DOI:
10.1039/C7QM00613F
(Research Article)
Mater. Chem. Front., 2018,
2, 791-795
Synthesis of optically active π-stacked compounds based on planar chiral tetrasubstituted [2.2]paracyclophane†
Received
27th December 2017
, Accepted 31st January 2018
First published on 1st February 2018
Abstract
Optically active X-shaped compounds based on planar chiral [2.2]paracyclophane were synthesized. These compounds composed of two different π-electron systems stacked at central aromatic rings through chemoselective Sonogashira–Hagihara coupling. The optical and chiroptical properties of the obtained compounds were investigated, which exhibited large molar absorption coefficients, good photoluminescence quantum efficiency, and intense circularly polarized luminescence (CPL) with large dissymmetry factors on the order of 10−3, indicating that the compounds are excellent CPL emitters.
Introduction
[2.2]Paracyclophane is a unique molecule consisting of face-to-face benzene rings in proximity; the distance between the two benzene rings is approximately 3.0 Å.1 [2.2]Paracyclophane has been utilized as a scaffold to construct π-stacked structures; various π-electron systems can be stacked in a molecule. 4,12-Disubstituted and 4,7,12,15-tetrasubstituted [2.2]paracyclophanes are representative scaffolds, as shown in Fig. 1.2 For example, when using 4,12-disubstituted [2.2]paracyclophane to construct a π-stacked structure, the terminal benzene rings of the π-electron systems are stacked (Fig. 1a). Using 4,7,12,15-tetrasubstituted [2.2]paracyclophane as a scaffold, the central phenylenes of the π-electron systems are stacked (Fig. 1b). Thus, the π-stacked position of the π-electron system can be tuned by the choice of the [2.2]paracyclophane scaffold.
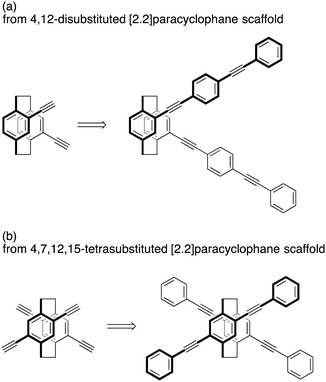 |
| Fig. 1 [2.2]Paracyclophane scaffolds to construct π-stacked structures. | |
[2.2]Paracyclophane compounds with substituent(s) exhibit planar chirality due to the restricted rotational motion of the π-stacked benzene rings, depending on the substitution positions.3 4,12-Disubstituted and 4,7,12,15-tetrasubstituted [2.2]paracyclophanes are also planar chiral compounds. Recently, we have developed a practical optical resolution of 4,12-disubstituted [2.2]paracyclophane4,5 and the first optical resolution of 4,7,12,15-tetrasubstituted [2.2]paracyclophane,6,7 leading to enantiopure scaffolds to synthesize optically active compounds with higher-ordered chiral structures. In that study, optically active V-shaped,4 X-shaped,8 8-shaped,6a,b and double-helical6c compounds were synthesized which emit excellent circularly polarized luminescence (CPL) by photo-excitation.
The optical resolution of 4,7,12,15-tetrasubstituted [2.2]paracyclophane is carried out with 4-hydroxy-7,12,15-tribromo[2.2]paracyclophane (rac-1) using a chiral auxiliary, and then, the hydroxyl group is converted to the trifluoromethylsulfonyl group to afford enantiopure compound 2 (Fig. 2).6a The catalytic system, Pd2(dba)3/P(t-Bu)3/CuI, was found for chemoselective Sonogashira–Hagihara coupling;9 only bromo groups reacted, and the trifluoromethylsulfonyl group remained (Fig. 2).6a This chemoselective coupling makes it possible to synthesize new types of X-shaped compounds with different π-electron systems stacked in molecules (Fig. 2). In this study, we demonstrate the stacking of different π-electron systems (p-phenylene ethynylenes) consisting of three benzene rings at the central phenylene units. The synthetic routes and optical properties of the obtained π-stacked compounds are also shown.
