Synthesis and characterization of pyrrolyldipyrrin F-BODIPYs†
Received
8th September 2017
, Accepted 27th November 2017
First published on 8th December 2017
Abstract
A series of synthetic analogs of the tripyrrolic natural product prodigiosin were complexed with boron trifluoride to generate the corresponding F-BODIPYs. The maximum wavelengths of absorption and emission of the pyrrolyldipyrrin F-BODIPYs were tuned through variation of the substituents about the pyrrolyldipyrrinato core. The limited variation of substituents on the C-ring did not significantly affect absorption and emission. However, variation of substituents on the B-ring and A-ring resulted in a corresponding red-shift in absorption and emission reaching maximum wavelengths of 600 nm. The presence of electron donating substituents on the B-ring caused a decrease in the Stokes shift, while the presence of electron-withdrawing substituents caused an increase, ranging from 3–25 nm. Stokes shifts were solvent-dependant for some compounds. The inclusion of a dimethylamino group resulted in photo-induced electron transfer and thus quenched fluorescence which was restored upon protonation.
Introduction
Tripyrrolic compounds that contain a dipyrrin and a pyrrolic unit, connected at the 9-position, constitute what are known as pyrrolyldipyrrins. The presence of this third pyrrolic unit extends the π-conjugation of the dipyrrinato ligand and causes red-shifted absorption maxima that have facilitated applications in the life sciences and materials chemistry.1 The natural product prodigiosin and its synthetic analogues, termed prodigiosenes (Fig. 1), belong to the pyrrolyldipyrrin family2 and exhibit significant biological activity including immunosuppressive,3 anti-malaria,4 anti-microbial5 and anti-cancer activity.6,7
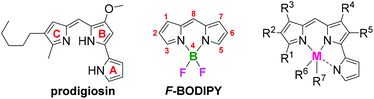 |
| Fig. 1 Prodigiosin, F-BODIPY and pyrrolyldipyrrin metal complexes. | |
Dipyrrins coordinate to a variety of metals1,8 but the most prominent type of dipyrrinato complex features a –BF2 unit to yield 4,4-difluoro-4-bora-3a,4a-diaza-s-indacenes (F-BODIPYs, Fig. 1). F-BODIPYs are chemically robust, as well as thermally, photochemically and physiologically stable. They exhibit sharp fluorescence, with high molar extinction coefficients and quantum yields, making them useful in applications such as cell imaging, chemosensors and laser dyes.9–12 Like dipyrrins, several metal complexes of pyrrolyldipyrrins have been reported, including those of cobalt, copper, tin, zinc and boron.4,13–17 Compared to boron complexes of dipyrrins, pyrrolyldipyrrinato complexes absorb and emit light at much longer wavelengths, due to the extended π-conjugation of the dipyrrinato ligand. As a result, these highly fluorescent complexes are useful as probes in biological applications, e.g., the commercially available fluorescent dyes BODIPY 576/589 and BODIPY 650/665 (absorption/emission, respectively).16,18–20 Other reported examples of boron difluoro pyrrolyldipyrrinato complexes include the unsubstituted pyrrolyldipyrrin20 and those with substituted aromatics,15,17,21–23 heterocycles22 and alkyl groups21 at the meso-position, phenyl substitution at the meso-position with different functional groups present on the A-ring24,25 or the presence of halogens about the pyrrolyldipyrrin core26,27 and isoindole substitution of the B-ring.16,28 Boron difluoro complexes of prodigiosenes (pyrrolyldipyrrins bearing a methoxy substituent on the B-ring) have recently been reported. However, the purpose of the synthesis was to afford the desired synthetic natural products in a facile manner by removal of the –BF2 protecting group.29 Additionally, our group has recently synthesized two pyrrolyldipyrrin BF2 complexes bearing ester functionality on the C-ring of the pyrrolyldipyrrin core.4,13,30 To the best of our knowledge these are the only reported examples of pyrrolyldipyrrin F-BODIPYs. Despite these examples, a thorough study investigating how changing the substituents about the three rings of the pyrrolyldipyrrin core affects the photophysical properties is lacking. Herein, a series of pyrrolyldipyrrin F-BODIPYs with varying substituents on each of the pyrrolic rings, and their respective photophysical properties, are described.
Results and discussion
To investigate the photophysical properties of prodigiosene-based F-BODIPYs, the pyrrolyldipyrrin HCl salts 1 bearing modifications to the A, B and C-rings were synthesized.4,6,31 Modifications included conjugated carbonyl and alkyl substituents on the C-ring, electron-withdrawing and electron-donating groups on the B-ring, and indole substitution at the A-ring. The pyrrolyldipyrrin HCl salts 1 were complexed with boron trifluoride in the presence of base.11,32 Several additions of Lewis acid and base, plus extended reaction times, were required to afford the eighteen novel pyrrolyldipyrrin F-BODIPYs 2–4 in 38–95% yields after multiple purification attempts via column chromatography (Scheme 1).
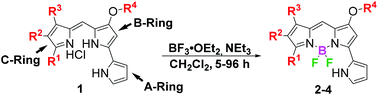 |
| Scheme 1 Synthesis of pyrrolyldipyrrin F-BODIPYs 2–4. | |
A crystal suitable for X-ray analysis of pyrrolyldipyrrin F-BODIPY 2d was obtained from the slow evaporation of a chloroform solution. The thermal ellipsoid diagram of 2d is shown in Fig. 3 and illustrates that the pyrrolyldipyrrin core is perpendicular to the plane of the coordinated F–B–F atoms. The B–N bond distances range from 1.54–1.56 Å indicating that the dipyrrinato unit is significantly resonance stabilized, as expected. The core structure of 2d is essentially planar, with the uncoordinated pyrrolic unit slightly out of plane, which may accommodate a hydrogen bond between the hydrogen atom of the pyrrolic nitrogen and a fluorine. The NH–F hydrogen bond distance ranges from 2.05–2.53 Å.
The pyrrolyldipyrrin F-BODIPYs featured a variety of substituents on the C-ring (2a–2l, Fig. 2) and B-ring (3m–3p, Fig. 4). In addition, two analogues 4q and 4r (Fig. 5) of Obatoclax,33 bearing an indole moiety in lieu of the A-ring, were prepared. F-BODIPY 2a (Fig. 2), the unsubstituted pyrrolyldipyrrin bearing only the signature methoxy group on the B-ring, was chosen as a parent compound to which the properties of the C-ring substituted pyrrolyldipyrrin F-BODIPYs would be compared. Such substituents included a mimic of the natural product prodigiosin (2b), conjugated esters (2c–2f, 2l), conjugated ketones (2g–2i) and derivatives of ethanoic acid (2j and 2k). These substituents provide a wide scope to demonstrate the influence that C-ring substituents have on the photophysical properties of pyrrolyldipyrrin F-BODIPYs.
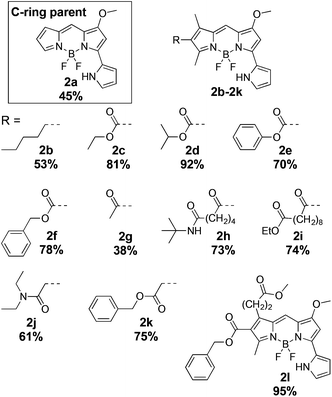 |
| Fig. 2 Pyrrolyldipyrrin F-BODIPYs (2a–2l) with C-ring modifications. | |
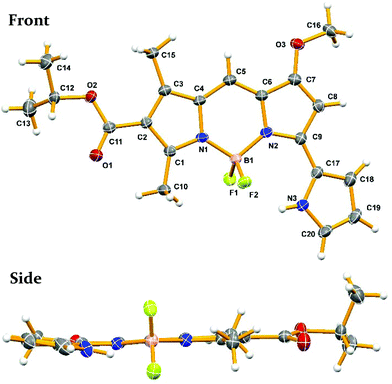 |
| Fig. 3 Thermal ellipsoid diagram of 2d (50%). Selected bond distances (Å): B(1)–N(1), 1.539(2); B(1)–N(2), 1.551(2); selected bond angles (°): N(1)–B(1)–N(2), 108.36(11); selected torsional angles (°): N(2)–C(9)–C(17)–N(3), −7.5(2); hydrogen bond distance (Å): N(3)–H(3N)⋯F(1), 2.529(17), N(3)–H(3N)⋯F(2), 2.049(18). | |
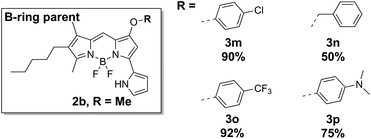 |
| Fig. 4 Pyrrolyldipyrrin F-BODIPYs (3m–3p) with B-ring modifications. | |
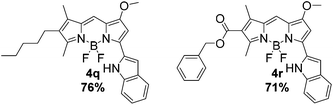 |
| Fig. 5 Pyrrolyldipyrrin F-BODIPYs (4q–4r) A-ring (indole) modifications. | |
The synthetic analog 2b of prodigiosin was selected as the parent compound by which substrates (3m–3p), bearing benzoxy or phenoxy substituents in lieu of the methoxy group, would be compared. In this regard, the effect that the methoxy substituent has upon the photophysical properties would be evaluated. The nature of this substituent is highly important to some aspects of the biological activity of prodigiosenes, yet substituting the methyl group for benzyl or phenyl groups does not diminish the anticancer properties of the compound.6 Lastly, pyrrolyldipyrrin F-BODIPYs 4q and 4r feature an indole moiety which further extends the π-conjugation of the F-BODIPY core and as a result are expected to inherently absorb and emit at longer wavelengths. Compounds 2b and 2f (Fig. 2) represent the parent compounds of the A-ring indole analogs, 4q and 4r.
