DOI:
10.1039/C8RA02009D
(Paper)
RSC Adv., 2018,
8, 15444-15447
A C1-symmetric N-heterocyclic carbene catalysed oxidative spiroannulation of isatin-derived enals: highly enantioselective synthesis of spirooxindole δ-lactones†
Received
7th March 2018
, Accepted 2nd April 2018
First published on 24th April 2018
Abstract
A C1-symmetric N-heterocyclic carbene (NHC)-catalysed activation of isatin-derived enals under oxidative conditions was achieved. The in situ generated α,β-unsaturated acyl azolium species was efficiently trapped by 1,3-dicarbonyl compounds via a Michael addition/spiroannualtion cascade, delivering a series of synthetically important spirooxindole δ-lactones with up to 96% enantioselectivity.
Spirooxindoles are present in a wide variety of natural products and biologically active molecules.1 Polycyclic spirooxindole scaffold with multiple stereocenters are particularly intriguing targets in organic synthesis owing to their structural complexity and potential pharmaceutical value.2 For example, the spirooxindole δ-lactone constitutes the backbone of many natural products, such as trigolutes A–C (Fig. 1a).3 However, the catalytic asymmetric synthesis of this privileged scaffold in a highly stereocontrolled fashion is still a challenging task, and very limited protocols were established to this end.3c,4 In this regard, the development of new, practical and efficient strategy for the highly enantioselective synthesis of spirooxindole δ-lactone scaffold is still in great demand.
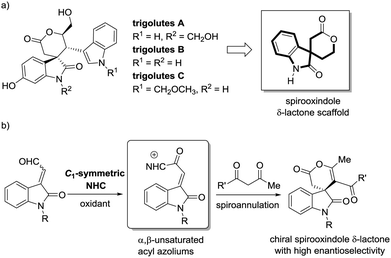 |
| Fig. 1 (a) Examples of spirooxindole-containing natural products. (b) Assembly of spirooxindole δ-lactone scaffolds via C1-symmetric NHC-catalysed spiroannulation (this work). | |
Over the past decades, the ability of chiral N-heterocyclic carbenes (NHCs)5 toward activation of aldehyde and activated carboxylic acid derivatives through formation of transient acyl anion,6 enolate,7 homoenolate8 and acyl azolium9 intermediates was well-investigated for enantioselective C–C, C–O and C–N bond formations. Within the context, the oxidative NHC catalysis strategy enabled generation of acyl azolium from aldehydes via in situ oxidation, which provided new opportunities for reaction design and selectivity control, thus remarkably extending the scope of NHC catalysis.10,11 However, despite fruitful achievements in the oxidative annulation of simple unsaturated aldehydes,12 oxidative NHC catalysed addition/cyclization of β,β-disubstituted enals, such as isatin-derived enals, was a very attractive yet challenging topic with the concomitant formation of a congested quaternary center.13 Recently, we disclosed that C1-symmetric biaryl-saturated imidazolium catalyst was a superior NHC for asymmetric oxidative annulation of α-aryl-substituted α,β-unsaturated aldehydes.14 With our ongoing interest in the development of practical methods toward asymmetric synthesis of chiral spirooxindole scaffolds,4b,15 we attempted to explore the catalytic performance of C1-symmetric NHC in oxidative activation of isatin-derived enals.16 The in situ generated isatin-derived α,β-unsaturated acyl azolium could be trapped by readily available 1,3-dicarbonyl compounds via a spiro-quaternary carbon-forming Michael addition/cyclization process, eventually delivering synthetically intriguing chiral spirooxindole δ-lactones (Fig. 1b).17
Our investigation started with the reaction of isatin-derived α,β-unsaturated aldehyde 1a (a mixture of E and Z isomer) with 1,3-dicarbonyl compound 2a under the catalysis of C1-symmetric diaryl-saturated imidazolium catalysts I–III.18 To our delight, the desired oxidative annulation product 3a and its regioisomer 3a' (6
:
1 rr) were obtained in 23% yield and with high enantioselectivity (89% ee) when catalyst I/DBU combination were employed under the oxidation of 3,3′,5,5′-tetra-tert-butyldiphenoquinone in THF (Table 1, entry 1), while catalyst II and III gave a relatively higher stereoselectivity and regioselectivity (Table 1, entries 2 and 3). As a comparison, the catalytic activity of C2-symmetric diaryl-saturated catalyst IV, triazolium catalysts V and VI was further examined, and inferior results with respect to reactivity and selectivity were observed (Table 1, entries 4–6). Then different bases including inorganic and organic bases were varied, and we found that DBU give better stereoselectivity and regioselectivity within a shorter time (Table 1, entries 7–12). Solvent screening revealed that toluene gave rise to a better yield (Table 1, entries 13–17). When the catalyst loading was reduced to 10%, the reaction yield was obviously decreased (Table 1, entry 18). For further investigation, we modulated the ratio of 1 and 2 to 1
:
1, and further improvement in yield was observed (Table 1, entry 19).
