DOI:
10.1039/C8RA00443A
(Paper)
RSC Adv., 2018,
8, 18175-18179
Anti-inflammatory and antioxidant activities of flavonoids from the flowers of Hosta plantaginea†
Received
15th January 2018
, Accepted 11th May 2018
First published on 17th May 2018
Abstract
Hosta plantaginea was a traditional Chinese medicinal plant used to treat inflammation-related diseases with little scientific validation. Twelve flavonoids, including two new compounds namely plantanones A (1) and B (2), were isolated from the flowers of Hosta plantaginea. Their structures were elucidated by NMR and HRMS as well as comparison with literature data. All of the isolated compounds showed significant inhibitory activities against ovine COX-1 and COX-2 at a concentration of 50 μM, with inhibition ratios from 53.00% to 80.55% for COX-1 and from 52.19% to 66.29% for COX-2. Further detailed testing showed that compounds 1, 2, 4 and 12 inhibited the COX-1 and COX-2 enzymes with IC50 values 12.90–33.37 μM and 38.32–46.16 μM, respectively. Moreover, the antioxidant effects of these isolates against DPPH free radical-scavenging were also evaluated in vitro, and a tight structure-activity relationship was discussed. Our results suggested that the anti-inflammatory and antioxidant activities of H. plantaginea flowers are partly attributed to these flavonoids.
Introduction
Plant flavonoids are an important class of secondary metabolites, showing a broad spectrum of biological activities, such as anti-inflammatory, antioxidant, antiviral, antihyperlipidemic, cytotoxic and anti-cathepsin K effects.1–4 Furthermore, the antiphlogistic effects of flavonoids probably derives from pleiotropic actions on different molecular targets and pathways, including antioxidant and pro-oxidant effects, interaction with inflammatory and pro-inflammatory proteins and enzymes.1,5 In general, flavonoids exhibited inhibitory effects against cyclooxygenase (COX)-1 and 2, but they usually inhibit COX-1 preferentially over COX-2.
Hosta plantaginea (Lam.) Aschers, belonging to the Liliaceae family, a rich source of biologically active secondary metabolites, was widely used as a traditional Chinese medicine and known as Yu zan (Chinese:
).1,6 It's recorded that the flowers of H. plantaginea were an effective traditional Mongolian medicine for treating various inflammatory and painful diseases, such as sore throat, mute, lung heat and toxic heat.8 Previous phytochemical studies on H. plantaginea afforded structurally diverse and biologically active compounds, such as steroids, alkaloids, flavonoids and fatty acids, and some of them showed potent anti-inflammatory, cytotoxic, antibacterial, antiviral, and antioxidant activities.7,9 In order to provide more evidence regarding the efficacy and safety of H. plantaginea flowers in clinical applications, we investigated the chemical constituents of H. plantaginea flowers, which led to the isolation of 12 flavonoids, including two new compounds, plantanones A (1) and B (2), together with 10 known ones (3–12) (Fig. 1). All isolated compounds were evaluated for their anti-inflammatory activities by measuring their inhibitory activities against COX-1 and 2 in vitro. Moreover, the antioxidant effects of these isolates against DPPH free radical-scavenging were also evaluated in vitro, and a tight structure-activity relationship was discussed.