The photophysical properties of solutions of pyrrolyldipyrrin F-BODIPYS (2–4) in CH2Cl2 were evaluated. All absorbance spectra exhibited a similar band structure in the visible region, with a peak and shoulder that are characteristic of BF2 dipyrrin complexes. Additionally, all compounds emitted at wavelengths in the yellow/orange visible region, displaying high extinction coefficients (11
000–156
000 mol M−1 cm−1) and have Stokes’ shift in the range of 3–25 nm. As representative examples, the absorbance and emission spectra of F-BODIPY 2a and 3o (Fig. 6) illustrate the increase in Stokes’ shift observed for electron-withdrawing groups of the B-ring compared to the unsubstituted pyrrolyldipyrrin. A summary of the results is presented in Table 1.
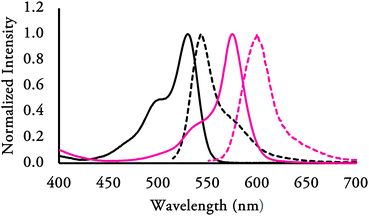 |
| Fig. 6 Normalized absorbance and emission spectra of 2a (left, black, Stokes shift 13 nm) and 3o (right, pink, Stokes shift 25 nm). | |
Table 1 Photophysical properties of F-BODIPYs 2–4 in CH2Cl2 at 22 °C
F-BODIPY |
λ
abs (nm) |
λ
em (nm) |
Stokes shift (nm) |
log ε |
Φ
F
|
Relative to Rhodamine 6G in EtOH (ΦF = 0.94).
Relative to Rhodamine 101 in EtOH (ΦF = 0.96).
Hexanes used instead of CH2Cl2 for quantum yield data.
|
2a
|
530 |
543 |
13 |
4.57 |
0.95b |
2b
|
565 |
578 |
13 |
4.69 |
0.84b |
2c 34 |
542 |
555 |
13 |
4.81 |
0.85a |
2d
|
543 |
555 |
12 |
4.83 |
0.90a |
2e
|
539 |
555 |
16 |
4.80 |
0.90a |
2f 4 |
542 |
553 |
11 |
5.20 |
1.00a |
2g
|
544 |
557 |
13 |
4.04 |
0.78a |
2h
|
545 |
557 |
12 |
4.85 |
0.85a |
2i
|
546 |
559 |
13 |
4.93 |
0.90a |
2j
|
561 |
574 |
13 |
4.65 |
0.81b |
2k
|
558 |
571 |
13 |
5.10 |
0.79b |
2l
|
540 |
559 |
19 |
4.74 |
0.70a |
3m
|
573 |
597 |
24 |
4.88 |
0.89b |
3n
|
566 |
572 |
6 |
4.74 |
0.98a |
3o
|
575 |
600 |
25 |
4.41 |
0.74b |
3p
|
567 |
570 |
3 |
4.69 |
0.55b,c |
4q
|
579 |
591 |
12 |
4.73 |
1.00b |
4r
|
562 |
574 |
12 |
4.87 |
0.95b |
To evaluate the effects of C-ring substituents on the absorption maxima, each compound was compared to the parent (2a) bearing only the methoxy substituent on the B-ring. F-BODIPY 2a itself has a maximum absorbance wavelength of 530 nm, which is hypsochromically shifted by 44 nm from the unsubstituted pyrrolyldipyrrin F-BODIPY.16 This indicates that the electron-donating methoxy group causes a blue-shift in absorption. All modifications to the C-ring (2b–2l) bathochromically shifted the absorbance wavelength (9–35 nm) compared to the unsubstituted, methoxy bearing F-BODIPY 2a (see ESI, Fig. S3 and S4†). The greatest shift in absorption wavelength maxima of the C-ring modified pyrrolyldipyrrin F-BODIPYs occurred with the alkyl and ethanoic acid derivative substituents: 2b, 2j and 2k bearing a –CH2CH3, –CH2C(O)NEt2 and –CH2CO2Bn group, respectively. This trend is in agreement with that observed for F-BODIPYs whereby as the core becomes more alkyl substituted, the fluorophore absorbs at a higher wavelength.
The presence of electron-withdrawing groups on the B-ring (–Ph-pCl, 3m or –Ph-pCF3, 3o) resulted in a slight red-shift in absorption and emission wavelengths when compared to the parent compound 2b (see ESI, Fig. S5 and S6†) A similar trend has been reported for dipyrrin F-BODIPYs that contain electron-withdrawing groups in the meso-position.35 Both F-BODIPYS 4q and 4r bearing the indole moiety in lieu of the A-ring pyrrole, exhibited significantly red-shifted absorbance maxima compared to the unsubstituted F-BODIPY 2a. Furthermore, 4q and 4r, the A-ring indole analogs of 2b and 2f, respectively, exhibited a red shift in absorption of 14–20 nm of their A-ring pyrrole analogues, courtesy of the indole moiety (see ESI, Fig. S7 and S8†).
Unlike their constituent ligands, pyrrolyldipyrrin F-BODIPYs (2–4) exhibited considerable fluorescence with excellent relative quantum yields (ΦF > 0.70, Table 1) with the exception of F-BODIPY 3p. Pyrrolyldipyrrin F-BODIPY 3p did not display fluorescence when dissolved in CH2Cl2 (Fig. 7a). However, when 3p was dissolved in hexanes, an immediate display of fluorescence was evident (Fig. 7b). The emission spectra of 3p in CH2Cl2 and hexanes is shown in ESI Fig. S9.† As well as a broader band structure for the emission of 3p in hexanes, compared to that of F-BODIPY 2a (see Fig. 6), 3p exhibited a very narrow Stokes’ shift of 3 nm and much lower quantum yield of 0.55.
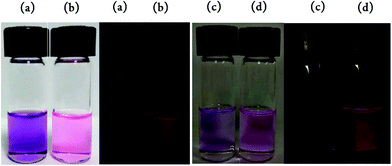 |
| Fig. 7
3p in CH2Cl2 (a), hexanes (b), CH2Cl2 without 1 M HCl (c) and CH2Cl2 with 1 M HCl (d) under ambient light and UV light. | |
The lack of emission of 3p in CH2Cl2 can be attributed to the lone pair of the amino substituent transferring an electron to the F-BODIPY fluorophore to quench its fluorescence through photo-induced electron transfer (PET).36,37 This process can be effectively controlled by variation of the polarity of the solvent or by protonation of the amino substituent. To investigate the PET process observed for 3p, a solution of this compound in hexanes was titrated with CH2Cl2 and indeed fluorescence quenching was observed (Fig. 8a). Treatment of a CH2Cl2 solution of 3p, using 5 μL of 1 M HCl, restored fluorescence (Fig. 7 and 8b). The maximum wavelength of emission for 3p in CH2Cl2 with the addition of 1 M HCl is 597 nm with a Stokes’ shift of 28 nm and a quantum yield of 0.41. The absorbance spectra of 3p in CH2Cl2 shows a red-shift in the maximum wavelength of absorption (569 nm–574 nm) upon addition of 1 M HCl (see ESI Fig. S10†).
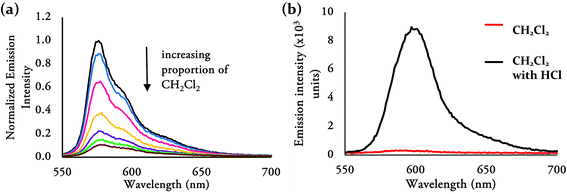 |
| Fig. 8 (a) Observed fluorescence quenching of 3p in hexanes via titration with CH2Cl2; (b) emission spectra of 3p dissolved in CH2Cl2 and in CH2Cl2 with addition of 1 M HCl. | |
Additionally, solvatochromatic effects of 2b (ESI Fig. S11 and S12†) and 3n–3p were observed in hexane, toluene, dichloromethane, tetrahydrofuran, acetonitrile and methanol and is summarized in the ESI (Table S1†). Interestingly, when 3p was dissolved in polar solvents such as tetrahydrofuran, acetonitrile and methanol, the Stokes’ shift was significantly increased from 3 nm (in hexanes) to 22–25 nm, yet with a lower quantum yield ranging from 0.22–0.30. Such an effect was not observed for 2b and 3m–3o, where the Stokes’ shift and relative quantum yield did not vary amongst the different solvents. A slight blue-shift in absorption and emission wavelengths was observed for 2b and 3m–3o when dissolved in polar solvents (acetonitrile and methanol) compared to the wavelengths observed for these compounds dissolved in CH2Cl2.