Table 1 Optimization of reaction conditions
With the optimized reaction conditions in hand, the generality of the reaction was explored (Table 2, entries 1–20). A broad range of differently substituted 1,3-dione bearing electron-donating or electron-withdrawing substituents on meta- or para-position of aromatic rings were well tolerated, whereas the 1,3-dione with substituents on ortho-position of aromatic rings gave complex products as a result of steric hindrance. Although no pronounced electronic effect was observed, we discovered that substrates with the nitro group (2e, 2n) gave lower yields and enantioselectivities (Table 2, entries 5 and 14), while substrates with the methyl group (2g, 2j) gave lower regioselectivities but higher yields (Table 2, entries 7, 8 and 10). Heteroaryl-containing substrate (2o) also worked well to give the δ-lactone 3o with excellent regioselectivity in moderate yield (52%) and high ee (83%) (Table 2, entry 15). Aliphatic 1,3-dione 2p could participate in the oxidative reaction efficiently, giving rise to the product in 58% yield and 90% ee within 10 minutes (Table 2, entry 16), while biaryl-substituted substrate 2q give the corresponding product 3t in 60% yield and 96% ee over 24 h (Table 2, entry 20). Further investigation revealed that this method was compatible with different N-substituted enals, affording the desired δ-lactone 3q-3s in moderate yield with good regioselectivities and excellent enantioselectivities (Table 2, entries 17–19).
Table 2 Scope of the reaction
The absolute configuration of the product 3a was assigned to be S by X-ray crystallographic analysis (Cu target, see ESI†).19 Based on our experimental results and previous literature reports, a proposed catalytic cycle is shown in Scheme 1. The free NHC catalyst, derived from precatalyst by in situ deprotonation, could react with isatin-derived unsaturated aldehyde 1a, giving rise to Breslow intermediate A, which could be converted to acyl azolium intermediate B through two-electron oxidation and deprotonation. Subsequent Re-face Michael attack of 2a-derived enolate to species B along with the proton transfer sequentially produced intermediates C and D. Intramolecular cyclization of D gave the product 3a and released the NHC catalyst to next catalytic cycle. Mechanistically, the formation of byproduct 3a′ arose from intramolecular annulation of intermediate D′, which was generated from Michael adduct C via undesired competing proton transfer step.20
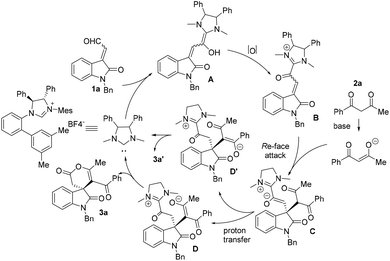 |
| Scheme 1 Proposed catalytic cycle. | |
Conclusions
In conclusion, we have developed a C1-symmetric NHC catalyzed oxidative spiroannulation of isatin-derived enals. The in situ generated isatin-derived α,β-unsaturated acyl azolium species was efficiently trapped by 1,3-dicarbonyl compounds via a Michael addition/cyclization process. A series of synthetically intriguing spirooxindole δ-lactones was readily obtained in moderate to high yields with excellent enantioselectivities under mild conditions. Further application of the C1-symmetric NHCs to novel and challenging transformations toward synthesis of synthetically or pharmaceutically valuable molecules are currently underway.