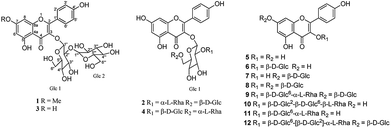 |
| Fig. 1 Chemical structures of compounds 1–12. | |
Results and discussion
Plantanone A (1) was obtained as a yellow amorphous powder with a molecular formula of C28H32O16 on the basis of the HR-ESI-MS (m/z 647.15826, calcd for 647.15888 [M + Na]+). The UV-vis absorption bands at λmax 267 and 347 nm, suggesting the presence of a kaempferol skeleton in 1.10 The NMR features of 1 (Table 1) indicated the presence of a flavonoid diglycoside containing typical signals of two anomeric protons at δH 5.72 (1H, d, J = 6.9 Hz, H-1′′) and 4.62 (1H, d, J = 7.8 Hz, H-1′′′), which had HSQC correlations with the relevant anomeric carbons at δC 97.9 (C-1′′) and 104.1 (C-1′′′). The acid hydrolysis and HPLC analysis revealed that compound 1 only has β-D-glucoses (tR 19.5 min), which was confirmed by the coupling constants of the anomeric protons [H-1′′, 6.9 Hz and H-1′′′, 7.8 Hz] and the chemical shifts of two hydroxymethyl groups [δC 60.8 (C-6′′) and 60.5 (C-6′′′)]. Moreover, the presence of two meta-coupled [δH 6.74 (1H, d, J = 1.8 Hz, H-8) and 6.36 (1H, d, J = 1.8 Hz, H-6)] and four ortho-coupled [δH 8.08–8.10 (H-2′ and H-6′) and 6.91–6.93 (H-3′ and H-5′), each 2H, d, J = 8.7 Hz] aromatic protons is typical for a kaempferol skeleton. The 1H and 13C NMR data of 1 (Table 1) were similar to those of kaempferol-3-O-sophoroside (3),11 except for the presence of an additional methoxy group [δH 3.86 (3H, s) and δC 56.1] in 1. This methoxy group positioned at C-7 was confirmed by the HMBC correlation (Fig. 2) from 7-OCH3 to C-7 (δC 165.0). Attached positions of the first glucose at C-3 and the second glucose at C-2′′ were indicated by HMBC correlations from H-1′′ (δH 5.72) to C-3 (δC 133.1) and from H-1′′′ (δH 4.62) to C-2′′ (δC 82.4), respectively. Detailed analysis of the 1H–1H COSY, HSQC and HMBC experiments (Fig. 2) clearly confirmed the structure of 1.
Table 1 1H and 13C NMR data for 1 in DMSO-d6 (δ in ppm, J in Hz)
No. |
δC |
δH (J in Hz) |
No. |
δC |
δH (J in Hz) |
2 |
156.2 |
— |
3′′ |
77.5 |
3.06–3.10 (3H, m) |
3 |
133.1 |
— |
4′′ |
69.7 |
3.12–3.17 (2H, m) |
4 |
177.6 |
— |
5′′ |
76.6 |
3.46–3.52 (4H, m) |
4a |
104.9 |
— |
6′′ |
60.8 |
3.60 (1H, d, 11.2) |
5 |
160.9 |
— |
3.46–3.52 (4H, m) |
6 |
97.9 |
6.36 (1H, d, 1.8) |
Glc 2 |
|
|
7 |
165.0 |
— |
1′′′ |
104.1 |
4.62 (1H, d, 7.8) |
8 |
92.2 |
6.74 (1H, d, 1.8) |
2′′′ |
74.4 |
3.06–3.10 (3H, m) |
8a |
155.9 |
— |
3′′′ |
77.0 |
3.12–3.17 (2H, m) |
1′ |
120.8 |
— |
4′′′ |
69.6 |
3.06–3.10 (3H, m) |
2′, 6′ |
131.0 |
8.08–8.10 (2H, d, 8.7) |
5′′′ |
76.7 |
3.18 (1H, m) |
3′, 5′ |
115.3 |
6.91–6.93 (2H, d, 8.7) |
6′′′ |
60.5 |
3.46–3.52 (4H, m) |
4′ |
160.1 |
— |
3.26 (1H, m) |
Glc 1 |
|
|
5-OH |
— |
12.65 (1H, s) |
1′′ |
97.9 |
5.72 (1H, d, 6.9) |
4′-OH |
— |
10.22 (1H, s) |
2′′ |
82.4 |
3.46–3.52 (4H, m) |
7-OMe |
56.1 |
3.86 (3H, s) |
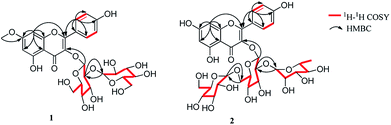 |
| Fig. 2 The selected 1H–1H COSY and HMBC correlations of compounds 1 and 2. | |