The solvatochromatic effect on the emission of 3p is illustrated by the Lippert–Mataga plot (Fig. 9) of solvent orientation polarizability (Δf) versus Stokes’ Shift (in cm−1). There is a linear correlation between the solvent polarity and magnitude of the Stokes’ Shift, with high polarity solvents exerting the greatest effect. It should be noted that Lippert–Mataga plots for compounds 2b and 3m–3o (ESI Fig. 13†) do not illustrate such correlation, alluding that these compounds are not as sensitive to solvent polarity as 3p. The larger Stokes’ shift observed for 3p dissolved in more polar solvents can be rationalized by considering the magnitude of the slope from the Lippert–Mataga plot, which gives an estimation of the change in dipole moment of the excited state and ground state of 3p. In general, polar species can orient around the dipole of the excited state and thereby lower the energy of the excited state and shift emission maxima to longer wavelengths.
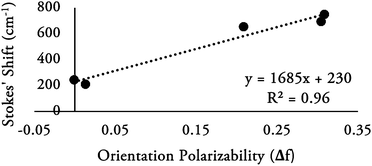 |
| Fig. 9 Lippert–Mataga plot of 3p illustrating the Stokes’ shift as a function of orientation polarizability (Δf). Solvents from left-right: hexane, toluene, tetrahydrofuran, acetonitrile and methanol. Dichloromethane is not represented on the plot as there was no measurable emission maximum for the compound in this solvent. | |
Conclusions
A series of pyrrolyldipyrrin F-BODIPYS with varying substituents on the A, B and C rings has been synthesized. Variation of substituents on the B-ring and A-ring result in the corresponding red-shift in absorption and emission reaching maximum emission wavelengths of 600 nm. Complexes bearing electron withdrawing substituents on the B-ring exhibited the largest Stokes’ shift of 24–25 nm, while complexes bearing electron donating groups on the B-ring displayed the smallest Stokes’ shift of only 3–6 nm. Extending the conjugation of the pyrrolyldipyrrin F-BODIPY core via placement of an indole substituent in lieu of the A-ring (4q and 4r), results in the exhibition of a red-shift in absorption and emission wavelength (562–579 nm) with Stokes’ shift comparable to that of C-ring substituents. Pyrrolyldipyrrin F-BOIPY 3p exhibited interesting photophysical properties where the fluorescence is controlled depending on the polarity of the solvent. In hexanes, 3p displays appreciable fluorescence, while in CH2Cl2 the fluorescence is effectively quenched. Additionally, the small Stokes’ shift observed for 3p could be enhanced when dissolved in polar solvents such as tetrahydrofuran, acetonitrile and methanol, or upon protonation with HCl. These data regarding the effects of substitution upon absorption and emission provide insight regarding how pyrrolyldipyrrin F-BODIPYs can be designed to match performance criteria.
Experimental procedures
General experimental
All chemicals, including anhydrous solvents and reagents, were obtained from commercial sources and used as received unless otherwise noted. CH2Cl2 and hexanes for chromatography were obtained crude and distilled under air at 1 atm pressure before use. Column chromatography was performed using 150 mesh Brockman III, basic or neutral aluminum oxide. NMR spectra were obtained using a 500 MHz or 300 MHz instrument using CDCl3 as solvent. Chemical shifts are reported in parts per million using the solvent signals as an internal 1H and 13C references. 11B and 19F chemical shifts were referenced using BF3·Et2O (15% in CDCl3) and CCl3F defining the 0 ppm position as per the absolute referencing procedure standard for Bruker digital spectrometers. Coupling constants (J) are reported in hertz. Mass spectra were recorded using ESI TOF ionization. UV-Vis and emission analyses were performed using a UV-visible spectrophotometer and PTI spectrofluorometer, respectively. X-ray crystallography measurements were made on a CCD-equipped diffractometer (30 mA, 50 kV) using monochromated Mo Kα radiation (λ = 0.71073 Å) at 125 K. Compounds 1a–1r were prepared according to literature procedures.4,6,31
General procedure for absorbance and emission measurements
In all measurements, a 10 mm quartz cuvette was used for solutions. For fluorescence experiments, a slit width of 3 nm was used for both excitation and emission.
Fluorescence quantum yield
Relative fluorescence quantum yields were obtained by comparing the area under the emission spectrum of the compound of interest to that of the standard, rhodamine 6G (ϕ = 0.94 in ethanol) or rhodamine 101 (ϕ = 0.96 in ethanol).38 The excitation wavelength was 520 nm for rhodamine 6G and 546 nm for rhodamine 101. The excitation wavelength was 520 nm for 2c–2i, 2l and 3n and compared to rhodamine 6G excited at 520 nm. The excitation wavelength for 2a–2b, 2j–2k, 3m, 3o–3p and 4q–4r was 546 nm and compared to rhodamine 101 excited at 546 nm. Relative quantum yields were determined using eqn (1),39 where Φst is the reported quantum yield of the standard, I is the area of the integrated emission spectra, A is the absorbance at the excitation wavelength and η is the refractive index of the solvent used. The subscripts “X” and “st” denote the unknown and standard, respectively.
Relative quantum yield (ΦX):
| 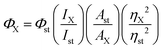 | (1) |
General procedure (GP1) for BF2 complexation of pyrrolyldipyrrins
The corresponding pyrrolyldipyrrin HCl salt (1 equiv.) was dissolved in dry CH2Cl2 under a nitrogen atmosphere. Triethylamine (6 equiv.) was added and the reaction mixture then stirred for 10 minutes. BF3·OEt2 (9 equiv.) was then added slowly, over a period of a few minutes. The reaction mixture was stirred until complete loss of starting material according to analysis using thin layer chromatography. Subsequent additions of base (6 equiv.) and BF3·OEt2 (9 equiv.) were added after a period of 8 hours. Reaction time and total amount of base and BF3·OEt2 varied (5 h–96 h) depending upon the substrate. Upon completion, the reaction mixture was quenched via addition to 1 M HCl (15 mL) and then extracted with CH2Cl2 (25 mL). The organic phase was washed with 1 M HCl (1 × 15 mL) and brine (2 × 15 mL), dried over anhydrous sodium sulfate and concentrated in vacuo. The resulting crude material was purified via column chromatography on basic or neutral alumina eluting with 5–20% ethyl acetate in hexanes, unless otherwise stated.
Boron, difluoro[[(2H-pyrrol-2-ylidene)methyl]-4-methoxy-1H,1′H-2,2′-bipyrrole] (2a).
Following GP1, over a 72 hour period a solution of 1a (20 mg, 0.07 mmol) in CH2Cl2 (4 mL) was reacted with BF3·OEt2 (233 μL, 1.89 mmol) and NEt3 (176 μL, 1.26 mmol). The product was purified using column chromatography on basic alumina, eluting with 10–25% CH2Cl2 in hexanes, to provide F-BODIPY 2a (9 mg, 45% yield). 1H NMR (500 MHz, CDCl3) 10.61 (br s, 1H), 7.51 (s, 1H), 7.20–7.18 (m, 1H), 7.13 (s, 1H), 7.01–6.99 (m, 1H), 6.77–6.76 (m, 1H), 6.42–6.37 (m, 2H), 6.12 (s, 1H), 3.98 (s, 3H); 13C NMR (CDCl3, 125 MHz) 165.3, 152.7, 134.1, 131.3, 130.5, 126.9, 123.5, 121.7, 119.2, 117.8, 114.9, 111.8, 97.1, 58.8; 11B NMR (CDCl3, 160 MHz) 1.23 (t, J = 35 Hz); 19F NMR (235 MHz, CDCl3) −139 (q, J = 34 Hz); UV/vis (CH2Cl2) λmax (nm) 530, ε 37
000 mol L−1 cm−1; fluorescence (CH2Cl2) λex (nm), 543, ΦF: 0.95; LRMS: 310.1 (M + Na)+; HRMS: 310.0925. Found, 310.0934 calculated for C14H12BF2N3NaO.
Boron, difluoro[5-[(3,5-dimethyl-4-pentyl-2H-pyrrol-2ylidene)methyl]-4-methoxy-1H,1′H-2,2′-bipyrrole] (2b).
