variance of ALK, ROS1 and RET are listed in Table 2. |
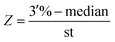 | (1) |
when Z score ≥ 3, sample was determined as fusion positive.
Table 2 Median and variance of ALK, ROS1 and RET
ALK median |
ALK variance |
ROS1 median |
ROS1 variance |
RET median |
RET variance |
0.519 |
0.126 |
0.504 |
0.092 |
0.518 |
0.105 |
2.5 Validation of clinical samples
A total of 34 clinical samples were tested by array-based MALDI-TOFMS, Sanger sequencing and NGS and the results were compared.
3. Results
3.1 Results of three cell lines and negative samples
Three cell lines (H2228, ALK positive; HCC78, ROS1 positive; LC-2/AD, RET positive) and negative samples were used as positive and negative standard to establish DR MassARRAY and all these samples were also tested by Sanger sequencing and NGS. The results are listed in Table 3.
Table 3 Summary of fusion detection results in cell lines. P = positive, N = negative
|
Array-based MALDI-TOF |
NGS |
Sanger sequencing |
ALK |
ROS1 |
RET |
ALK |
ROS1 |
RET |
ALK |
ROS1 |
ALK |
H2228 |
P |
N |
N |
EML4-ALK (E13, A20) |
N |
N |
EML4-ALK (E13, A20) |
N |
N |
HCC78 |
N |
P |
N |
N |
SLC34A2-ROS1 (S4, R34) |
N |
N |
SLC34A2-ROS1 (S4, R34) |
N |
LC-2/AD |
N |
N |
P |
N |
N |
KIF5B-RET (K15, R12) |
N |
N |
KIF5B-RET (K15, R12) |
Negative samples |
N |
N |
N |
N |
N |
N |
N |
N |
N |
As showed in Table 3, the results of array-based MALDI are totally in accordance with Sanger sequencing and NGS.
3.2 Results of 34 clinical samples
After the establishment of array-based MALDI-TOFMS, a total of 34 samples were performed fusion types detecting with three methods and the results are listed in Table 4.
Table 4 Summary of fusion detection results in clinical samples. N = negative
Sample ID |
Array-based MALDI-TOF |
Sanger sequencing |
NGS |
01 |
ALK |
EML4-ALK(E13, A20) |
EML4-ALK (E13, A20) |
02 |
ALK |
EML4-ALK (E13, A20) |
EML4-ALK (E13, A20) |
EML4-ALK (E20,A20) |
EML4-ALK (E20, A20) |
03 |
ALK&ROS1 |
EML4-ALK (E13, A20) |
EML4-ALK (E18, A20) |
SLC34A2-ROS1(S4-R34) |
SLC34A2-ROS1(S4-R32) |
SLC34A2-ROS1(S4-R32) |
04 |
ALK&ROS1 |
EML4-ALK (E13, A20) |
EML4-ALK (E13, A20) |
SLC34A2-ROS1(S4-R34) |
SLC34A2-ROS1(S4-R34) |
05 |
ALK |
EML4-ALK (E13, A20) |
EML4-ALK (E13, A20) |
06 |
ALK |
EML4-ALK (E13, A20) |
EML4-ALK (E13, A20) |
07 |
ALK |
EML4-ALK (E13, A20) |
EML4-ALK (E13, A20) |
08 |
ROS1 |
SLC34A2-ROS1(S4-R34) |
SLC34A2-ROS1(S4-R34) |
SLC34A2-ROS1(S4-R32) |
SLC34A2-ROS1(S4-R32) |
09 |
ALK |
EML4-ALK (E13, A20) |
N |
10 |
N |
N |
EML4-ALK (E13, A20) |
11 |
N |
N |
N |
12 |
N |
N |
N |
13 |
N |
N |
N |
14 |
ALK&ROS1 |
EML4-ALK (E13, A20) |
EML4-ALK (E6, A20) |
SLC34A2-ROS1(S4-R34) |
15 |
ALK |
EML4-ALK (E13, A20) |
N |
16 |
ALK&ROS1 |
EML4-ALK (E13, A20) |
N |
SLC34A2-ROS1(S4-R34) |
17 |
N |
N |
N |
18 |
N |
N |
N |
19 |
N |
N |
N |
20 |
N |
N |
N |
21 |
N |
N |
N |
22 |
N |
N |
N |
23 |
N |
N |
N |
24 |
N |
N |
N |
25 |
N |
N |
N |
26 |
N |
N |
N |
27 |
N |
N |
N |
28 |
N |
N |
N |
29 |
N |
N |
N |
30 |
N |
N |
N |
31 |
N |
N |
N |
32 |
N |
N |
N |
33 |
N |
N |
N |
34 |
N |
N |
N |
As shown in Table 4, a total of 34 samples were analyzed by array-based MALDI-TOFMS, Sanger sequencing and NGS. Of these, 12 cases contain fusions gene. 7 samples were ALK positive, 1 sample was ROS1 positive, 4 samples had both ALK and ROS1 fusions gene and no RET fusions gene were detected. Comparison of the three methods showed that the results of array-based MALDI-TOFMS were fully concordant with Sanger sequencing and 82.3% with NGS.