Conflicts of interest
There are no conflicts to declare.
Acknowledgements
We are grateful to the NSFC (21572087, 21632003), the Key program of Gansu province (17ZD2GC011) and the “111” program from the MOE of P. R. China for financial support.
Notes and references
- For selected reviews, see:
(a) C. Marti and E. M. Carreira, Eur. J. Org. Chem., 2003, 2209 CrossRef CAS;
(b) C. V. Galliford and K. A. Scheidt, Angew. Chem., Int. Ed., 2007, 46, 8748 CrossRef CAS PubMed;
(c) R. Dalpozzo, G. Bartoli and G. Bencivenni, Chem. Soc. Rev., 2012, 41, 7247 RSC;
(d) N. R. Ball-Jones, J. J. Badillo and A. K. Franz, Org. Biomol. Chem., 2012, 10, 5165 RSC.
- For selected examples, see:
(a) X. H. Chen, Q. Wei, S. W. Luo, H. Xiao and L. Z. Gong, J. Am. Chem. Soc., 2009, 131, 13819 CrossRef CAS PubMed;
(b) A. P. Antonchick, C. Gerding-Reimers, M. Catarinella, M. Schürmann, H. Preut, S. Ziegler, D. Rauh and H. Waldmann, Nat. Chem., 2010, 2, 735 CrossRef CAS PubMed;
(c) B. Tan, N. R. Candeias and C. F. Barbas III, Nat. Chem., 2011, 3, 473 CrossRef CAS PubMed;
(d) Y. Liu, M. Nappi, E. Arceo, S. Vera and P. Melchiorre, J. Am. Chem. Soc., 2011, 133, 15212 CrossRef CAS PubMed;
(e) G. Zhan, M. L. Shi, Q. He, W. J. Lin, Q. Ouyang, W. Du and Y. C. Chen, Angew. Chem., Int. Ed., 2016, 55, 2147 CrossRef CAS PubMed;
(f) X. Han, W. L. Chan, W. Yao, Y. Wang and Y. Lu, Angew. Chem., Int. Ed., 2016, 55, 6492 CrossRef CAS PubMed.
-
(a) Y. G. Chen, J. C. Wu, G. Y. Chen, C. R. Han and X. P. Song, Chem. Biodiversity, 2011, 8, 1958 CrossRef CAS PubMed;
(b) S. S. Ma, W. L. Mei, Z. K. Guo, S. B. Liu, Y. X. Zhao, D. L. Yang, Y. B. Zeng, B. Jiang and H. F. Dai, Org. Lett., 2013, 15, 1492 CrossRef CAS PubMed;
(c) J. Z. Huang, C. L. Zhang, Y. F. Zhu, L. L. Li, D. F. Chen, Z. Y. Han and L. Z. Gong, Chem.–Eur. J., 2015, 21, 8389 CrossRef CAS PubMed.
-
(a) D. Du, Z. Y. Hu, J. L. Jin, Y. Y. Lu, W. F. Tang, B. Wang and T. Lu, Org. Lett., 2012, 14, 1274 CrossRef CAS PubMed;
(b) S. Zhao, J. B. Lin, Y. Y. Zhao, Y. M. Liang and P. F. Xu, Org. Lett., 2014, 16, 1802 CrossRef CAS PubMed.
- For selected reviews, see:
(a) D. Enders, O. Niemeier and A. Henseler, Chem. Rev., 2007, 107, 5606 CrossRef CAS PubMed;
(b) V. Nair, S. Vellalath and B. P. Babu, Chem. Soc. Rev., 2008, 37, 2691 RSC;
(c) A. Grossmann and D. Enders, Angew. Chem., Int. Ed., 2012, 51, 314 CrossRef CAS PubMed;
(d) J. Izquierdo, G. E. Hutson, D. T. Cohen and K. A. Scheidt, Angew. Chem., Int. Ed., 2012, 51, 11686 CrossRef CAS PubMed;
(e) X. Bugaut and F. Glorius, Chem. Soc. Rev., 2012, 41, 3511 RSC;
(f) S. J. Ryan, L. Candish and D. W. Lupton, Chem. Soc. Rev., 2013, 42, 4906 RSC;
(g) M. N. Hopkinson, C. Richter, M. Schedler and F. Glorius, Nature, 2014, 510, 485 CrossRef CAS PubMed;
(h) J. Mahatthananchai and J. W. Bode, Acc. Chem. Res., 2014, 47, 696 CrossRef CAS PubMed;
(i) D. M. Flanigan, F. Romanov-Michailidis, N. A. White and T. Rovis, Chem. Rev., 2015, 115, 9307 CrossRef CAS PubMed;
(j) Y. Wang, D. Wei and W. Zhang, ChemCatChem, 2018, 10, 338 CrossRef CAS;
(k) X. Y. Chen, Q. Liu, P. Chauhan and D. Enders, Angew. Chem., Int. Ed., 2018, 57, 3862 CrossRef CAS PubMed.