Plantanone B (2) was isolated as a yellow amorphous powder with a molecular formula of C33H40O20, determined by a quasi-molecular ion peak at m/z 779.20052, calcd for 779.20027 [M + Na]+ in its HR-ESI-MS spectrum. It also showed UV maxima absorption bands at λmax 265 and 347 nm. The NMR features of 2 (Table 2) indicated the presence of a flavonoid triglycoside containing typical signals of three anomeric protons at δH 5.44 (1H, d, J = 7.6 Hz, H-1′′) and 4.38–4.40 (2H, m, H-1′′′ and 1′′′′), which had HSQC correlations with the relevant anomeric carbons at δC 100.4 (C-1′′), 100.8 (C-1′′′) and 104.0 (C-1′′′′). The acid hydrolysis and HPLC analysis revealed that compound 2 has β-D-glucose (tR 19.5 min) and α-L-rhamnose (tR 32.8 min), which were confirmed by the chemical shifts of two hydroxymethyl groups [δC 61.1 (C-6′′′′) and 66.6 (C-6′′)] and methyl group [δC 17.8 (C-6′′′). Moreover, the presence of two meta-coupled [δH 6.41 (1H, br.s, H-8) and 6.20 (1H, br.s, H-6)] and four ortho-coupled [δH 7.95–8.00 (H-2′ and H-6′) and 6.87–6.90 (H-3′ and H-5′), each 2H, d, J = 8.4 Hz] aromatic protons is typical for a kaempferol skeleton. By HSQC and TOCSY experiments, the three sugars spin systems were assigned to two β-glucoses [δC 100.4, 86.9, 75.3, 73.1, 68.4 and 66.6 for the first spin system, as well as δC 104.0, 76.9, 76.2, 73.9, 70.6 and 61.1 for the second spin system ] and one α-rhamnose [δC 100.8, 71.8, 70.4, 70.2, 68.4 and 17.8]. Comparison of the 1H and 13C NMR data for 2 (Table 2) and kaempferol-3-O-β-D-glucopyranosyl-(1 → 2)-[α-L-rhamnopyranosyl-(1 → 6)]-β-D-glucopyranoside (4)11 revealed that they possess the same aglycon and sugar chain at C-3 of aglycon, except for the linkage site of the second β-glucose moiety, which was connected to C-6′′ in 2 instead of to C-2′′ in 4, according to the HMBC correlations of H-1′′′′ to C-6′′/C-3′′′′, H-6′′ to C-1′′′′, H-1′′′ to C-2′′/C-3′′′ and H-2′′ to C-1′′′, together with the upfield chemical shift of C-2′′ (ΔC +2.5).12 Based on the above evidence, the structure of 2 was determined to be kaempferol-3-O-β-D-glucopyranosyl-(1 → 6)-[α-L-rhamnopyranosyl-(1 → 2)]-β-D-glucopyranoside and named as plantanone B.
Table 2 1H and 13C NMR data for 2 in DMSO-d6 (δ in ppm, J in Hz)
No. |
δC |
δH (J in Hz) |
No. |
δC |
δH (J in Hz) |
2 |
156.7 |
— |
6′′ |
66.6 |
3.67–3.71 (2H, m) |
3 |
133.1 |
— |
3.33–3.35 (2H, m) |
4 |
177.3 |
— |
Rha |
|
|
4a |
104.0 |
— |
1′′′ |
100.8 |
4.37–4.40 (2H, m) |
5 |
161.2 |
— |
2′′′ |
70.4 |
3.26–3.29 (2H, m) |
6 |
98.8 |
6.20 (1H, br.s) |
3′′′ |
70.2 |
3.38–3.40 (3H, m) |
7 |
164.2 |
— |
4′′′ |
71.8 |
3.09 (1H, m) |
8 |
93.8 |
6.41 (1H, br.s) |
5′′′ |
68.4 |
3.17–3.22 (3H, m) |
8a |
156.5 |
— |
6′′′ |
17.8 |
0.98 (3H, d, 5.0) |
1′ |
120.9 |
— |
Glc 2 |
|
|
2′, 6′ |
130.9 |
7.95–8.00 (2H, d, 8.4) |
1′′′′ |
104.0 |
4.37–4.40 (2H, m) |
3′, 5′ |
115.2 |
6.87–6.90 (2H, d, 8.4) |
2′′′′ |
73.9 |
3.38–3.40 (3H, m) |
4′ |
159.9 |
— |
3′′′′ |
76.2 |
3.17–3.22 (3H, m) |
Glc 1 |
|
|
4′′′′ |
70.6 |
3.04–3.08 (2H, m) |
1′′ |