3.2.1 Array-based MALDI-TOFMS. Array-based MALDI-TOFMS is a promising method detecting fusion types by the expression of 3′ and 5′ of fusion genes. The positive results of ALK, ROS1 and RET fusion genes are as follows.Fig. 2–4 show the results of ALK, ROS1 and RET fusion positive tested by array-based MALDI-TOFMS. We tested 34 clinical samples of which 12 samples were positive for fusions. 7 were ALK positive, 1 was ROS1 positive and 4 were both ALK and ROS1 positive, which agreed with the results from the Sanger sequencing, but was 82.3% concordant with NGS.
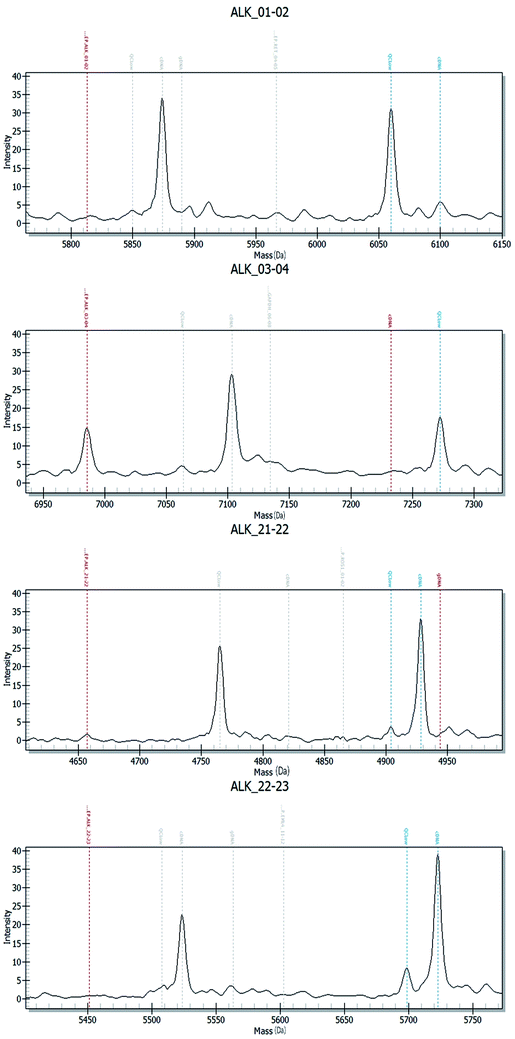 |
| Fig. 2 Array-based MALDI-TOFMS results of ALK fusions. In the mass spectra of ALK01-02, extended primers were supposed to be detected in 6100 dalton and 7232 in ALK03-04, but no cDNA or very few cDNAs were detected in ALK 01-02 and ALK 03-04, in the mass spectra of ALK 21-22, extended primers were supposed to be detected in 4929 dalton and 5722 in ALK 22-23, and large amounts of cDNA were detected in ALK 21-22 and ALK 22-23. By calculating Z score, ALK fusion was determined. | |
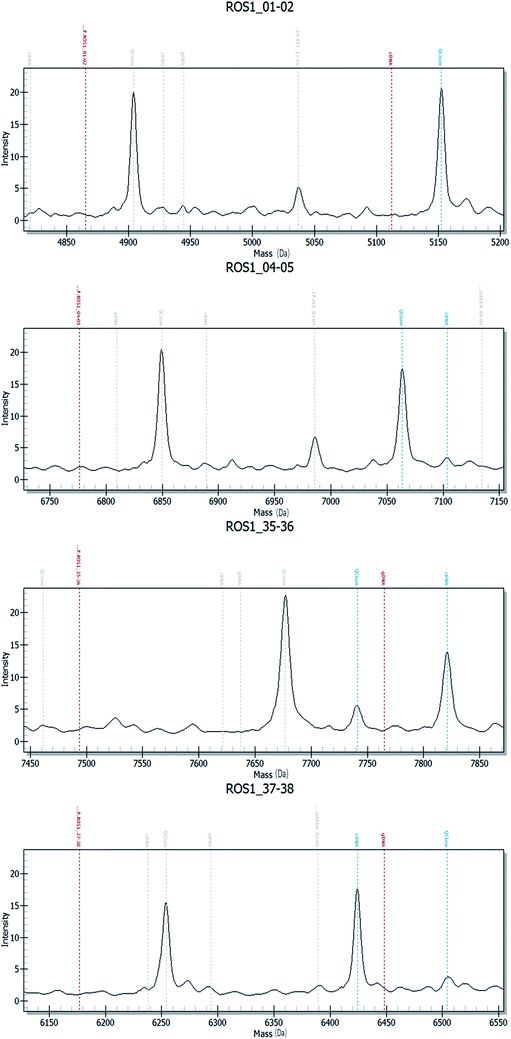 |