-
(a) T. Ukai, S. Tanaka and S. Dokawa, J. Pharm. Soc. Jpn., 1943, 63, 296 CrossRef CAS;
(b) D. Enders, K. Breuer, J. Runsink and J. H. Teles, Helv. Chim. Acta, 1996, 79, 1899 CrossRef CAS;
(c) D. Enders and U. Kallfass, Angew. Chem., Int. Ed., 2002, 41, 1743 CrossRef CAS;
(d) M. S. Kerr, J. R. Alaniz and T. Rovis, J. Am. Chem. Soc., 2002, 124, 10298 CrossRef CAS PubMed;
(e) Y. Hachisu, J. W. Bode and K. Suzuki, J. Am. Chem. Soc., 2003, 125, 8432 CrossRef CAS PubMed;
(f) H. Y. Zhao, F. W. Foss and R. Breslow, J. Am. Chem. Soc., 2008, 130, 12590 CrossRef CAS PubMed;
(g) Q. Liu, S. Perreault and T. Rovis, J. Am. Chem. Soc., 2008, 130, 14066 CrossRef CAS PubMed;
(h) S. P. Lathrop and T. Rovis, J. Am. Chem. Soc., 2009, 131, 13628 CrossRef CAS PubMed;
(i) A. T. Biju, N. E. Wurz and F. Glorius, J. Am. Chem. Soc., 2010, 132, 5970 CrossRef CAS PubMed;
(j) T. Jousseaume, N. E. Wurz and F. Glorius, Angew. Chem., Int. Ed., 2011, 50, 1410 CrossRef CAS PubMed;
(k) X. Fang, X. Chen, H. Lv and Y. R. Chi, Angew. Chem., Int. Ed., 2011, 50, 11782 CrossRef CAS PubMed;
(l) C. A. Rose, S. Gundala, C. L. Fagan, J. F. Franz, S. J. Connon and K. Zeitler, Chem. Sci., 2012, 3, 735 RSC;
(m) M. Schedler, D. S. Wang and F. Glorius, Angew. Chem., Int. Ed., 2013, 52, 2585 CrossRef CAS PubMed.
-
(a) M. He, J. R. Struble and J. W. Bode, J. Am. Chem. Soc., 2006, 128, 8418 CrossRef CAS PubMed;
(b) M. He, G. J. Uc and J. W. Bode, J. Am. Chem. Soc., 2006, 128, 15088 CrossRef CAS PubMed;
(c) J. Kaeobamrung, M. C. Kozlowski and J. W. Bode, Proc. Natl. Acad. Sci. U. S. A., 2010, 107, 20661 CrossRef CAS PubMed;
(d) L. Hao, Y. Du, H. Lv, X. Chen, H. Jiang, Y. Shao and Y. R. Chi, Org. Lett., 2012, 14, 2154 CrossRef CAS PubMed;
(e) L. Wang, Q. Ni, M. Blgmel, T. Shu, G. Raabe and D. Enders, Chem.–Eur. J., 2015, 21, 8033 CrossRef CAS PubMed.