100.4 |
5.44 (1H, d, 7.6) |
5′′′′ |
76.9 |
3.17–3.22 (3H, m) |
2′′ |
86.9 |
3.48 (1H, m) |
6′′′′ |
61.1 |
3.67–3.71 (2H, m) |
3′′ |
73.1 |
3.04–3.08 (2H, m) |
3.38–3.40 (3H, m) |
4′′ |
68.4 |
3.26–3.29 (2H, m) |
5-OH |
— |
12.58 (1H, br.s) |
5′′ |
75.3 |
3.33–3.35 (2H, m) |
7-OH |
— |
10.85 (1H, br.s) |
|
|
|
4′-OH |
— |
10.18 (1H, br.s) |
The structures of the known compounds were elucidated as kaempferol-3-O-sophoroside (3),11 kaempferol-3-O-β-D-glucopyranosyl-(1 → 2)-[α-L-rhamnopyranosyl-(1 → 6)]-β-D-glucopyranoside (4),13 kaempferol (5),13 astragalin (6),14 kaempferol-7-O-β-D-glucopyranoside (7),15 kaempferol-3,7-di-O-β-D-glucopyranoside (8),16 kaempferol-3-O-rutinoside-7-O-glucopyranoside (9),11 kaempferol-3-O-α-L-rhamnopyranosyl-(1 → 6)-β-D-glucopyranosyl-(1 → 2)-β-D-glucopyranoside (10),17 kaempferol-3-O-rutinoside (11)13 and kaempferol-3-O-(2G-glucosylrutinoside)-7-O-glucoside (12),11 according to their spectroscopic data and by comparison with literature data. Among them, compounds 4 and 12 from this species, compounds 6, 8 and 10 from the genus Hosta, while compounds 6 and 10 from the Liliaceae family for the first time.
Considering the pronounced treatment effect of H. plantaginea flowers on inflammation-related diseases, compounds 1–12 were evaluated for their anti-inflammatory and antioxidant activities in vitro. All of isolated compounds showed significant inhibitory activities against ovine COX-1 and COX-2 at concentration of 50 μM, with the inhibition rations from 53.00% to 80.55% for COX-1 and from 52.19% to 66.29% for COX-2 (Table 3). Due to the similar structures but different potency, we further tested compounds 1, 2, 4 and 12 at several concentration levels, and they showed significant COX-1 inhibitory activities with IC50 values of 33.37 ± 0.28, 21.78 ± 0.20, 12.90 ± 0.11 and 20.74 ± 0.41 μM, respectively, comparable to that of the positive control celecoxib with an IC50 value of 9.00 ± 0.60 μM. Meanwhile, compounds 1, 2, 4 and 12 also displayed moderate COX-2 inhibitory activities with IC50 values of 46.16 ± 0.41, 44.01 ± 0.42, 45.21 ± 0.43 and 38.32 ± 0.34 μM, respectively, comparable to that of celecoxib with an IC50 value of 1.04 ± 0.10 μM. Unfortunately, compounds 1, 2, 4 and 12 inhibit COX-1 preferentially over COX-2 with selectivity index (SI) values were less than 1, comparable to that of celecoxib with SI value of 8.65.
Table 3 Inhibitory activity of compounds 1–12 on COX assay
Comp. |
Inhibitionsa (%) |
IC50 (μM) |
SIb |
COX-1 |
COX-2 |
COX-1 |
COX-2 |
Inhibition (%) values were tested at 50 μM and expressed as the mean S.D. (n = 3). SI (Selectivity Index) = IC50 (COX-1)/IC50 (COX-2). |
1 |
62.56 ± 0.56 |
56.24 ± 0.43 |
33.37 ± 0.28 |
46.16 ± 0.41 |
0.72 |
2 |
76.18 ± 0.62 |
57.70 ± 0.51 |
21.78 ± 0.20 |
44.01 ± 0.42 |
0.49 |
3 |
61.10 ± 0.47 |
52.67 ± 0.49 |
— |
— |
— |
4 |
72.61 ± 0.71 |
53.81 ± 0.52 |
12.90 ± 0.11 |
45.21 ± 0.43 |
0.28 |
5 |
61.43 ± 0.35 |
52.19 ± 0.26 |
— |
— |
— |
6 |
66.94 ± 0.45 |
55.11 ± 0.42 |
— |