| Fig. 3 Array-based MALDI-TOFMS results of ROS1 fusions. In the mass spectra of ROS1 01-02, extended primers were supposed to be detected in 5112 dalton and 7103 in ROS1 04-05, but no cDNA or very few cDNAs were detected in ROS1 01-02 and ROS1 04-05, in the mass spectra of ROS1 35-36, extended primers were supposed to be detected in 7821 dalton and 6423 in ROS1 37-38, and large amounts of cDNA were detected in ROS1 35-36 and ROS1 37-38. By calculating Z score, ROS1 fusion was determined. | |
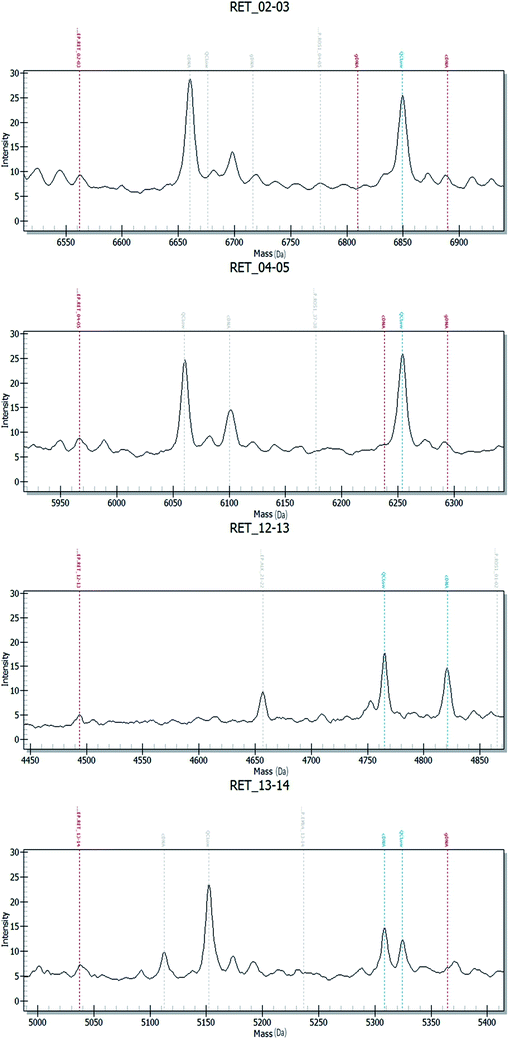 |
| Fig. 4 Array-based MALDI-TOFMS results of RET fusions in the mass spectra of RET 02-03, extended primers were supposed to be detected in 6890 dalton and 6238 in RET04-05, but no cDNA or very few cDNAs were detected in RET 02-03 and RET 04-05, in the mass spectra of RET12-13, extended primers were supposed to be detected in 4821 dalton and 5309 in RET 13-14, and large amounts of cDNAs were detected in RET 12-13 and RET 13-14. By calculating Z score, RET fusion was determined. | |
3.2.2 Sanger sequencing. Sanger sequencing is the gold standard for sequencing and it was performed as the standard of these samples, the results are as follows.Fig. 5 shows the results of ALK, ROS1 and RET fusion positive and negative tested by Sanger sequencing. A total of 34 samples were tested and gave the same results as array-based MALDI-TOFMS. Sanger