Following GP1, over a 4 day period a solution of 1b (18 mg, 0.05 mmol) in CH2Cl2 (4 mL) was reacted with BF3·OEt2 (278 μL, 2.25 mmol) and NEt3 (167 μL, 1.20 mmol). The product was purified using column chromatography on neutral alumina, eluting with 1%, 3%, 5% and then 10% ethyl acetate in hexanes, to provide F-BODIPY 2b (10 mg, 53% yield). M.p. 124–126 °C; 1H NMR (500 MHz, CDCl3) 10.43 (br s, 1H), 7.08–7.07 (m, 1H), 7.05 (s, 1H), 6.84 (m, 1H), 6.33–6.31 (m, 1H), 6.10 (s, 1H), 3.95 (s, 3H), 2.49 (s, 3H), 2.37 (t, J = 7.7 Hz, 2H), 2.15 (s, 3H), 1.48–1.41 (m, 2H), 1.37–1.30 (m, 4H), 0.91 (t, J = 7.0 Hz, 3H); 13C NMR (125 MHz, CDCl3) 162.6, 150.2, 147.8, 134.3, 130.7, 129.5, 126.2, 124.2, 124.1, 115.6, 114.8, 110.8, 96.5, 58.4, 31.9, 30.2, 24.3, 22.7, 14.2, 12.6, 9.7; 11B NMR (160 MHz, CDCl3) 1.41 (t, J = 36 Hz); 19F NMR (235 MHz, CDCl3) −139 (q, J = 33 Hz); UV/vis (CH2Cl2) λmax (nm): 565, ε 49
000 mol L−1 cm−1; fluorescence (CH2Cl2) λexci, 520 (nm); λmax (nm), 578, ΦF: 0.84; LRMS: 408.2 (M + Na)+; HRMS: 408.2031. Found, 408.2029 calculated for C21H26BF2N3NaO.
Boron, difluoro[ethyl 2-[(4-methoxy-1H,1′H-2,2′-bipyrrol-5-yl)-methylene]-3,5-dimethyl-2H-pyrrole-4-carboxylate] (2c)13.
Following GP1, over a 24 hour period a solution of 1c (50 mg, 0.13 mmol) in CH2Cl2 (6 mL) was reacted with BF3·OEt2 (610 μL, 4.94 mmol) and NEt3 (544 μL, 3.90 mmol). The reaction mixture was quenched via addition of 5% citric acid and extracted with diethyl ether (20 mL). The product was purified using column chromatography on neutral alumina, eluting with 75% CH2Cl2 in hexanes, to provide F-BODIPY 2c (42 mg, 81% yield). 1H NMR (500 MHz, CDCl3) 10.50 (br s, 1H), 7.19–7.18 (m, 1H), 7.17 (s, 1H), 6.97–6.96 (m, 1H), 6.39–6.37 (m, 1H), 6.14 (s, 1H), 4.31 (q, J = 7.1 Hz, 2H), 3.99 (s, 3H), 2.81 (s, 3H), 2.43 (s, 3H), 1.37 (t, J = 7.1 Hz, 3H); 11B NMR (160 MHz, CDCl3) 1.39 (t, J = 36 Hz); 1HNMR and 11B NMR chemical shifts were in agreement with literature values.13 UV/vis (CH2Cl2) λmax (nm): 542, ε 64
000 mol L−1cm−1; fluorescence (CH2Cl2) λexci, 520 (nm); λmax (nm), 555, ΦF: 0.85.
Boron, difluoro[isopropyl 2-[(4-methoxy-1H,1′H-2,2′-bipyrrol-5-yl)-methylene]-3,5-dimethyl-2H-pyrrole-4-carboxylate] (2d).
Following GP1, over a 72 hour period a solution of 1d (23 mg, 0.08 mmol) in CH2Cl2 (4 mL) was reacted with BF3·OEt2 (133 μL, 1.08 mmol) and NEt3 (100 μL, 0.72 mmol). The product was purified using column chromatography on neutral alumina, eluting with 5%, 10% and then 25% ethyl acetate in hexanes, to provide F-BODIPY 2d (22 mg, 92% yield). M.p. 191–193 °C; 1H NMR (300 MHz, CDCl3) 10.50 (br s, 1H), 7.18–7.16 (m, 1H), 7.15 (s, 1H), 6.97–6.94 (m, 1H), 6.38–6.36 (m, 1H), 6.12 (s, 1H), 5.21 (sep, J = 6.2 Hz, 1H), 3.97 (s, 3H), 2.80 (s, 3H), 2.41 (s, 3H), 1.35 (d, J = 6.3 Hz, 6H); 13C NMR (CDCl3, 125 MHz): 165.0, 164.4, 153.0, 151.4, 136.7, 129.5, 129.4, 126.4, 123.7, 118.6, 118.3, 115.1, 111.8, 97.3, 67.1, 58.8, 22.5, 14.5, 12.0; 11B NMR (160 MHz, CDCl3) 1.38 (t, J = 36 Hz); 19F NMR (235 MHz, CDCl3) −138 (q, J = 34 Hz); UV/vis (CH2Cl2) λmax (nm): 543, ε 68
000 mol L−1 cm−1; fluorescence (CH2Cl2) λexci, 520 (nm); λmax (nm), 555, ΦF: 0.90; LRMS: 424.2 (M + Na)+; HRMS: 424.1607. Found, 424.1614 calculated for C20H22BF2N3NaO3.
Boron, difluoro[phenyl 2-[(4-methoxy-1H,1′H-2,2′-bipyrrol-5-yl)-methylene]-3,5-dimethyl-2H-pyrrole-4-carboxylate] (2e).
Following GP1, over a 24 hour period a solution of 1e (50 mg, 0.12 mmol) in CH2Cl2 (6 mL) was reacted with BF3·OEt2 (267 μL, 2.16 mmol) and NEt3 (201 μL, 1.44 mmol). The product was purified using column chromatography on neutral alumina, eluting with 60% CH2Cl2 in hexanes, to provide F-BODIPY 2e (37 mg, 70% yield). M.p. 248–250 °C; 1H NMR (500 MHz, CDCl3) 10.52 (br s, 1H), 7.41 (t, J = 7.93 Hz, 2H), 7.25–7.23 (m, 1H), 7.21–7.20 (m, 3H), 7.19 (s, 1H), 7.01–7.00 (m, 1H), 6.40–6.39 (m, 1H), 6.17 (s, 1H), 4.01 (s, 3H), 2.88 (s, 3H), 2.52 (s, 3H); 13C NMR (125 MHz, CDCl3) 164.6, 163.6, 153.4, 152.0, 150.9, 136.4, 129.9, 129.6, 126.8, 125.6, 123.5, 122.2, 119.1, 114.8, 111.9, 97.4, 58.8, 14.6, 12.0; 11B NMR (160 Hz, CDCl3) 1.41 (t, J = 36 Hz); 19F NMR (235 Hz, CDCl3) −138 (q, 34 Hz); UV/vis (CH2Cl2) λmax (nm): 539, ε 63
000 mol L−1 cm−1; fluorescence (CH2Cl2) λexci, 520 (nm); λmax (nm), 555, ΦF: 0.90; LRMS: 458.1 (M + Na)+; HRMS: 458.1459. Found, 458.1458 calculated for C23H20BF2N3NaO3.
Boron, difluoro[benzyl 2-[(4-methoxy-1H,1′H-2,2′-bipyrrol-5-yl)-methylene]-3,5-dimethyl-2H-pyrrole-4-carboxylate] (2f).
Following GP1, over a 24 hour period a solution of 1f (22 mg, 0.05 mmol) in CH2Cl2 (8 mL) was reacted with BF3·OEt2 (56 μL, 0.45 mmol) and NEt3 (42 μL, 0.30 mmol). The product was purified using column chromatography on neutral alumina, eluting with 25% CH2Cl2 in hexanes, to provide F-BODIPY 2f (6 mg, 26% yield). 1H NMR (500 MHz, CDCl3) 10.49 (br s, 1H), 7.45–7.34 (m, 1H), 7.20–7.17 (m, 1H), 7.16 (s, 1H), 6.99–6.96 (m, 1H), 6.40–6.36 (m, 1H), 6.14 (s, 1H), 5.32 (s, 2H), 3.99 (s, 3H), 2.81 (s, 3H), 2.42 (s, 3H); 11B NMR (160 MHz, CDCl3) 1.35 (t, J = 36 Hz). 1H and 11B NMR chemical shifts were in agreement with literature values.40 UV/vis (CH2Cl2) λmax (nm): 542, ε 156
000 mol L−1 cm−1; fluorescence (CH2Cl2) λexci, 520 (nm); λmax (nm), 553, ΦF: 1.00.
Boron, difluoro[1-[2-[(4-methoxy-1H,1′H-2,2′-bipyrrol-5-yl)methylene]-3,5-dimethyl-2H-pyrrol-4-yl]ethanone] (2g).