— |
— |
7 |
58.83 ± 0.36 |
66.29 ± 0.38 |
— |
— |
— |
8 |
62.88 ± 0.54 |
54.78 ± 0.51 |
— |
— |
— |
9 |
53.00 ± 0.41 |
57.54 ± 0.50 |
— |
— |
— |
10 |
53.32 ± 0.35 |
57.54 ± 0.32 |
— |
— |
— |
11 |
55.43 ± 0.38 |
58.35 ± 0.39 |
— |
— |
— |
12 |
80.55 ± 0.42 |
60.78 ± 0.51 |
20.74 ± 0.41 |
38.32 ± 0.34 |
0.54 |
Celecoxib |
— |
— |
9.00 ± 0.60 |
1.04 ± 0.10 |
8.65 |
The antioxidant activity of compounds 1–12 was measured by the DPPH method and the results were summarized in Table 4. Compounds 5 and 7 exhibited very strong or strong antioxidant activities, with IC50 values of 36.3 ± 1.1 and 77.6 ± 2.4 μM, comparable to that of the positive control L-ascorbic acid (Vc) with an IC50 value of 33.9 ± 1.1 μM. Meanwhile, compounds 2–4, 6, 10 and 11 exhibited moderate antioxidant activities, with IC50 values of 169.8 ± 5.2, 195.0 ± 9.2, 257.0 ± 19.9, 100.0 ± 3.2, 208.9 ± 7.3 and 128.8 ± 9.2 μM, respectively. However, compounds 1, 8, 9 and 12 showed insignificant antioxidant activities, with IC50 values from 302.0 to 467.7 μM. The above experimental results suggested that the presence of hydroxyl groups substituted on the aromatic ring seem to increase the antioxidant activity, and the 3-OH more important than 7-OH for the antioxidant activity, which were consistent with previously reports.18,19
Table 4 Antioxidant activity of compounds 1–12
Comp. |
IC50 (μM) |
Compounds |
IC50 (μM) |
Compounds |
IC50 (μM) |
|
1 |
467.7 ± 17.9 |
5 |
36.3 ± 1.1 |
9 |
398.1 ± 26.3 |
2 |
169.8 ± 5.2 |
6 |
100.0 ± 3.2 |
10 |
208.9 ± 7.3 |
3 |
195.0 ± 9.2 |
7 |
77.6 ± 2.4 |
11 |
128.8 ± 9.2 |
4 |
257.0 ± 19.9 |
8 |
407.4 ± 22.1 |
12 |
302.0 ± 15.1 |
Vc |
33.9 ± 1.1 |
|
|
|
|
Conclusions
This study investigated chemically the flowers of H. plantaginea and isolated two flavonol glycosides, plantanones A (1) and B (2) along with 10 known ones (3–12). To the best of knowledge, this is the first report of compounds 4 and 12 from this species, compounds 6, 8 and 10 from the genus Hosta, while compounds 6 and 10 from the Liliaceae family. All of isolated compounds showed significant inhibitory activities against ovine COX-1 and COX-2 at concentration of 50 μM, with the inhibition rations from 53.00% to 80.55% for COX-1 and from 52.19% to 66.29% for COX-2. Moreover, compounds 1, 2, 4 and 12 showed significant COX-1 and moderate COX-2 inhibitory activities with IC50 values of 33.37 ± 0.28, 21.78 ± 0.20, 12.90 ± 0.11 and 20.74 ± 0.41 μM for COX-1, as well as 46.16 ± 0.41, 44.01 ± 0.42, 45.21 ± 0.43 and 38.32 ± 0.34 μM for COX-2, respectively. In addition, compounds 5 and 7 exhibited very strong antioxidant activities, with IC50 values of 36.3 ± 1.1 and 77.6 ± 2.4 μM. Their structure-antioxidant activity relationship was also discussed. Our results suggested that the anti-inflammatory and antioxidant activities of H. plantaginea flowers are partly attributed to these flavonoids, and support the claim for their traditional Mongolian medicine use in the treatment of inflammation-related diseases.