sequencing provided more information than array-based MALDI-TOFMS, showing that among the 7 ALK fusion samples, six ALK fusions were an EML4-ALK (E13, A20) and 1 sample was an EML4-ALK (E13, A20) (E20, A20), and sample 8 was ROS1 (S4, R32) (S4, R34) fusion. 4 samples were both ALK and ROS1 fusions, no RET fusion was detected.
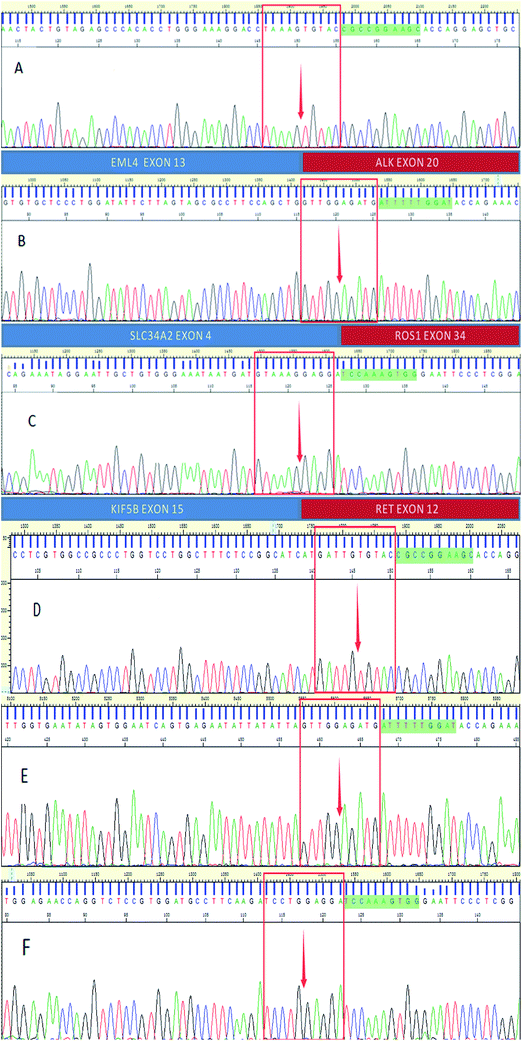 |
| Fig. 5 Results of Sanger sequencing. A is ALK fusion positive (E13, A34), the sequence before the arrow belongs to EML4, the sequence after the arrow belongs to ALK, B is ROS1 fusion positive (S4, R34), the sequence before the arrow belongs to EML4, the sequence after the arrow belongs to ROS1, C is RET fusion positive (K15, R12), the sequence before the arrow belongs to EML4, the sequence after the arrow belongs to RET, D is ALK fusion negative, E is ROS1 fusion negative, F is RET fusion negative. | |
3.2.3 Next-generation sequencing. A total of six samples gave different results between Sanger sequencing and array-based MALDI-TOFMS. This included the exon difference in sample 03, a false ALK positive in sample 10, a false ALK negative in samples 09, 15 and 16, and a false ROS1 negative in sample 14. In conclusion, the NGS method matched 82.3% with Sanger sequencing.