Following GP1, over a 48 hour period a solution of 1g (13 mg, 0.04 mmol) in CH2Cl2 (4 mL) was reacted with BF3·OEt2 (99 μL, 0.80 mmol) and NEt3 (84 μL, 0.60 mmol). The product was purified using column chromatography on basic alumina, eluting with 25% ethyl acetate in hexanes, F-BODIPY 2g (5 mg, 38% yield). 1H NMR (500 MHz, CDCl3) 10.50 (br s, 1H), 7.20 (s, 1H), 7.18 (s, 1H), 6.99 (s, 1H), 6.39 (s, 1H), 6.15 (s, 1H), 4.00 (s, 3H), 2.80 (s, 3H), 2.48 (s, 3H), 2.44 (s 3H); 13C NMR (125 MHz, CDCl3) 195.4, 164.4, 152.1, 151.8, 134.3, 129.5, 127.2, 126.7, 123.5, 118.9, 115.0, 114.8, 111.8, 97.3, 58.8, 31.7, 15.2, 12.5; 11B NMR (160 MHz, CDCl3) 1.41 (t, J = 36 Hz); 19F NMR (235 MHz, CDCl3) −138 (q, J = 29 Hz); UV/vis (CH2Cl2) λmax (nm): 544, ε 11
000 mol L−1 cm−1; fluorescence (CH2Cl2) λexci, 520 (nm); λmax (nm), 557, ΦF: 0.78; LRMS: 380.1 (M + Na)+; HRMS: 380.1363. Found, 380.1352 calculated for C18H18BF2N3NaO2.
Boron, difluoro[N-tert-butyl-6-[2-[(4-methoxy-1H,1′H-2,2′-bipyrrol-5-yl)methylene]-3,5-dimethyl-2H-pyrrol-4-yl]-6-oxohexanamide] (2h).
Following GP1, over a 60 hour period a solution of 1h (21 mg, 0.04 mmol) in CH2Cl2 (3 mL) was reacted with BF3·OEt2 (74 μL, 0.60 mmol) and NEt3 (50 μL, 0.36 mmol). The product was purified using column chromatography on neutral alumina, eluting with 40% ethyl acetate in hexanes, to provide F-BODIPY 2h (16 mg, 73% yield). M.p. 214–216 °C; 1H NMR (500 MHz, CDCl3) 10.49 (s, 1H), 7.19–718 (m, 1H), 7.16 (s, 1H), 7.00–6.97 (m, 1H), 6.39–6.37 (m, 1H), 6.14 (s, 1H), 5.44 (br s, 1H), 3.99 (s, 3H), 2.79–2.76 (m, 5H, overlapping CH3 and CH2), 2.42 (s, 3H), 2.15 (t, J = 7.0 Hz, 2H), 1.73–1.68 (m, 4H), 1.35 (s, 9H); 13C NMR (125 MHz, CDCl3) 197.9, 172.2, 164.4, 151.9, 151.7, 133.8, 129.7, 129.5, 127.3, 126.6, 123.5, 118.9, 114.8, 111.8, 97.3, 58.8, 51.3, 42.9, 37.8, 29.0, 25.6, 23.8, 15.2, 12.6; 11B NMR (160 MHz, CDCl3) 1.41 (t, J = 32 Hz); 19F NMR (235 MHz, CDCl3) −138 (q, J = 34 Hz); UV/vis (CH2Cl2) λmax (nm): 545, ε 71
000 mol L−1 cm−1; fluorescence (CH2Cl2) λexci, 520 (nm); λmax (nm), 557, ΦF: 0.85; LRMS: 521.3 (M + Na)+; HRMS: 521.2517. Found, 521.2506 calculated for C26H33BF2N4NaO3.
Boron, difluoro[ethyl 10-[2-[(4-methoxy-1H,1′H-2,2′-bipyrrol-5-yl)methylene]-3,5-dimethyl-2H-pyrrol-4-yl]-10-oxodecanoate] (2i).
Following GP1, over a 72 hour period a solution of 1i (24 mg, 0.05 mmol) in CH2Cl2 (4 mL) was reacted with BF3·OEt2 (111 μL, 0.90 mmol) and NEt3 (77 μL, 0.55 mmol). The product was purified using column chromatography on basic alumina, flushing first with 100% hexanes and then eluting compound with CH2Cl2, to provide F-BODIPY 2i (20 mg, 74% yield) M.p. 138–140 °C; 1H NMR (300 MHz, CDCl3): 10.50 (br s, 1H), 7.19 (s, 1H), 7.16 (s, 1H), 6.98 (s, 1H), 6.38 (s, 1H), 6.14 (s, 1H), 4.12 (q, J = 7.1 Hz, 2H), 3.98 (s, 3H), 2.78 (s, 3H), 2.74 (t, J = 7.4 Hz, 2H), 2.42 (s, 3H), 2.28 (t, J = 7.6 Hz, 2H), 1.72–1.54 (m, 4H), 1.33 (s, 8H), 1.25 (t, J = 7.1 Hz, 3H); 13C NMR (125 MHz, CDCl3) 198.5, 174.1, 164.4, 151.9, 151.6, 134.0, 129.7, 129.6, 127.6, 126.6, 123.6, 118.8, 114.9, 111.8, 97.3, 60.3, 58.8, 43.3, 34.6, 29.6, 29.4, 29.3, 25.2, 24.5, 15.2, 14.5, 12.5; 11B NMR (160 MHz, CDCl3) 1.41 (t, J = 30 Hz); 19F NMR (235 MHz, CDCl3) −138, J = 36 Hz; UV/vis (CH2Cl2) λmax (nm): 546, ε 85
000 mol L−1 cm−1; fluorescence (CH2Cl2) λexci, 520 (nm); λmax (nm), 559, ΦF: 0.90; LRMS: 550.3 (M + Na)+; HRMS: 550.2661. Found, 550.2659 calculated for C28H36BF2N3NaO4.
Boron, difluoro[N,N-diethyl-2-[2-[(4-methoxy-1H,1′H-2,2′-bipyrrol-5-yl)methylene]-3,5-dimethyl-2H-pyrrol-4-yl]acetamide] (2j).
Following GP1, over a 72 hour period a solution of 1j (17 mg, 0.04 mmol) in CH2Cl2 (4 mL) was reacted with BF3·OEt2 (89 μL, 0.72 mmol) and NEt3 (67 μL, 0.48 mmol). The product was purified using column chromatography on basic alumina, eluting with 25% and then 50% ethyl acetate in hexanes, to provide F-BODIPY 2j (11 mg, 61% yield). 1H NMR (500 MHz, CDCl3) 10.44 (br s, 1H), 7.09 (s, 1H), 7.07 (s, 1H), 6.86 (s, 1H), 6.33 (s, 1H), 6.10 (s, 1H), 3.95 (s, 3H), 3.44 (s, 2H), 3.37 (dq, 22.4, 7.2 Hz, 4H), 2.49 (s, 3H), 2.17 (s, 3H), 1.14 (dt, J = 10.7, 7.1 Hz, 6H); 13C NMR (CDCl3, 125 MHz) 169.3, 162.9, 149.1, 148.4, 134.4, 130.1, 126.7, 124.4, 123.8, 121.8, 116.1, 114.7, 110.8, 96.4, 58.2, 42.1, 40.4, 30.1, 14.1, 13.0, 12.5, 9.7; 11B NMR (160 MHz, CDCl3) 1.40 (t, J = 36 Hz); 19F NMR (235 MHz, CDCl3) −139 (q, J = 34 Hz) UV/vis (CH2Cl2) λmax (nm): 561, ε 45
000 mol L−1 cm−1; fluorescence (CH2Cl2) λexci, 546 (nm); λmax (nm), 574, ΦF: 0.81; LRMS: 451.2 (M + Na)+; HRMS: 451.2081. Found, 451.2087 calculated for C22H27BF2N4NaO2.
Boron, difluoro[benzyl 2-[2-[(4-methoxy-1H,1′H-2,2′-bipyrrol-5-yl)methylene]-3,5-dimethyl-2H-pyrrol-4-yl]acetate] (2k).
Following GP1, over a 48 hour period a solution of 1k (12 mg, 0.03 mmol) in CH2Cl2 (4 mL) was reacted with BF3·OEt2 (100 μL, 0.81 mmol) and NEt3 (75 μL, 0.54 mmol). The product was purified using column chromatography on basic alumina, eluting with CH2Cl2, to provide F-BODIPY 2k (9 mg, 75% yield) 1H NMR (500 MHz, CDCl3): 10.46 (br s, 1H), 7.35–7.30 (m, 5H), 7.11–7.10 (m, 1H), 7.08 (s, 1H), 6.89–6.88 (m, 1H), 6.35–6.33 (m, 1H), 6.11 (s, 1H), 5.13 (s, 2H), 3.96 (s, 3H), 3.46 (s, 2H), 2.49 (s, 3H), 2.17 (s, 3H); 13C NMR (125 MHz, CDCl3) 171.0, 163.3, 149.1, 136.0, 135.3, 134.5, 131.0, 128.7, 128.2, 127.2, 124.9, 124.0, 120.5, 116.7, 115.0, 111.1, 106.1, 96.7, 66.8, 58.5, 30.6, 12.6, 9.8; 11B NMR (160 MHz, CDCl3) 1.39 (J = 36 Hz); 19F (235 MHz, CDCl3) −139 (J = 35 Hz); UV/vis (CH2Cl2) λmax (nm): 558, ε 127
000 mol L−1 cm−1; fluorescence (CH2Cl2) λexci, 546 (nm); λmax (nm), 571, ΦF: 0.79; LRMS: 486.2 (M + Na)+; HRMS: 486.1778. Found, 486.1771 calculated for C25H24BF2N3NaO3.