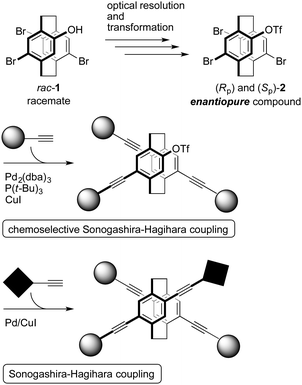 |
| Fig. 2 Strategy to obtain X-shaped compounds with different π-electron systems. | |
Results and discussion
Schemes 1 and 2 show the synthetic routes to the target X-shaped compounds with different π-electron systems stacked; the synthetic routes from (Rp)-26a are also shown. Chemoselective Sonogashira–Hagihara coupling of (Rp)-2 with trimethylsilylacetylene in the presence of catalytic amounts of Pd2(dba)3 (dba = dibenzylideneacetone), (t-Bu)3P·HB4, and CuI afforded triyne (Rp)-3 in 86% isolated yield, as shown in Scheme 1. Sonogashira–Hagihara coupling of (Rp)-3 with p-methoxyphenylacetylene using PdCl2(dppf)·CH2Cl2 (dppf = 1,1′-bis(diphenylphosphino)ferrocene) and CuI afforded the corresponding tetrayne (Rp)-4 in 77% isolated yield. The trimethylsilyl group could be readily removed by reaction with K2CO3 in MeOH to obtain (Rp)-5 in 89% isolated yield. Finally, Sonogashira–Hagihara coupling of (Rp)-5 with iodobenzene using Pd2(dba)3, dppf, and CuI afforded the target compound (Rp)-6 in 74% isolated yield, as shown in Scheme 1.10
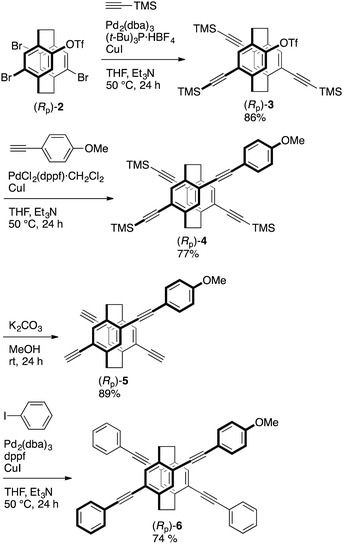 |
| Scheme 1 Synthesis of (Rp)-6. | |
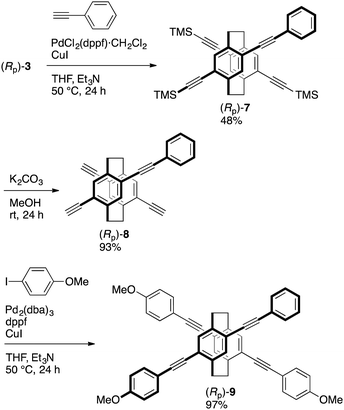 |
| Scheme 2 Synthesis of (Rp)-9. | |
The same synthetic strategy produced the other target compound (Rp)-9 (Scheme 2). Triyne (Rp)-3 was reacted with phenylacetylene using PdCl2(dppf)·CH2Cl2 and CuI to obtain tetrayne (Rp)-7 in 48% isolated yield. Its trimethylsilyl groups were also removed by K2CO3 in MeOH to obtain (Rp)-8 in 93% isolated yield. Sonogashira–Hagihara coupling of (Rp)-8 with p-iodoanisole using Pd2(dba)3, dppf, and CuI afforded compound (Rp)-9 in 97% isolated yield, as shown in Scheme 2.10
The optical properties of compounds (both enantiomers) 6 and 9 were evaluated by ultraviolet (UV) and photoluminescence (PL) spectroscopies. Fig. 3 shows the UV and PL spectra of (Rp)-6 and (Rp)-9 in their dilute CHCl3 solutions (1.0 × 10−5 M). Both compounds exhibited similar optical behaviours. Their peak tops in the UV spectra were at 352 nm and 357 nm, respectively, and those in the PL spectra appeared at 420 nm and 424 nm, respectively. The absolute PL quantum efficiencies (Φlum) of (Rp)-6 and (Rp)-9 were estimated to be 0.71 and 0.73, respectively. PL lifetime decay curves were fitted with a single exponential equation, and their lifetimes (τ) were calculated to be 3.57 ns and 3.00 ns, respectively. In addition, we have prepared two kinds of optically active X-shaped compounds: without a methoxy group8a and with four methoxy groups8b (Table S1 in the ESI†). As the number of methoxy groups increased, the spectra were slightly red-shifted, Φ values improved, and τ values decreased. The PL spectra of (Rp)-6 and (Rp)-9 were broad without any vibronic structure, and the τ values were relatively long (>3 ns). It is known that the excited state is delocalized in the whole molecule in the case of close energy levels between through-bond and through-space states.2b,d,e,i,j Thus, the emission mechanisms of (Rp)-6 and (Rp)-9 are identical to those of previous studies, and there are no essential differences among these compounds.