4. Discussion
ALK, ROS1 and RET fusions are important biomarkers for the detection of fusions in cancer,22–24 and a cost-effective, rapid and sensitive method that are capable of screening multiple fusions is required.
In the present study, we established a method to detect ALK, ROS1 and RET fusions gene in one single tube, three cell lines (H2228, ALK positive; HCC78, ROS1 positive; LC-2/AD, RET positive) and negative samples were used as standard. At the meantime, 128 negative samples were collected to establish baseline by giving median and variance, Z score would be calculated to determine fusion types. Then we collected 34 FFPE samples which were not randomly collected but partly validated by FISH previously to test this method and compared results with Sanger sequencing and NGS.
The blinded evaluation of these three methods revealed that array-based MALDI-TOFMS was fully concordant with Sanger sequencing but 82.3% concordant with NGS. However, with the limited cohort size, our statistical power for the analysis of all the three fusions was insufficient. A further assessment of these platforms with a larger number of samples is warranted.
Array-based MALDI-TOFMS requires a cDNA synthesis step, an amplification step, an annealing and single base extension step followed by MALDI-TOFMS whereby the masses of extended primers are obtained. Each extension primer was along with 250 copies competitive sequence to evaluate the 3′ and 5′ section expression of fusion gene. The Z scores of each fusion would be calculated to determine fusion type of samples.
In this study, this method provides a high-throughput, sensitive and cost-effective way to detect ALK, ROS1 and RET fusions. Array-based MALDI-TOFMS is an efficient method because it detects three fusion types in one tube, but Sanger sequencing needs at least 10 tubes. In addition, it requires no professional analysis which means the results are more objective. The whole process takes only one day to get results which is much faster compared with three or four days of NGS. However, this method is unable to identify specific fusion types and it is therefore limited for fundamental research. In this way, the method can not only be time and cost saving but also reduced the need of samples. However, we did not find RET fusion samples as this fusion only accounts for less than 1% and are hard to collect.25
The NGS fusion panel provides not only different fusion types but also the sequence information. Furthermore, it requires only 10 ng of total RNA, 10-fold less RNA than array-based MALDI-TOFMS requires. Ali and co-authors suggested that NGS could be especially useful for detecting ALK rearrangements.26 However, the disadvantages, such as expense and more effort for analysis it required are two major problems for large-scale application of NGS.27 Besides, because of the short sequence of fusion genes, NGS may not reach a precise result.
As the gold standard sequencing method, Sanger sequencing has been applied in many laboratories. Though this method did not approach a high precision in mutation detecting, it still cannot be replaced by new methods like NGS, especially when involves low-quality single-nucleotide variants and insertions or deletions <10 bp.28–30 The reasons why Sanger sequencing has not been used in large-scale application are the high cost and professional results analysis.31
Progression-free survival was significantly longer with targeted medicine than chemotherapy. For examples, patients harboring ALK positive lung cancer treated with crizotinib got a longer progression-free survival than chemotherapy.32 And crizotinib to ROS1 fusion,33 alectinib for RET fusion also approached good effect.34 Fusion gene detecting is vital for patients to get a reasonable treatment. Besides, it can also optimize the clinical resource, reduce the pain and save cost. Methods for detecting fusion gene largely applicated in clinical are FISH and IHC, however, these two methods are limited in throughput, sensitive and cost. FISH and IHC detect one fusion of samples a time, and according to the study of Yi-cheng Wu, these two methods are less sensitive.35 Besides, these two methods are expensive, about 7200yuan for FISH and 1950yuan for IHC.36 Array-based MALDI-TOF is quite a method to overcome the three problems. It detects three fusion gene of 96 samples a time and according to our study, it reached a high accordance with Sanger sequencing, lastly, it costs less than 100yuan per sample.