Boron, difluoro[benzyl 2[(4-methoxy-1H,1′H-2,2′-bipyrrol-5-yl)methylene]-3-(3-methoxy-3-oxopropyl)-5-methyl-2H-pyrrole-4-carboxylate] (2l).
Following GP1, over a 48 hour period a solution of 1l (19 mg, 0.04 mmol) in CH2Cl2 (4 mL) was reacted with BF3·OEt2 (89 μL, 0.72 mmol) and NEt3 (67 μL, 0.48 mmol). The product was purified using column chromatography on basic alumina, eluting with 2%, 4%, 8%, 10% and then 25% ethyl acetate in hexanes, to provide F-BODIPY 2l (18 mg, 95% yield). 1H NMR (300 MHz, CDCl3): 10.50 (s, 1H), 7.44–7.32 (m, 5H), 7.23–7.17(m, 2H), 6.99 (s, 1H), 6.38 (s, 1H), 6.12 (s, 1H), 5.30 (s, 2H), 3.98 (s, 3H), 3.61 (s, 3H), 3.12 (t, J = 7.5 Hz, 2H), 2.80 (s, 3H), 2.56 (t, J = 7.4 Hz, 2H); 13C NMR (125 MHz, CDCl3) 173.4, 164.7, 152.7, 152.3, 137.6, 136.5, 130.3, 129.0, 128.7, 128.5, 128.2, 127.0, 123.4, 119.3, 114.9, 111.9, 97.4, 65.9, 58.8, 51.7, 35.4, 21.3, 14.6; 11B NMR (160 MHz, CDCl3) 1.34 (J = 36 Hz); 19F NMR (235 MHz, CDCl3) −138 (J = 35 Hz); UV/vis (CH2Cl2) λmax (nm): 540, ε 55
000 mol L−1 cm−1; fluorescence (CH2Cl2) λexci, 520 (nm); λmax (nm), 559, ΦF: 0.70; LRMS: 544.2 (M + Na)+; HRMS: 544.1825. Found, 544.1826 calculated for C27H26BF2N3NaO5.
Boron, difluoro[4-(4-chlorophenoxy)-5-[(3,5-dimethyl-4-pentyl-2H-pyrrol-2-ylidene)methyl]-1H,1′H-2,2′-bipyrrole] (3m).
Following GP1, over a 72 hour period a solution of 1m (20 mg, 0.04 mmol) in CH2Cl2 (4 mL) was reacted with BF3·OEt2 (148 μL, 1.2 mmol) and NEt3 (109 μL, 0.78 mmol). The product was purified using column chromatography on basic alumina, eluting with 20% CH2Cl2 in hexanes, to provide F-BODIPY 3m (18 mg, 90% yield). M.p. °C 135–137 °C; 1H NMR (500 MHz, CDCl3) 10.35 (br s, 1H), 7.40–7.38 (m, 2H), 7.19–7.16 (m, 3H), 7.07–7.05 (m, 1H), 6.70–6.68 (m, 1H), 6.29–6.27 (m, 1H), 5.98 (s, 1H), 2.53 (s, 3H), 2.38 (t, J = 7.7 Hz, 2H), 2.18 (s, 3H), 1.46–1.41 (m, 2H), 1.35–1.30 (m, 4H), 0.91 (t, J = 6.9 Hz, 3H); 13C NMR (125 MHz, CDCl3) 158.3, 154.7, 153.3, 146.0, 136.2, 131.7, 130.7, 130.2, 130.1, 125.9, 124.1, 124.0, 120.9, 115.5, 115.4, 110.8, 100.4, 31.8, 30.1, 24.2, 22.7, 14.3, 12.9, 9.8; 11B NMR (CDCl3, 160 MHz) 1.37 (J = 31 Hz); 19F NMR (235 MHz, CDCl3) −140 (J = 33 Hz); UV-vis (CH2Cl2) λmax (nm): 573, ε 75
000 mol L−1 cm−1; fluorescence (CH2Cl2) λexci, 546 (nm); λmax (nm), 597, ΦF: 0.89; LRMS: 504.2 (M + Na)+; HRMS: 504.1793. Found, 504.1796 calculated for C26H27BClF2N3NaO.
Boron, difluoro[4-(benzyloxy)-5-[(3,5-dimethyl-4-pentyl-2H-pyrrol-2-ylidene)methyl]-1H,1′H-2,2′-bipyrrole] (3n).
Following GP1, over a 96 hour period a solution of 1n (16 mg, 0.04 mmol) in CH2Cl2 (8 mL) was reacted with BF3·OEt2 (160 μL, 1.3 mmol) and NEt3 (120 μL, 0.86 mmol). The product was purified using column chromatography on basic alumina, eluting with 2%, 4%, 6% and then 8% ethyl acetate in hexanes, to provide F-BODIPY 3n (9 mg, 50% yield). 1H NMR (500 MHz, CDCl3) 10.42 (br s, 1H), 7.46–7.38 (m, 5H), 7.10 (s, 1H), 7.07 (s, 1H), 6.81 (s, 1H), 6.33–6.31 (m, 1H), 6.14 (s, 1H), 5.16 (s, 2H), 2.49 (s, 3H), 2.36 (t, 2H), 2.14 (s, 3H), 1.48–1.40 (m, 2H), 1.37–1.29 (m, 4H), 0.90 (t, 3H); 13C NMR (126 MHz, CDCl3) 161.4, 156.5, 147.6, 135.7, 134.6, 130.8, 129.6, 128.9, 128.8, 128.0, 126.4, 124.2, 124.1, 115.6, 115.0, 110.8, 97.4, 73.2, 31.9, 30.2, 24.3, 22.7, 14.2, 12.7, 9.7; 11B NMR (160 MHz, CDCl3) 1.41 (J = 36 Hz); 19F NMR (235 MHz, CDCl3) −139 (J = 34 Hz); UV/vis (CH2Cl2) λmax (nm): 566, ε 55
000 mol L−1 cm−1; fluorescence (CH2Cl2) λexci, 520 (nm); λmax (nm), 572, ΦF: 0.98. LRMS: 484.2 (M + Na)+; HRMS: 484.2339. Found, 484.2342 calculated for C27H30BF2N3NaO.
Boron, difluoro[5-[(3,5-dimethyl-4-pentyl-2H-pyrrol-2-ylidene)methyl]-4-[4-(trifluoromethyl)phenoxy]-1H,1′H-2,2′-bipyrrole] (3o).
Following GP1, over a 48 hour period a solution of 1o (12 mg, 0.02 mmol) in CH2Cl2 (8 mL) was reacted with BF3·OEt2 (54 μL, 0.44 mmol) and NEt3 (42 μL, 0.30 mmol). The product was purified using column chromatography on basic alumina, eluting with 5% ethyl acetate in hexanes, to provide F-BODIPY 3o (12 mg, 92% yield). 1H NMR (500 MHz, CDCl3) 10.34 (br s, 1H), 7.68 (d, J = 8.7 Hz, 2 H), 7.32 (J = 8.6 Hz, 2H), 7.13 (s, 1H), 7.07–7.05 (m, 1H), 6.72–6.70 (m, 1H), 6.30–6.28 (m, 1H), 6.11 (s, 1H), 2.54 (s, 3H), 2.39 (t, J = 7.7, 2H), 2.18 (s, 1H), 1.49–1.41 (m, 2H), 1.38–1.31 (m, 4H), 0.91 (t, J = 6.8 Hz, 3H); 13C NMR (126 MHz, CDCl3,) 159.2, 154.5, 145.8, 136.8, 131.2, 131.0, 129.0, 127.6, 126.1, 124.2, 124.0, 119.0, 115.7, 115.4, 110.9, 110.1, 101.6, 68.3, 31.9, 30.0, 24.3, 22.7, 14.2, 13.0, 9.8; 11B NMR (160 MHz, CDCl3) 1.27 (J = 35 Hz); 19F NMR (235 MHz, CDCl3) −62 (s, CF3), −140 (q, J = 35 Hz); UV/vis (CH2Cl2) λmax (nm): 575, ε 26
000 mol L−1 cm−1; fluorescence (CH2Cl2) λexci, 546 (nm); λmax (nm), 600, ΦF: 0.74. LRMS: 516.2 (M + Na)+; HRMS: 516.2262. Found, 516.2240 calculated for C27H28BF5N3NaO.
Boron, difluoro[4-[5-((3,5-dimethyl-4-pentyl-2H-pyrrol-2-ylidene)methyl)-1H,1′H-2,2′-bipyrrol4-yloxy]-N,N-dimethylaniline] (3p).