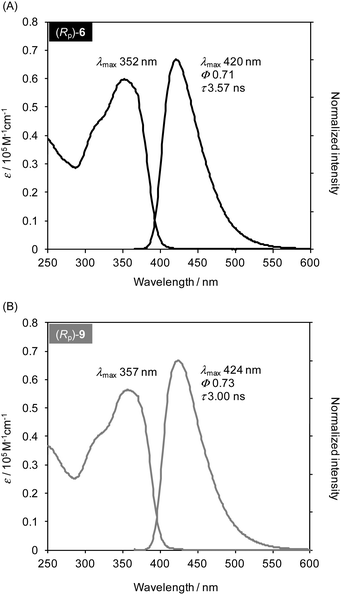 |
| Fig. 3 UV and PL spectra of (A) (Rp)-6 and (B) (Rp)-9 in CHCl3 (1.0 × 10−5 M, excited at each absorption maximum for the PL spectrum). | |
The chiroptical properties of both enantiomers of 6 and 9 were investigated using circular dichroism (CD) and CPL spectra. The CD and the absorption spectra of both enantiomers of 6 and 9 in dilute CHCl3 (1.0 × 10−5 M) are shown in Fig. 4A and B. In both cases, the absorption peaks around 350 nm are the typical π–π* transition bands of p-phenylene-ethynylenes, and clear Cotton effects were observed in the absorption bands. As shown in the CD spectra, chirality was induced in the arylene–ethynylene moieties by the planar chiral [2.2]paracyclophane moiety.
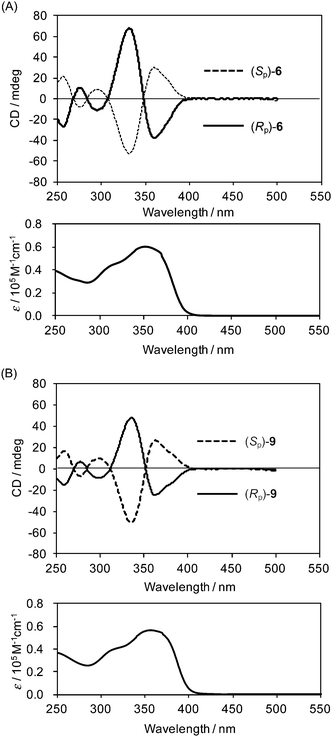 |
| Fig. 4 CD and UV spectra of (A) enantiomers of 6 and (B) enantiomers of 9 in CHCl3 (1.0 × 10−5 M). | |
Fig. 5 shows the CPL and PL spectra of (Sp)- and (Rp)-6 and 9 in dilute CHCl3 (1.0 × 10−5 M), and the glum spectra are also included. The glum is given by the following equation: 2(Ileft − Iright)/(Ileft + Iright), where Ileft and Iright indicate the luminescence intensities of left- and right-handed CPL, respectively.11,12 Both compounds 6 and 9 exhibited mirror image spectra. Positive and negative signals were observed for (Sp)- and (Rp)-isomers, respectively. These behaviours were the same as those reported in previous studies (Table S1 in the ESI†). The positive/negative signals were identical to those of the longest wavelength in the corresponding CD spectra, indicating the same orientations of dipole moments between electric and magnetic transitions in the ground and excited states. The absolute glum values between the enantiomers of 6 and 9 were estimated to be approximately 1.5 × 10−3 and 1.7 × 10−3, respectively. Compounds 6 and 9 exhibited relatively large ε and good Φlum (>0.7), in addition to the large glum value on the order of 10−3. Therefore, optically active X-shaped compounds are promising CPL materials.