5. Conclusion
We established a high-throughput, sensitive and cost-effective screening method for detecting ALK, ROS1 and RET fusions which expended the application of mass spectra. Our findings validate this promising method that is a favorable alternative to FISH testing. Although the further evaluation with a larger number of samples would be carried. The developed method showed the potential in single-nucleotide variants, ctDNA detecting and may also be applied to the diagnosing, monitoring and prognosis of illness.
Conflict of interest
None.
Acknowledgements
The work was supported by the National Natural Science Foundation of China (grant number 21575058, 30901382), 2014 Guangzhou Healthy Care Synergy Innovate Major Special Project (2014) and 2014 Guangzhou Technology Project Cooperation Foundation (2014). The Special Project of International Scientific and Technological Cooperation in Guangzhou Development District (2017GH01).
References
- C. S. Dela Cruz, L. T. Tanoue and R. A. Matthay, Lung Cancer: Epidemiology, Etiology, and Prevention, Clin. Chest Med., 2011, 32(4), 605–644 CrossRef PubMed.
- R. Siegel, D. Naishadham and A. Jemal, Cancer statistics, 2013, Ca-Cancer J. Clin., 2013, 63(1), 11–30 CrossRef PubMed.
- Y. Chen, S. Han, M. Zheng, Y. Xue and W. Liu, Clinical Characteristics of 274 Non-Small Cell Lung Cancer Patients in China, Onkologie, 2013, 36(5), 3 CrossRef PubMed.
- H. Wakelee, K. Kelly, M. J. Edelman, 50 Years of Progress in the Systemic Therapy of Non-Small Cell Lung Cancer, American Society of Clinical Oncology Educational Book, 2014, vol. 34, pp. 177–189 Search PubMed.
- E. L. Kwak, Y. Bang, D. R. Camidge, A. T. Shaw, B. Solomon and R. G. Maki, et al., Anaplastic Lymphoma Kinase Inhibition in Non-Small-Cell Lung Cancer, N. Engl. J. Med., 2010, 363(18), 1693–1703 CrossRef PubMed.
- D. W. Wong, E. L. Leung, K. K. So, I. Y. Tam, A. D. Sihoe and L. Cheng, et al., TheEML4-ALK fusion gene is involved in various histologic types of lung cancers from nonsmokers with wild-typeEGFR andKRAS, Cancer-Am Cancer Soc, 2009, 115(8), 1723–1733 Search PubMed.
- D. R. Camidge, Y. Bang, E. L. Kwak, A. J. Iafrate, M. Varella-Garcia and S. B. Fox, et al., Activity and safety of crizotinib in patients with ALK-positive non-small-cell lung cancer: updated results from a phase 1 study, Lancet Oncol., 2012, 13(10), 1011–1019 CrossRef PubMed.
- K. Bergethon, A. T. Shaw, S. Ou, R. Katayama and C. M. Lovly, ROS1 Rearrangements Define a Unique Molecular Class of Lung Cancers, J. Clin. Oncol., 2012, 30(8), 863–870 CrossRef PubMed.
- A. T. Shaw, S. I. Ou, Y. Bang, D. R. Camidge, B. J. Solomon and R. Salgia, et al., Crizotinib inROS1 -Rearranged Non–Small-Cell Lung Cancer, N. Engl. J. Med., 2014, 371(21), 1963–1971 CrossRef PubMed.
- J. S. Seo, Y. S. Ju, W. C. Lee, J. Y. Shin, J. K. Lee and T. Bleazard, et al., The transcriptional landscape and mutational profile of lung adenocarcinoma, Genome Res., 2012, 22(11), 2109–2119 CrossRef PubMed.