Experimental section
General experimental procedures
UV-vis spectra were recorded on a Shimadzu UV-1800 UV-vis recording spectrophotometer (Shimadzu, Kyoto, Japan). Fluorescence spectra were recorded on a Cary Eclipse spectrophotometer (Varian, California, USA). 1H (600 MHz), 13C (150 MHz), DEPT 135 (150 MHz) and 2D NMR (1H–1H COSY, HSQC, TOCSY and HMBC) spectra were recorded on a Bruker AV 600 spectrometer (Bruker Corporation, Fallanden, Switzerland) with TMS as internal reference, and using DMSO-d6 as solvent. HR-ESI-MS were measured on a Waters Synapt UPLC G2 TOF mass spectrometer (Waters Corporation, Manchester, UK). Column chromatographies (CCs) were carried out using D101 (Jinan Bona Biotechnology Co., Ltd., Jinan, China), silica gel (200–300 mesh, Qingdao Haiyang Chemical Group Corporation, Qingdao, China), octodecyl silica gel (ODS) (C18, 60–80 μm, YMC, Tokyo, Japan) and Sephadex LH-20 (Pharmacia) as a stationary phase. Silica gel GF254 (Qingdao Haiyang Chemical Group Corporation, Qingdao, China) was used for analytical TLC. 2,2-Di-phenyl-1-picrylhydrazyl (DPPH) was purchased from Sigma Corporation (Ronkonkoma, New York, NY, USA), L-cysteine methyl ester and O-tolyl isothiocyanate were purchased from Meilun Biotech. Co. Ltd (Dalian, China), D-glucose and L-glucose were purchased from Energy Chemical (Shanghai, China). COX inhibitor screening assay kit was purchased from Cayman Chemical Company (Ann Arbor, Michigan, USA). The analytical HPLC was performed on a Shimadzu HPLC system equipped with a LC-20AB pump, and a SPD-20A diode array detector (Shimadzu, Kyoto, Japan), using a Phenomenex Gemini C18 column (5 μm, 4.6 × 250 mm, Phenomenex Inc., CA, USA). The Preparative HPLC was performed on Shimadzu LC-6AD system equipped with a LC-6AD pump and a SPD-M20A detector (Shimadzu, Kyoto, Japan) using RP-18 columns (5 μm, 21.2 × 250 mm, Gemini, Phenomenex Inc., CA, USA; 5 μm, 10 × 250 mm, park ODS-A, YMC Co., Ltd., Kyoto, Japan) with the detector set at 220 and 254 nm.
Plant material
The flowers of Hosta plantaginea (Lam.) Aschers were collected in the town of Shanquan, Nanchuan district, Chongqing, China, in September 2014. A botanical voucher specimen of this plant (No. YZH201409) was deposited at Research Center of Natural Resources of Chinese Medicinal Materials and Ethnic Medicine, Jiangxi University of Traditional Chinese Medicine, Nanchang, China, and was identified by one of the authors (Guo-yue Zhong).
Extraction and isolation
The air-dried and powdered flowers of H. plantaginea (16.5 kg) were extracted for three times with 80% EtOH (40 L) by maceration at room temperature for three days. After filtration, combination, and solvent evaporation, the residue (6.60 kg) was dissolved in water and successively partitioned with three different kinds of solvents to afford petroleum ether (A, 363 g), ethyl acetate (B, 127 g), n-BuOH (C, 804 g), and water (5.27 kg) extracts, respectively. The n-BuOH extract (760 g) was subjected to HP20 macroporous adsorption resin column chromatography (CC) eluting with 0%, 20%, 50% and 95% aqueous EtOH to give four fractions (c1 to c4). Fr. c3 (123 g) was subjected to silica gel CC eluting with dichloromethane–CH3OH (10
:
1, 5
:
1, 1
:
1, 0
:
100, v/v) to give four fractions (c31 to c34). The subfraction c32 (9.48 g) was applied to ODS CC eluting with 20%, 30%, 50% and 100% aqueous CH3OH to give four fractions (c32a to c32d). c32c (2.11 g) was purified by preparative HPLC eluting with CH3OH/H2O (48
:
52, flow rate: 9.0 mL min−1) to afford compound 1 (224.2 mg, tR 36.1 min). c32d (0.93 g) was purified by semi-preparative HPLC eluting with CH3OH/H2O (55
:
45, flow rate: 2.5 mL min−1) to afford compound 5 (9.5 mg, tR 46.3.0 min). The subfraction c32b (5.47 g) was applied to polyamide CC eluting with 10%, 30% and 95% aqueous EtOH to give three fractions (c32b1 to c32b3). The subfraction c32b2 (6.43 g) was fractionated by Sephadex LH-20 column (100% aqueous CH3OH) and further purified by preparative HPLC eluting with CH3CN–H2O (18