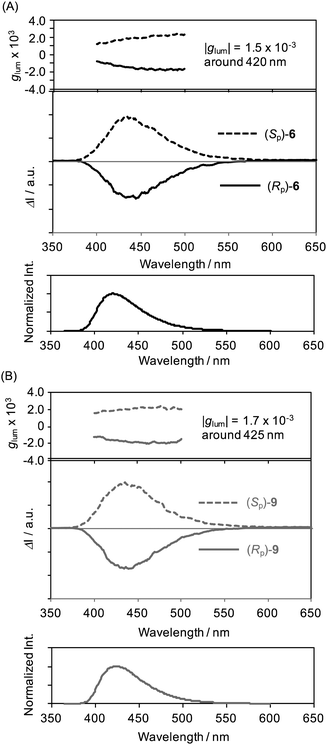 |
| Fig. 5 CPL and PL spectra, including glum spectra, of (A) enantiomers of 6 and (B) enantiomers of 9 in CHCl3 (1.0 × 10−5 M, excited at 280 nm). | |
Conclusions
In summary, planar chiral [2.2]paracyclophane-based optically active X-shaped compounds were synthesized. Different π-electron systems could be stacked at the central phenylene units by the chemoselective Sonogashira–Hagihara coupling using a Pd2(dba)3/(t-Bu)3P/CuI catalytic system. Although essential differences between compounds consisting of different π-electron systems and the same π-electron systems were not observed, the compounds exhibited good emission behaviours, resulting in an excellent CPL emitter with a large ε, good Φlum, and a large glum value on the order of 10−3. The strategy in this study can stack two different π-electron systems; thus, our next target is the synthesis of optically active π-stacked compounds consisting of strong donor and acceptor π-electron systems. In addition, the systematic synthesis of planar chiral [2.2]paracyclophane-based optically active compounds by changing the stacking position4c and orientation of the stacked-π-electron systems will be studied to elucidate the CPL emission mechanism of the planar chiral [2.2]paracyclophane.
Conflicts of interest
There are no conflicts to declare.
Acknowledgements
This work was supported by Grants-in-Aid for Scientific Research on Innovative Areas (No. 17H05165 for Y. M. and 17H05148 for T. O.) “π-Figuration” from the Ministry of Education, Culture, Sports, Science and Technology, Japan. The financial support from the Nagase Science and Technology Foundation is also acknowledged. The authors are grateful to Professor Naoto Tamai (Department of Chemistry, School of Science and Technology, Kwansei Gakuin University) for PL lifetime measurements.
Notes and references
-
(a)
F. Vögtle, Cyclophane Chemistry: Synthesis, Structures and Reactions, John Wiley & Sons, Chichester, 1993 Search PubMed;
(b)
Modern Cyclophane Chemistry, ed. R. Gleiter and H. Hopf, Wiley-VCH, Weinheim, Germany, 2004 Search PubMed;
(c) H. Hopf, Angew. Chem., Int. Ed., 2008, 47, 9808–9812 CrossRef CAS PubMed.
- Representative examples are shown.