Following GP1, over a 48 hour period a solution of 1p (16 mg, 0.03 mmol) in CH2Cl2 (8 mL) was reacted with BF3·OEt2 (67 μL, 0.54 mmol) and NEt3 (50 μL, 0.36 mmol). The product was purified using column chromatography on basic alumina, eluting with 2%, 4%, 6%, and then 8% ethyl acetate in hexanes, to provide F-BODIPY 3p (12 mg, 75% yield). 1H NMR (500 MHz, CDCl3) 10.38 (br s, 1H), 7.21 (s, 1H), 7.10 (d, J = 9.0 Hz, 2H), 7.04 (t, J = 1.8 Hz, 1H), 6.75 (d, J = 9.0 Hz, 2H), 6.67 (t, J = 1.8 Hz, 1H), 6.26–6.25 (m, 1H), 5.87 (s, 1H), 2.98 (s, 6H), 2.51 (s, 3H), 2.38 (t, J = 7.7 Hz, 2H), 2.18 (s, 3H), 1.49–1.43 (m, 2H), 1.36–1.31 (m, 4H), 0.91 (t, J = 6.9 Hz, 3H); 13C NMR (126 MHz, CDCl3) 161.2, 150.3, 148.4, 146.6, 134.9, 131.1, 130.1, 126.0, 123.6, 120.6, 115.5, 115.2, 114.4, 113.4, 110.7, 99.6, 41.1, 31.9, 30.2, 24.3, 22.7, 14.2, 12.5, 9.7 (one carbon signal missing); 11B NMR (160 MHz, CDCl3) 1.41 (J = 35 Hz); 19F NMR (235 MHz, CDCl3,) −139 (q, J = 35 Hz); UV/vis (CH2Cl2) λmax (nm): 569, ε 55
000 mol L−1 cm−1. LRMS: 491.3 (M + H)+; HRMS: 491.2803. Found, 491.2788 calculated for C28H34BF2N4O.
Boron, difluoro[2-[5-((3,5-dimethyl-4-pentyl-2H-pyrrol-2-ylidene)methyl)-4-methoxy-1H-pyrrol-2-yl]-1H-indole] (4q).
Following GP1, over a 5 day period a solution of 1q (28 mg, 0.07 mmol) in CH2Cl2 (15 mL) was reacted with BF3·OEt2 (273 μL, 2.21 mmol) and NEt3 (205 μL, 1.47 mmol). The product was purified using column chromatography on basic alumina, eluting with 0%, 1%, 2% and then 4% ethyl acetate in hexanes, to provide F-BODIPY 4q (22 mg, 76% yield). 1H NMR (500 MHz, CDCl3) 10.19 (br s, 1H), 7.62 (d, J = 8.0 Hz, 1H), 7.50 (d, J = 8.2 Hz, 1H), 7.28–7.24 (m, 1H), 7.13–7.09 (m, 3H), 6.30 (s, 1H), 3.95 (s, 3H), 2.56 (s, 3H), 2.39 (t, J = 7.7 Hz, 2H), 2.17 (s, 3H), 1.49–43 (m, 2H), 1.38–1.32 (m, 4H), 0.92 (t, J = 6.8 Hz, 3H); 13C NMR (126 MHz, CDCl3) 161.8, 153.5, 146.4, 138.2, 136.3, 131.8, 130.7, 129.5, 128.1, 126.0, 124.4, 121.2, 120.6, 116.0, 112.2, 107.9, 98.0, 58.4, 31.9, 30.0, 24.3, 22.7, 14.2, 13.0, 9.7; 11B NMR (160 MHz, CDCl3) 1.39 (t, J = 35 Hz); 19F NMR (235 MHz, CDCl3) −138 (q, J = 35 Hz); UV/vis (CH2Cl2) λmax (nm): 579, ε 54
000 mol L−1 cm−1; fluorescence (CH2Cl2) λexci, 546 (nm); λmax (nm), 591, ΦF: 1.0; LRMS: 458.2 (M + Na)+; HRMS: 458.2183. Found, 458.2186 calculated for C25H28BF2N3NaO.
Boron, difluoro[benzyl 2-[(5-(1H-indol-2-yl)-3-methoxy-1H-pyrrol-2-yl)methylene]-3,5-dimethyl-4-pentyl-2H-pyrrol-4-carboxylate] (4r).
Following GP1, over a 5 hour period a solution of 1r (14 mg, 0.03 mmol) in CH2Cl2 (4 mL) was reacted with BF3·OEt2 (48 μL, 0.39 mmol) and NEt3 (38 μL, 0.27 mmol). The product was purified using column chromatography on basic alumina, eluting with CH2Cl2, to provide F-BODIPY 4r (10 mg, 71% yield). 1H NMR (500 MHz, CDCl3,) 10.24 (br s, 1H), 7.67 (d, J = 8 Hz, 1H), 7.54 (d, J = 8 Hz, 1H), 7.48 (d, J = 7 Hz, 2H), 7.43 (t, J = 7 Hz, 2H), 7.39–7.304 (m, 2H), 7.31 (s, 1H), 7.25 (s, 1H), 7.17 (t, J = 8 Hz, 1H), 6.38 (s, 1H), 5.37 (s, 2H), 4.05 (s, 3H), 2.90 (s, 3H), 2.49 (s, 3H); 13C NMR (126 MHz, CDCl3) 164.8, 164.0, 155.3, 151.1, 139.1, 139.0, 136.6, 130.3, 130.2, 129.3, 128.7, 128.4, 18.2, 127.9, 125.9, 121.7, 121.2, 117.0, 112.5, 111.1, 98.6, 65.9, 58.8, 14.8, 12.1; 11B NMR (160 MHz, CDCl3) 1.39 (J = 36 Hz); 19F NMR (235 MHz, CDCl3) −137 (q, J = 31 Hz); UV/vis (CH2Cl2) λmax (nm): 562, ε 74
000 mol L−1 cm−1; fluorescence (CH2Cl2) λexci, 546 (nm); λmax (nm), 574, ΦF: 0.95; LRMS: 522.2 (M + Na)+; HRMS: 522.1762. Found, 522.1771 calculated for C28H24BF2N3NaO3.
Conflicts of interest
There are no conflicts to declare.
Acknowledgements
We thank Dr Estelle Marchal for preparation of pyrrolyldipyrrin HCl salts, Pankaj Subedi for assistance with obtaining fluorescence spectra and Dr Norman Schepp for spectrofluorometer access.
References
- E. V. Antina, N. A. Bumagina, A. I. V'yugin and A. V. Solomonov, Fluorescent indicators of metal ions based on dipyrromethene platform, Dyes Pigm., 2017, 136, 368–381 CrossRef CAS.
- W. R. Hearn, M. K. Elson, R. H. Williams and J. Medina-Castro, Prodigiosene [5-(2-pyrryl)-2,2′-dipyrrylmethene] and some substitited prodigiosenes, J. Org. Chem., 1970, 35, 142–146 CrossRef CAS PubMed.
- R. D'Alessio, A. Bargiotti, O. Carlini, F. Colotta, M. Ferrari, P. Gnocchi, A. M. Isetta, N. Mongelli, P. Motta, A. Rossi, M. Rossi, M. Tibolla and E. Vanotti, Synthesis and immunosuppressive activity of novel prodigiosin derivatives, J. Med. Chem., 2000, 43, 2557–2565 CrossRef.
- E. Marchal, D. A. Smithen, I. M. Uddin, A. W. Robertson, D. L. Jakeman, V. Mollard, C. D. Goodman, K. S. MacDougall, S. A. McFarland, G. I. McFadden and A. Thompson, Antimalarial activity of prodigiosenes, Org. Biomol. Chem., 2014, 12, 4132–4142 CAS.
- E. Marchal, M. I. Uddin, D. A. Smithen, L. A. Hawco, M. Lanteigne, D. P. Overy, R. G. Kerr and A. Thompson, Antimicrobial activity of non-natural prodigiosenes, RSC Adv., 2013, 3, 22967–22971 RSC.
- E. Marchal, S. Rastogi, A. Thompson and J. T. Davis, Influence of B-ring modifications on proton affinity, transmembrane anion transport and anti-cancer properties of synthetic prodigiosenes, Org. Biomol. Chem., 2014, 12, 7515–7522 CAS.
- E. Marchal, M. I. Uddin, C. L. A. Hawco and A. Thompson, Synthesis of prodigiosene-estrogen conjugates: optimization of protecting group strategies and anticancer properties, Can. J. Chem., 2015, 93, 526–535 CrossRef CAS.
- T. E. Wood and A. Thompson, Advances in the chemistry of dipyrrins and their complexes, Chem. Rev., 2007, 107, 1831–1861 CrossRef CAS PubMed.
- M. Benstead, G. H. Mehl and R. W. Boyle, 4,4′-Difluoro-4-bora-3a,4a-diaza-s-indacenes (BODIPYs) as components of novel light active materials, Tetrahedron, 2011, 67, 3573–3601 CrossRef CAS.