-
(a) C. Burstein and F. Glorius, Angew. Chem., Int. Ed., 2004, 43, 6205 CrossRef CAS PubMed;
(b) S. S. Sohn, E. L. Rosen and J. W. Bode, J. Am. Chem. Soc., 2004, 126, 14370 CrossRef CAS PubMed;
(c) C. Guo, M. Schedler, C. G. Daniliuc and F. Glorius, Angew. Chem., Int. Ed., 2014, 53, 10232 CrossRef CAS PubMed;
(d) C. Guo, B. Sahoo, C. G. Daniliuc and F. Glorius, J. Am. Chem. Soc., 2014, 136, 17402 CrossRef CAS PubMed;
(e) C. Guo, M. Fleige, D. Janssen-Müller, C. G. Daniliuc and F. Glorius, Nat. Chem., 2015, 7, 842 CrossRef CAS PubMed;
(f) L. Wang, S. Li, M. Blümel, R. Puttreddy, A. Peuronen, K. Rissanen and D. Ender, Angew. Chem., Int. Ed., 2017, 56, 8516 CrossRef CAS PubMed.
-
(a) S. J. Ryan, L. Candish and D. W. Lupton, J. Am. Chem. Soc., 2009, 131, 14176 CrossRef CAS PubMed;
(b) F. G. Sun, L. H. Sun and S. Ye, Adv. Synth. Catal., 2011, 353, 3134 CrossRef CAS;
(c) J. Cheng, Z. Huang and Y. R. Chi, Angew. Chem., Int. Ed., 2013, 52, 8592 CrossRef CAS PubMed.
- For reviews on oxidative NHC catalysis, see:
(a) C. E. I. Knappke, A. Imami and A. J. Wangelin, ChemCatChem, 2012, 4, 937 CrossRef CAS;
(b) S. D. Sarkar, A. Biswas, R. C. Samanta and A. Studer, Chem.–Eur. J., 2013, 19, 4664 CrossRef PubMed.
-
(a) J. Guin, S. De Sarkar, S. Grimme and A. Studer, Angew. Chem., Int. Ed., 2008, 47, 8727 CrossRef CAS PubMed;
(b) B. E. Maki, A. Chan, E. M. Phillips and K. A. Scheidt, Org. Lett., 2007, 9, 371 CrossRef CAS PubMed;
(c) S. D. Sarkar, S. Grimme and A. Studer, J. Am. Chem. Soc., 2010, 132, 1190 CrossRef PubMed;
(d) S. D. Sarkar and A. Studer, Org. Lett., 2010, 12, 1992 CrossRef PubMed;
(e) X. Zhao, K. E. Ruhl and T. Rovis, Angew. Chem., Int. Ed., 2012, 51, 12330 CrossRef CAS PubMed;
(f) J. Mo, X. Chen and Y. R. Chi, J. Am. Chem. Soc., 2012, 134, 8810 CrossRef CAS PubMed;
(g) E. G. Delany, C. L. Fagan, S. Gundala, A. Mari, T. Broja, K. Zeitler and S. J. Connon, Chem. Commun., 2013, 49, 6510 RSC;
(h) J. Mo, L. Shen and Y. R. Chi, Angew. Chem., Int. Ed., 2013, 52, 8588 CrossRef CAS PubMed;
(i) H. Inoue and K. Higashiura, J. Chem. Soc., Chem. Commun., 1980, 549 RSC.
- For selected examples, see:
(a) S. D. Sarkar and A. Studer, Angew. Chem., Int. Ed., 2010, 49, 9266 CrossRef PubMed;
(b) A. Biswas, S. D. Sarkar, R. Fröhlich and A. Studer, Org. Lett., 2011, 13, 4966 CrossRef CAS PubMed;
(c) Z. Q. Rong, M. Q. Jia and S. L. You, Org. Lett., 2011, 13, 4080 CrossRef CAS PubMed;
(d) Z. Q. Zhu, X. L. Zheng, N. F. Jiang, X. L. Wan and J. C. Xiao, Chem. Commun., 2011, 47, 8670 RSC;
(e) A. G. Kravina, J. Mahatthananchai and J. W. Bode, Angew. Chem., Int. Ed., 2012, 51, 9433 CrossRef CAS PubMed;
(f) S. Bera, R. C. Samanta, C. G. Daniliuc and A. Studer, Angew. Chem., Int. Ed., 2014, 53, 9622 CrossRef CAS PubMed;
(g) X. X. Wu, B. Liu, Y. X. Zhang, M. Jeret, H. L. Wang, P. C. Zheng, S. Yang, B. A. Song and Y. R. Chi, Angew. Chem., Int. Ed., 2016, 55, 12280 CrossRef CAS PubMed.