(a) G. C. Bazan, W. J. Oldham Jr., R. J. Lachicotte, S. Tretiak, V. Chernyak and S. Mukamel, J. Am. Chem. Soc., 1998, 120, 9188–9204 CrossRef CAS;
(b) S. Wang, G. C. Bazan, S. Tretiak and S. Mukamel, J. Am. Chem. Soc., 2000, 122, 1289–1297 CrossRef CAS;
(c) J. Zyss, I. Ledoux, S. Volkov, V. Chernyak, S. Mukamel, G. P. Bartholomew and G. C. Bazan, J. Am. Chem. Soc., 2000, 122, 11956–11962 CrossRef CAS;
(d) G. P. Bartholomew and G. C. Bazan, Acc. Chem. Res., 2001, 34, 30–39 CrossRef CAS PubMed;
(e) G. P. Bartholomew and G. C. Bazan, Synthesis, 2002, 1245–1255 CAS;
(f) G. P. Bartholomew and G. C. Bazan, J. Am. Chem. Soc., 2002, 124, 5183–5196 CrossRef CAS PubMed;
(g) D. S. Seferos, D. A. Banach, N. A. Alcantar, J. N. Israelachvili and G. C. Bazan, J. Org. Chem., 2004, 69, 1110–1119 CrossRef CAS PubMed;
(h) G. P. Bartholomew, M. Rumi, S. J. K. Pond, J. W. Perry, S. Tretiak and G. C. Bazan, J. Am. Chem. Soc., 2004, 126, 11529–11542 CrossRef CAS PubMed;
(i) J. W. Hong, H. Y. Woo and G. C. Bazan, J. Am. Chem. Soc., 2005, 127, 7435–7443 CrossRef CAS PubMed;
(j) G. C. Bazan, J. Org. Chem., 2007, 72, 8615–8635 CrossRef CAS PubMed.
-
(a) D. J. Cram and N. L. Allinger, J. Am. Chem. Soc., 1955, 77, 6289–6294 CrossRef CAS;
(b)
V. Rozenberg, E. Sergeeva and H. Hopf, in Modern Cyclophane Chemistry, ed., R. Gleiter and H. Hopf, Wiley-VCH, Weinheim, Germany, 2004, pp. 435–462 Search PubMed;
(c) G. J. Rowlands, Org. Biomol. Chem., 2008, 6, 1527–1534 RSC;
(d) S. E. Gibson and J. D. Knight, Org. Biomol. Chem., 2003, 1, 1256–1269 RSC;
(e) A. A. Aly and A. B. Brown, Tetrahedron, 2009, 65, 8055–8089 CrossRef CAS;
(f) J. Paradies, Synthesis, 2011, 3749–3766 CrossRef CAS.
-
(a) Y. Morisaki, R. Hifumi, L. Lin, K. Inoshita and Y. Chujo, Chem. Lett., 2012, 41, 990–992 CrossRef CAS;
(b) Y. Morisaki, R. Hifumi, L. Lin, K. Inoshita and Y. Chujo, Polym. Chem., 2012, 3, 2727–2730 RSC;
(c) Y. Morisaki, K. Inoshita and Y. Chujo, Chem. – Eur. J., 2014, 20, 8386–8390 CrossRef CAS PubMed.
- For other optical resolutions of 4,12-disubstituted [2.2]paracyclophane, see:
(a) P. J. Pye, K. Rossen, R. A. Reamer, N. N. Tsou, R. P. Volante and P. J. Reider, J. Am. Chem. Soc., 1997, 119, 6207–6208 CrossRef CAS;
(b) K. Rossen, P. J. Pye, A. Maliakal and R. P. Volante, J. Org. Chem., 1997, 62, 6462–6463 CrossRef CAS;
(c) R. Zhuravsky, Z. Starikova, E. Vorontsov and V. Rozenberg, Tetrahedron: Asymmetry, 2008, 19, 216–222 CrossRef CAS;
(d) B. Jiang and X.-L. Zhao, Tetrahedron: Asymmetry, 2004, 15, 1141–1143 CrossRef CAS;
(e) P. G. Jones, J. Hillmer and H. Hopf, Acta Crystallogr., Sect. E: Struct. Rep. Online, 2003, 59, o24–o25 CAS;
(f) D. Pamperin, H. Hopf, C. Syldatk and M. Pietzsch, Tetrahedron: Asymmetry, 1997, 8, 319–325 CrossRef CAS;
(g) D. Pamperin, B. Ohse, H. Hopf and M. Pietzsch, J. Mol. Catal. B: Enzym., 1998, 5, 317–319 CrossRef CAS;
(h) D. C. Braddock, I. D. MacGilp and B. G. Perry, J. Org. Chem., 2002, 67, 8679–8681 CrossRef CAS PubMed;
(i) G. Meyer-Eppler, E. Vogelsang, C. Benkhäuser, A. Schneider, G. Schnakenburg and A. Lützen, Eur. J. Org. Chem., 2013, 4523–4532 CrossRef CAS.