- N. Boens, V. Leen and W. Dehaen, Fluorescent indicators based on BODIPY, Chem. Soc. Rev., 2012, 41, 1130–1172 RSC.
- A. Loudet and K. Burgess, BODIPY dyes and their derivatives: syntheses and spectroscopic properties, Chem. Rev., 2007, 107, 4891–4932 CrossRef CAS PubMed.
- R. Ziessel, G. Ulrich and A. Harriman, The chemistry of BODIPY: a new El Dorado for fluorescence tools, New J. Chem., 2007, 31, 496–501 RSC.
- S. M. Crawford, A. Al-Sheikh Ali, T. S. Cameron and A. Thompson, Synthesis and characterization of fluorescent pyrrolyldipyrrinato Sn(IV) complexes, Inorg. Chem., 2011, 50, 8207–8213 CrossRef CAS PubMed.
- G. Park, J. T. Tomlinson, M. S. Melvin, M. W. Wright, C. S. Day and R. A. Manderville, Zinc and copper complexes of prodigiosin: implications for copper-mediated double-strand DNA cleavage, Org. Lett., 2003, 5, 113–116 CrossRef CAS PubMed.
- M. R. Rao, M. D. Tiwari, J. R. Bellare and M. Ravikanth, Synthesis of BF2 complexes of prodigiosin type oligopyrroles, J. Org. Chem., 2011, 76, 7263–7268 CrossRef CAS PubMed.
- C. Yu, Q. Wu, J. Wang, Y. Wei, E. Hao and L. Jiao, Red to near-infrared isoindole BODIPY fluorophores: synthesis, crystal structures, and spectroscopic and electrochemical properties, J. Org. Chem., 2016, 81, 3761–3770 CrossRef CAS PubMed.
- M. Zhang, E. Hao, J. Zhou, C. Yu, G. Bai, F. Wang and L. Jiao, Synthesis of pyrrolyldipyrrinato BF2 complexes by oxidative nucleophilic substitution of boron dipyrromethene with pyrrole, Org. Biomol. Chem., 2012, 10, 2139–2145 CAS.
-
https://www.thermofisher.com/ca/en/home/brands/invitrogen.html
.
- S. Jenkins, C. D. Incarvito, J. Parr and H. H. Wasserman, Structural studies of C-ring substituted unnatural analogues of prodigiosin, CrystEngComm, 2009, 11, 242–245 RSC.
- V. Lakshmi, M. S. Shaikh and M. Ravikanth, Synthesis and properties of meso-unsubstituted 3-pyrrolyl boron dipyrromethene, J. Fluoresc., 2013, 23, 519–525 CrossRef CAS PubMed.
- M. Zhang, E. Hao, Y. Xu, S. Zhang, H. Zhu, Q. Wang, C. Yu and L. Jiao, One-pot efficient synthesis of pyrrolylBODIPY dyes from pyrrole and acyl chloride, RSC Adv., 2012, 2, 11215–11218 RSC.
- R. Sharma, V. Lakshmi, T. Chatterjee and M. Ravikanth, Effects of five membered aromatic heterocycles at the meso-position on the electronic properties of 3-pyrrolyl BODIPY, New J. Chem., 2016, 40, 5855–5860 RSC.
- K. J. Kadassery, A. Nimesh, S. Raj and N. Agarwal, 3-/3,5-Pyrrole-substituted BODIPY derivatives and their photophysical and electrochemical studies, J. Chem. Sci., 2016, 128, 1435–1443 CrossRef CAS.
- T. Kaur, V. Lakshmi and M. Ravikanth, Functionalized 3-pyrrolyl boron-dipyrromethenes, RSC Adv., 2013, 3, 2736–2745 RSC.
- V. Lakshmi, W.-Z. Lee and M. Ravikanth, Synthesis, structure and spectral and electrochemical properties of 3-pyrrolyl BODIPY-metal dipyrrin complexes, Dalton Trans., 2014, 43, 16006–16014 RSC.
- E. Dai, W. Pang, X.-F. Zhang, X. Yang, T. Jiang, P. Zhang, C. Yu, E. Hao, Y. Wei, X. Mu and L. Jiao, Synthesis and photophysics of BF2-rigidified partially closed chain bromotetrapyrroles: near infrared emitters and photosensitizers, Chem. – Asian J., 2015, 10, 1327–1334 CrossRef CAS PubMed.
- T. Jiang, P. Zhang, C. Yu, J. Yin, L. Jiao, E. Dai, J. Wang, Y. Wei, X. Mu and E. Hao, Straightforward synthesis of oligopyrroles through a regioselective SNAr reaction of pyrroles and halogenated boron dipyrrins, Org. Lett., 2014, 16, 1952–1955 CrossRef CAS PubMed.
- C. Yu, Y. Xu, L. Jiao, J. Zhou, Z. Wang and E. Hao, Isoindole-BODIPY dyes as red to near-infrared fluorophores, Chem. – Eur. J., 2012, 18, 6437–6442 CrossRef CAS PubMed.
- J. Li, Q. Zhang, J. Yin, C. Yu, K. Cheng, Y. Wei, E. Hao and L. Jiao, Metal-free and versatile synthetic routes to natural and synthetic prodiginines from boron dipyrrin, Org. Lett., 2016, 18, 5696–5699 CrossRef CAS PubMed.
- D. A. Smithen, A. E. G. Baker, M. Offman, S. M. Crawford, T. S. Cameron and A. Thompson, Use of F-BODIPYs as a protection strategy for dipyrrins: optimization of BF2 removal, J. Org. Chem., 2012, 77, 3439–3453 CrossRef CAS PubMed.
- S. Rastogi, E. Marchal, I. Uddin, B. Groves, J. Colpitts, S. A. McFarland, J. T. Davis and A. Thompson, Synthetic prodigiosenes and the influence of C-ring substitution on DNA cleavage, transmembrane chloride transport and basicity, Org. Biomol. Chem., 2013, 11, 3834–3845 CAS.
- G. Ulrich, R. Ziessel and A. Harriman, The chemistry of fluorescent BODIPY dyes: versatility unsurpassed, Angew. Chem., Int. Ed., 2008, 47, 1184–1201 CrossRef CAS PubMed.
- B. Díaz deGreñu, P. I. Hernández, M. Espona, D. Quiñonero, M. E. Light, T. Torroba, R. Pérez-Tomás and R. Quesada, Synthetic prodiginine Obatoclax (GX15-070) and related analogues: anion binding, transmembrane transport, and cytotoxicity properties, Chem. – Eur. J., 2011, 17, 14074–14083 CrossRef PubMed.
- S. M. Crawford and A. Thompson, Investigations into the nucleophilic meso-substitution of F-BODIPYs and improvements to the synthesis of 4,4-difluoro-4-bora-3a,4a-diaza-s-indacene, Heterocycles, 2011, 83, 311–322 CrossRef CAS.
- I. K. Petrushenko and K. B. Petrushenko, Effect of meso-substituents on the electronic transitions of BODIPY dyes: DFT and RI-CC2 study, Spectrochim. Acta, Part A, 2015, 138, 623–627 CrossRef CAS PubMed.
- X. Qi, E. J. Jun, L. Xu, S.-J. Kim, J. S. Joong Hong, Y. J. Yoon and J. Yoon, New BODIPY derivatives as OFF−ON fluorescent chemosensor and fluorescent chemodosimeter for Cu2+: cooperative selectivity enhancement toward Cu2+, J. Org. Chem., 2006, 71, 2881–2884 CrossRef CAS PubMed.
- T. Werner, C. Huber, S. Heinl, M. Kollmannsberger, J. Daub and O. S. Wolfbeis, Novel optical pH-sensor based on a boradiaza-indacene derivative, Fresenius’ J. Anal. Chem., 1997, 359, 150–154 CrossRef CAS.
-
K. Rurack, in Standardization and Quality Assurance in Fluorescence Measurements I: Techniques, ed. U. Resch-Genger, Springer Berlin Heidelberg, Berlin, Heidelberg, 2001, pp. 101 Search PubMed.
- S. Fery-Forgues and D. Lavabre, J. Chem. Educ., 1999, 76, 1260–1264 CrossRef CAS.
- M. I. Uddin, S. Thirumalairajan, S. M. Crawford, T. S. Cameron and A. Thompson, Improved synthetic route to C-ring ester-functionalized prodigiosenes, Synlett, 2010, 2561–2564 CAS.
Footnote |
† Electronic supplementary information (ESI) available: 1H, 13C NMR, absorption/emission spectra for all new compounds and crystal data for 2d. CCDC 1573487. For ESI and crystallographic data in CIF or other electronic format see DOI: 10.1039/c7pp00341b |
|
This journal is © The Royal Society of Chemistry and Owner Societies 2018 |
Click here to see how this site uses Cookies. View our privacy policy here.