:
82, flow rate: 8.0 mL min−1) to afford compounds 3 (1.12 g, tR 15.7 min), 2 (67.1 mg, tR 16.6 min), 11 (1.10 g, tR 18.2 min), 6 (390 mg, tR 19.3 min), and 7 (201 mg, tR 22.5 min). Fr. c2 (32.8 g) was subjected to MCI CC eluting with 10%, 20%, 30% and 95% aqueous EtOH to give four fractions (c2a to c2d). The subfraction c2c (6.02 g) was applied to silica gel CC eluting with dichloromethane–CH3OH (5
:
1, 3
:
1, 1
:
1, 0
:
100, v/v) to afford four subfractions (c2c1–c2c4). c2c3 (2.17 g) was purified by pre-HPLC eluting with CH3OH/H2O (35
:
65, flow rate: 10.0 mL min−1) to afford compound 4 (1.07 g, tR 20.4 min). The subfraction c2b (15.1 g) was applied to polyamide CC eluting with 10%, 20%, 30% and 95% aqueous EtOH to afford five subfractions (c2b1 to c2b5). The subfraction c2b2 (4.30 g) was purified by pre-HPLC eluting with CH3OH/H2O (30
:
70, flow rate: 10.0 mL min−1) to afford compounds 8 (1.11 g, tR 20.3 min) and 9 (1.58 g, tR 22.4 min). c2b3 (3.18 g) was purified by pre-HPLC eluting with CH3OH/H2O (40
:
60, flow rate: 9.0 mL min−1) to afford compound 10 (1.07 g, tR 19.2 min). The subfraction c2a (8.05 g) was applied to silica gel CC eluting with dichloromethane–CH3OH (1
:
1, 1
:
3, 1
:
10, v/v) to afford three subfractions (c2a1–c2a3). c2a1 (3.89 g) was subjected to Sephadex LH-20 CC eluting with CH3OH to afford three subfractions (c2a11–c2a13). c2a12 (2.59 g) was purified by pre-HPLC eluting with CH3OH/H2O (25
:
75, flow rate: 10.0 mL min−1) to afford compound 12 (1.07 g, tR 14.9 min).
Plantanone A (1). Yellow amorphous powder; UV-vis (CH3OH) λmax (log ε) 267 (4.16), 347 (4.10) nm; fluorescence spectra (CH3OH) λmax 575 nm; HR-ESI-MS m/z 647.15826 [M + Na]+, calcd for C28H32O16Na, 647.15888; 1H (DMSO-d6, 600 MHz) and 13C (DMSO-d6, 150 MHz) data see Table 1.
Plantanone B (2). Yellow amorphous powder; UV-vis (CH3OH) λmax (log ε) 267 (4.13), 347 (4.01) nm; fluorescence spectra (CH3OH) λmax 577 nm; HR-ESI-MS m/z 779.20052 [M + Na]+, calcd for C33H40O20Na, 779.20027; 1H (DMSO-d6, 600 MHz) and 13C (DMSO-d6, 150 MHz) data see Table 2.
Acid hydrolysis and HPLC analysis
The chiral derivatives of the sugars of compounds 1 and 2 were determined by the acid hydrolysis and HPLC analysis. Detailed protocol was the same as the literature described in our previously paper.9 The peaks of the standard monosaccharide derivatives were recorded at tR 17.9 (L-Glc), 19.5 (D-Glc) and 32.8 (L-Rha) min. Meanwhile, the derivative of 1 afforded one peak at tR 19.5 min (D-Glc), and the derivatives of 2 gave two peaks at tR 19.5 (D-Glc) and 32.8 (L-Rha) min.
In vitro COX-1 and COX-2 inhibitory assay
Inhibitory activities of the tested compounds towards COX-1 and COX-2 activities were determined using colorimetric COX (ovine) inhibitor screening assay kit (Cayman, no. 760111) following manufacturer's instructions, using celecoxib as a positive control.20 The 50% inhibitory concentration (IC50) values were calculated from the concentration-inhibition response curve.
Antioxidant assay
DPPH radical-scavenging activity of the tested samples were provided in a previously published paper with L-ascorbic acid as the reference drug.9
Conflicts of interest
There are no conflicts to declare.
Acknowledgements
This work was financially supported by grants from the National Natural Science Foundation of China (No. 81503357), the Natural Science Foundation of Jiangxi Province (No. 20171BBH80026 and 20171BAB215063), the China Postdoctoral Science Foundation (No. 2016M600514), the Postdoctoral Science Foundation of Jiangxi Province (2016KY50), the Jiangxi University of Traditional Chinese Medicine (2016jzzdxk004), and the Introduction Program of Scientific Researchist of Sichuan University of Science & Engineering (No. 2015RC24).