- D. Lipson, M. Capelletti, R. Yelensky, G. Otto, A. Parker and M. Jarosz, et al., Identification of new ALK and RET gene fusions from colorectal and lung cancer biopsies, Nat. Med., 2012, 18(3), 382–384 CrossRef PubMed.
- A. T. Shaw, D. Kim, K. Nakagawa, T. Seto, L. Crinó and M. Ahn, et al., Crizotinib versus Chemotherapy in AdvancedALK -Positive Lung Cancer, N. Engl. J. Med., 2013, 368(25), 2385–2394 CrossRef PubMed.
- T. Rogers, G. M. Arnau, G. L. Ryland, S. Huang, M. E. Lira and Y. Emmanuel, et al., Multiplexed transcriptome analysis to detect ALK, ROS1 and RET rearrangements in lung cancer, Sci. Rep., 2017, 7, 42259 CrossRef PubMed.
- M. Soda, S. Takada, K. Takeuchi, Y. L. Choi and M. Enomoto, A mouse model for EML4-ALK-positive lung cancer, Proc. Natl. Acad. Sci. U. S. A., 2008, 105(50), 19893 CrossRef PubMed.
- J. M. Boland, S. Erdogan, G. Vasmatzis, P. Yang, L. Tillmans and M. Erickson-Johnson, et al., Anaplastic lymphoma kinase immunoreactivity correlates with ALK gene rearrangement and transcriptional up-regulation in non-small cell lung carcinomas, Hum. Pathol., 2009, 40(8), 1152–1158 CrossRef PubMed.
- A. F. Evangelista, M. F. Zanon, A. C. Carloni, F. E. de Paula, M. A. Morini and M. Ferreira-Neto, et al., Detection of ALK fusion transcripts in FFPE lung cancer samples by
NanoString technology, BMC Pulm. Med., 2017, 17(1), 86 CrossRef PubMed.
- S. Jadhav, M. Bhave and E. A. Palombo, Methods used for the detection and subtyping of Listeria monocytogenes, J. Microbiol. Methods, 2012, 88(3), 327–341 CrossRef PubMed.
- D. Triest, D. Stubbe, K. De Cremer, D. Piérard, A. Normand and R. Piarroux, et al., Use of Matrix-Assisted Laser Desorption Ionization–Time of Flight Mass Spectrometry for Identification of Molds of the Fusarium Genus, J. Clin. Microbiol., 2015, 53(2), 465–476 CrossRef PubMed.
- J. C. Joyner, K. D. Keuper and J. A. Cowan, Analysis of RNA cleavage by MALDI-TOF mass spectrometry, Nucleic Acids Res., 2013, 41(1), e2 CrossRef PubMed.
- N. Kanaji, S. Bandoh, T. Ishii, A. Tadokoro, N. Watanabe and T. Takahama, et al., Detection of EML4-ALK fusion genes in a few cancer cells from transbronchial cytological specimens utilizing immediate cytology during bronchoscopy, Lung Cancer, 2012, 77(2), 293–298 CrossRef PubMed.
- P. Wijesinghe, G. Bepler and A. Bollig-Fischer, A Mass Spectrometry Assay to Simultaneously Analyze ROS1 and RET Fusion Gene Expression in Non–Small-Cell Lung Cancer, J. Thorac. Oncol., 2015, 10(2), 381–386 CrossRef PubMed.
- K. Takeuchi, Y. L. Choi, Y. Togashi, M. Soda, S. Hatano and K. Inamura, et al., KIF5B-ALK, a Novel Fusion Oncokinase Identified by an Immunohistochemistry-based Diagnostic System for ALK-positive Lung Cancer, Clin. Cancer Res., 2009, 15(9), 3143–3149 CrossRef PubMed.
- K. Rikova, A. Guo and Q. Zeng, et al., Global Survey of Phosphotyrosine Signaling Identifies Oncogenic Kinases in Lung Cancer, Cell, 2007, 131(6), 1190–1203 CrossRef PubMed.
- C. Tomuschat and P. Puri, RET gene is a major risk factor for Hirschsprung's disease: a meta-analysis, Pediatr. Surg. Int., 2015, 31(8), 701–710 CrossRef PubMed.