- For one report concerning trapping isatin-derived unsaturated acyl azolium by performed enamine, see:
(a) D. Xie, L. Yang, Y. Lin, Z. Zhang, D. Chen, X. Zeng and G. Zhong, Org. Lett., 2015, 17, 2318 CrossRef CAS PubMed , For a racemic
example, see:;
(b) L. L. Zhao, X. S. Li, L. L. Cao, R. Zhang, X. Q. Shi and J. Qi, Chem. Commun., 2017, 53, 5985 RSC.
- H. Lu, J. Y. Liu, C. G. Li, J. B. Lin, Y. M. Liang and P. F. Xu, Chem. Commun., 2015, 51, 4473 RSC.
-
(a) Y. Wang, H. Lu and P. F. Xu, Acc. Chem. Res., 2015, 48, 1832 CrossRef CAS PubMed;
(b) T. P. Gao, J. B. Lin, X. Q. Hu and P. F. Xu, Chem. Commun., 2014, 50, 8934 RSC;
(c) L. Tian, X. Q. Hu, Y. H. Li and P. F. Xu, Chem. Commun., 2013, 49, 7213 RSC;
(d) Y. Y. Zhao, S. Zhao, J. K. Xie, X. Q. Hu and P. F. Xu, J. Org. Chem., 2016, 81, 10532 CrossRef CAS PubMed.
-
(a) R. Liu and J. Zhang, Org. Lett., 2013, 15, 2266 CrossRef CAS PubMed;
(b) R. Liu and J. Zhang, Chem.–Eur. J., 2013, 19, 7319 CrossRef CAS PubMed;
(c) T. Mukaiyama, K. Ogata, I. Sato and Y. Hayashi, Chem.–Eur. J., 2014, 20, 13583 CrossRef CAS PubMed.
- For a racemic synthesis of this scaffold using isatin-derived 2-bromoenals, see:
(a) J. Xu, W. Zhang, Y. Liu, S. Zhu, M. Liu, X. Hua, S. Chen, T. Lu and D. Du, RSC Adv., 2016, 6, 18601 RSC , For a catalytic asymmetric synthesis using isatin-derived unsaturated acids with moderate ees, see:;
(b) W. Zhang, J. Xu, J. Cao, C. Fang, J. Zhu, T. Lu and D. Du, Tetrahedron, 2017, 73, 3249 CrossRef CAS.
-
(a) K. P. Jang, G. E. Hutson, R. C. Johnston, E. O. McCusker, P. H. Y. Cheong and K. A. Scheidt, J. Am. Chem. Soc., 2014, 136, 76 CrossRef CAS PubMed;
(b) A. Lee and K. A. Scheidt, Angew. Chem., Int. Ed., 2014, 53, 7594 CrossRef CAS PubMed.
- CCDC 1010784 (3a)..
- With regard to the mechanism, the hemiacetal formation-Coates-Claisen rearrangement pathway proposed by Bode et al. cannot be ruled out at current stage, see:
(a) J. Mahatthananchai, P. Zheng and Jeffrey W. Bode, Angew. Chem., Int. Ed., 2011, 50, 1673 CrossRef CAS PubMed;
(b) J. Mahatthananchai, J. Kaeobamrung and J. W. Bode, ACS Catal., 2012, 2, 494 CrossRef CAS PubMed;
(c) E. Lyngvi, J. W. Bode and F. Schoenebeck, Chem. Sci., 2012, 3, 2346 RSC.
Footnotes |
† Electronic supplementary information (ESI) available: CCDC 1010784. For ESI and crystallographic data in CIF or other electronic format see DOI: 10.1039/c8ra02009d |
‡ The two authors contributed equally to this work. |
|
This journal is © The Royal Society of Chemistry 2018 |
Click here to see how this site uses Cookies. View our privacy policy here.