-
(a) Y. Morisaki, M. Gon, T. Sasamori, N. Tokitoh and Y. Chujo, J. Am. Chem. Soc., 2014, 136, 3350–3353 CrossRef CAS PubMed;
(b) M. Gon, Y. Morisaki and Y. Chujo, J. Mater. Chem. C, 2015, 3, 521–529 RSC;
(c) Y. Morisaki, R. Sawada, M. Gon and Y. Chujo, Chem. – Asian J., 2016, 11, 2524–2527 CrossRef CAS PubMed.
- The optical resolution of rac-4,5,15,16-tetrasubstituted [2.2]paracyclophane was reported: N. V. Vorontsova, V. I. Rozenberg, E. V. Sergeeva, E. V. Vorontsov, Z. A. Starikova, K. A. Lyssenko and H. Hopf, Chem. – Eur. J., 2008, 14, 4600–4617 CrossRef CAS PubMed.
-
(a) M. Gon, Y. Morisaki and Y. Chujo, Eur. J. Org. Chem., 2015, 7756–7762 CrossRef CAS;
(b) M. Gon, Y. Morisaki, R. Sawada and Y. Chujo, Chem. – Eur. J., 2016, 22, 2291–2298 CrossRef CAS PubMed;
(c) M. Gon, Y. Morisaki and Y. Chujo, Chem. – Eur. J., 2017, 23, 6323–6329 CrossRef CAS PubMed.
-
(a) Y. Tohda, K. Sonogashira and N. Hagihara, Tetrahedron Lett., 1975, 16, 4467–4470 CrossRef;
(b)
K. Sonogashira, in Handbook of Organopalladium Chemistry for Organic Synthesis, ed. E. Negishi, Wiley-Interscience, New York, 2002, pp. 493–529 Search PubMed.
- Chemoselective Sonogashira–Hagihara coupling of 2 with arylacetylenes instead of trimethylsilyl acetylene is possible. In this study, trimethylsilyl acetylene was employed due to the easy removal of unreacted (excess amount) trimethylsilylacetylene and 1,4-bis(trimethylsilyl)-1,3-butadiyne as a byproduct by evaporation and column chromatography.
- For reviews, see:
(a) H. Maeda and Y. Bando, Pure Appl. Chem., 2013, 85, 1967–1978 CrossRef CAS;
(b) J. Kumar, T. Nakashima, H. Tsumatori and T. Kawai, J. Phys. Chem. Lett., 2014, 5, 316–321 CrossRef CAS PubMed;
(c) E. M. Sánchez-Carnereo, A. R. Agarrabeitia, F. Moreno, B. L. Maroto, G. Muller, M. J. Ortiz and S. de la Moya, Chem. – Eur. J., 2015, 21, 13488–13500 CrossRef PubMed;
(d) F. Zinna and L. Di Bari, Chirality, 2015, 27, 1–13 CrossRef CAS PubMed;
(e) G. Longhi, E. Castiglioni, J. Koshoubu, G. Mazzeo and S. Abbate, Chirality, 2016, 28, 696–707 CrossRef CAS PubMed.
- Very recently, excellent CPL-emitting organic molecules with large glum values on the order of 10−1 were reported. S. Sato, A. Yoshii, S. Takahashi, S. Furumi, M. Takeuchi and H. Isobe, Proc. Natl. Acad. Sci. U. S. A., 2017, 114, 13097–13101 CrossRef CAS PubMed.
Footnote |
† Electronic supplementary information (ESI) available: Experimental details including synthetic procedures, characterization, and NMR spectra. See DOI: 10.1039/c7qm00613f |
|
This journal is © the Partner Organisations 2018 |
Click here to see how this site uses Cookies. View our privacy policy here.