- K. Takeuchi, M. Soda, Y. Togashi, R. Suzuki, S. Sakata and S. Hatano, et al., RET, ROS1 and ALK fusions in lung cancer, Nat. Med., 2012, 18(3), 378–381 CrossRef PubMed.
- S. M. Ali, T. Hensing, A. B. Schrock, J. Allen, E. Sanford and K. Gowen, et al., Comprehensive Genomic Profiling Identifies a Subset of Crizotinib-ResponsiveALK -Rearranged Non-Small Cell Lung Cancer Not Detected by Fluorescence In Situ Hybridization, Oncologist, 2016, 21(6), 762–770 CrossRef PubMed.
- I. Dagogo-Jack and A. T. Shaw, Screening forALK Rearrangements in Lung Cancer: Time for a New Generation of Diagnostics?, Oncologist, 2016, 21(6), 662–663 CrossRef PubMed.
- E. Vuttariello, M. Borra, C. Calise, E. Mauriello, S. Greggi and A. Vecchione, et al., A New Rapid Methodological Strategy to Assess BRCA Mutational Status, Mol. Biotechnol., 2013, 54(3), 954–960 CrossRef PubMed.
- S. P. Strom, H. Lee, K. Das, E. Vilain, S. F. Nelson and W. W. Grody, et al., Assessing the necessity of confirmatory testing for exome-sequencing results in a clinical molecular diagnostic laboratory, Genet. Med., 2014, 16(7), 510–515 CrossRef PubMed.
- D. Dacheva, R. Dodova, I. Popov, T. Goranova, A. Mitkova and V. Mitev, et al., Validation of an NGS approach for diagnostic BRCA1/BRCA2 mutation testing, Mol. Diagn. Ther., 2015, 19(2), 119–130 CrossRef PubMed.
- S. Lee, S. Zhou, T. Zhou and G. Hong, Sanger Sequencing for BRCA1 c.68_69del, BRCA1 c.5266dup and BRCA2 c.5946del Mutation Screen on Pap Smear Cytology Samples, Int. J. Mol. Sci., 2016, 17(2), 229 CrossRef PubMed.
- B. J. Solomon, T. Mok, D. Kim, Y. Wu, K. Nakagawa and T. Mekhail, et al., First-Line Crizotinib versus Chemotherapy inALK-Positive Lung Cancer, N. Engl. J. Med., 2014, 371(23), 2167–2177 CrossRef PubMed.
- A. T. Shaw, S. I. Ou, Y. Bang, D. R. Camidge, B. J. Solomon and R. Salgia, et al., Crizotinib inROS1-Rearranged Non–Small-Cell Lung Cancer, N. Engl. J. Med., 2014, 371(21), 1963–1971 CrossRef PubMed.
- T. Kodama, T. Tsukaguchi, Y. Satoh, M. Yoshida, Y. Watanabe and O. Kondoh, et al., Alectinib Shows Potent Antitumor Activity against RET-Rearranged Non-Small Cell Lung Cancer, Mol. Cancer Ther., 2014, 13(12), 2910–2918 CrossRef PubMed.
- Y. Wu, Comparison of IHC, FISH and RT-PCR Methods for Detection of ALK Rearrangements in 312 Non-Small Cell Lung Cancer Patients in Taiwan, PLoS One, 2013, 8(8), e70839 CrossRef PubMed.
- H. Jiang-jiang, L. Xian-zhong and H. Da, Economic Analysis on Anaplastic Lymphoma Kinase Related Companion Diagnosis Methods before the Targeted Therapy for Non-small Cell Lung Cancer, Chinese Health Economics, 2017, 36(1), 40–43 Search PubMed.
Footnote |
† Electronic supplementary information (ESI) available. See DOI: 10.1039/c8ra05165h |
|
This journal is © The Royal Society of Chemistry 2018 |
Click here to see how this site uses Cookies. View our privacy policy here.