Notes and references
- Z. Hanáková, J. Hošek, Z. Kutil, V. Temml, P. Landa, T. Vaněk, D. Schuster, S. DalĺAcqua, J. Cvačka, O. Polanský and K. Šmejkal, J. Nat. Prod., 2017, 80, 999–1006 CrossRef PubMed.
- L. R. Wen, Y. P. Zhao, Y. M. Jiang, L. M. Yu, X. F. Zeng, J. L. Yang, M. M. Tian, H. L. Liu and B. Yang, Free Radical Biol. Med., 2017, 110, 92–101 CrossRef PubMed.
- W. Peng, L. Wang, X. H. Qiu, Y. P. Jiang, L. Pan, X. G. Jia, L. P. Qin and C. J. Zheng, Fitoterapia, 2016, 114, 105–109 CrossRef PubMed.
- W. J. Yuan, J. B. Yuan, J. B. Peng, Y. Q. Ding, J. X. Zhu and G. Ren, Fitoterapia, 2017, 117, 133–137 CrossRef PubMed.
- J. Hošek and K. Šmejkal, in compendium of inflammatory diseases, ed. M. J. Pharnham, Birhause Basel, Switzerland, 2016, pp. 1–17 Search PubMed.
- Editorial Committee of the Flora of China of Chinese Academy of Science, Zhongguo Zhiwu Zhi (Flora of China), Science Press, Beijing, China, 1980, vol. 14, pp. 49–50 Search PubMed.
- J. W. He, L. Yang and G. Y. Zhong, Chin. Tradit. Herb. Drugs, 2016, 47, 4295–4300 Search PubMed.
- State Administration of Traditional Chinese Medicine, Zhonghua Bencao (Chinese Materia Medica), Shanghai Science & Technology Press, Shanghai, China, 1999, vol. 8, pp. 107–108 Search PubMed.
- L. Yang, S. T. Jiang, Q. G. Zhou, G. Y. Zhong and J. W. He, Molecules, 2017, 22, 1825 CrossRef PubMed.
- B. Li, Y. Ni, L. J. Zhu, F. B. Wu, F. Yan, X. Zhang and X. S. Yao, J. Nat. Prod., 2015, 78, 987–995 CrossRef PubMed.
- J. Budzianowski, Phytochemistry, 1990, 29, 3643–3647 CrossRef PubMed.
- E. Corradi, M. D. Mieri, L. Cadisch, C. Abbet, M. Hamburger and O. Potterat, Chem. Biodiversity, 2016, 13, 188–197 Search PubMed.
- H. X. Xie, J. H. Zhang, H. G. Zhang and P. F. Xue, Chin. Pharm. J., 2009, 44, 733–735 Search PubMed.
- F. Y. Ni, X. Xie, L. Liu, Y. W. Zhao, W. Z. Huang, Z. Z. Wang and W. Xiao, Chin. Tradit. Herb. Drugs, 2016, 47, 214–218 Search PubMed.
- B. Y. Yang, T. Li, R. Guo, Q. H. Wang and H. X. Kuang, Chin. Tradit. Herb. Drugs, 2013, 44, 2803–2807 Search PubMed.
- D. Lang, Y. F. Liu, Z. Y. Hao, H. Luo, Y. Wang, C. L. Zhang, Q. J. Zhang, N. Y. Chen and D. Q. Yu, China J. Chin. Mater. Med., 2015, 40, 103–107 Search PubMed.
- Y. P. Tang, Y. F. Li, J. Hu and F. C. Lou, J. Asian Nat. Prod. Res., 2002, 4, 123–128 CrossRef PubMed.
- M. Musialik, R. Kuzmicz, T. S. Pawlowski and G. Litwinienko, J. Org. Chem., 2009, 74, 2699–2709 CrossRef PubMed.
- A. Mezzetti, S. Protti, C. Lapouge and J. P. Cornard, Phys. Chem. Chem. Phys., 2011, 13, 6858–6864 RSC.
- G. O. Ochieng, S. A. Opiyo, E. W. Mureka and I. O. Ishola, Fitoterapia, 2017, 119, 168–174 CrossRef PubMed.
Footnote |
† Electronic supplementary information (ESI) available: 1D and 2D NMR spectra, UV-vis absorption spectra and fluorescence spectra of new compounds 1 and 2. See DOI: 10.1039/c8ra00443a |
|
This journal is © The Royal Society of Chemistry 2018 |
Click here to see how this site uses Cookies. View our privacy policy here.