DOI:
10.1039/C8RA01637B
(Paper)
RSC Adv., 2018,
8, 15448-15458
One-pot sequential multicomponent reaction between in situ generated aldimines and succinaldehyde: facile synthesis of substituted pyrrole-3-carbaldehydes and applications towards medicinally important fused heterocycles†
Received
24th February 2018
, Accepted 18th April 2018
First published on 24th April 2018
Abstract
An efficient sequential multi-component method for the synthesis of N-arylpyrrole-3-carbaldehydes has been developed. This reaction involved a proline-catalyzed direct Mannich reaction-cyclization sequence between succinaldehyde and in situ generated Ar/HetAr/indolyl-imines, followed by IBX-mediated oxidative aromatization in one-pot operation. The practical utility of this procedure is shown at gram-scale and the synthesis of diverse bioactive fused heterocyclic scaffolds such as pyrroloquinoline, pyrrolo-oxadiazole, dihydro pyrroloquinoline, and pyrrolo-phenanthridine.
Introduction
Medium sized nitrogen heterocycles are privileged scaffolds present in numerous natural and unnatural compounds.1 Among them, the pyrrole core is present in many important classes of natural products and its derivatives have been used as valuable intermediates for the synthesis of many drugs that exhibit interesting biological activities (Fig. 1).2 In addition, functionalized pyrroles have shown wide applications in agrochemicals and flavor components, dyes, and functionalized materials.3 Over the past few decades, a number of elegant methods to access functionalized pyrroles have been reported, which includes classical methods,4 cycloadditions,5 multi-component,6 metal-catalyzed reactions,7 and several others.8 Despite the extensive efforts, the synthesis of C3-functionalized pyrroles is probably the most difficult task and required a special strategy.9 In particular, the regiospecific synthesis of pyrroles endowed with aldehyde group at C3-position is still very rare.10 Pyrrole-3-carbaldehydes have mainly been synthesized by the use of sterically bulky triisopropylsilyl (TIPS) as protecting group on the nitrogen of pyrrole followed by Vilsmeier–Hacck formylation and deprotection as a multistep process (eqn (1), Scheme 1),10a along with other direct/indirect methods.10b–e However, these reported approaches have one or more drawbacks, such as the requirement of specially designed substrates, multistep process with low yields, and harsh reaction conditions. Furthermore, none of these methods could directly yield 1,2-disubstituted pyrrole-3-carbaldehydes, to the best of our knowledge. The development of synthetic protocol, which directly produces the required functionality at the desired position of heterocyclic ring systems, has become a major contribution to the pharmaceutical industry. Thus, the development of modular and simple pot-economic protocol to strategically access substituted pyrrole-3-carbaldehydes from easily available materials is still in high demand. Notably, aldehyde group at C3-position of pyrroles can readily participate in numerous name reactions, thus, holds significant promises to serve as a suitable intermediate to synthesize new medicinal agents and functionalized materials.11
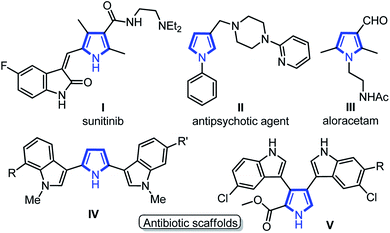 |
| Fig. 1 Bioactive pyrroles and related derivatives. | |
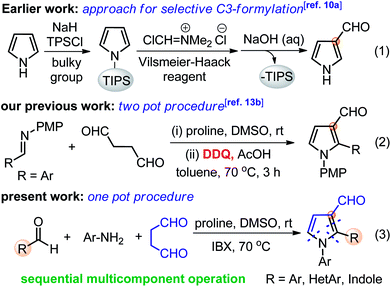 |
| Scheme 1 Synthetic approaches for pyrrole-3-carbaldehydes. | |
In continuation to our efforts to utilize using linear dialdehydes,12 for the synthesis of medium-sized N-heterocycles under metal-free conditions,13 we recently developed a two-pot protocol for the direct synthesis of pyrrole-3-carbaldehyde from succinaldehyde and imines (eqn (2), Scheme 1).13b This method resulted in a quick synthesis of pyrrole-3-aldehydes, though, it required the pre-assembled imines and DDQ as a harsh and toxic reagent for oxidative aromatization. The clear synthetic potential and novelty of this method to suitably functionalized pyrroles prompted us to explore similar transformation in one-pot sequential multicomponent fashion under mild conditions. In addition, the social and environmental demands for more sustainable and practical synthetic protocols that need the use of less hazardous reagents/conditions has also gained much attention of the scientific community. In this context, multicomponent reactions allow the rapid construction of novel libraries of pharmaceutically active compounds and marine alkaloids, thus, the development of such protocol is always applauded.14 Herein, we report a simple and most rational sequential multicomponent protocol for the synthesis of pyrrole-3-carbaldehydes via in situ imine formation between Ar/HetAr/indole-aldehydes and Ar-NH2, followed by amine-catalyzed direct Mannich reaction-cyclization with succinaldehyde, and IBX-mediated aromatization sequence in one-pot operation (eqn (3), Scheme 1). This improved method provides an easy access to pyrrole-3-carbaldehydes under mild and non-toxic condition as compare to our previous two-pot protocol. In addition, in situ generated imines derived from indole-3-aldehydes have been explored for the first time for such transformations to yield indole-based medicinally important scaffolds.
Results and discussion
Based on our previous experience in this direction, we quickly optimized the designed reactions by choosing proline 1 (20 mol%) as a catalyst, p-nitrobenzaldehyde 2c as model substrates, along with p-anisidine 3, and succinaldehyde 4 (3 M aqueous sol.), in one-pot operation and the results are shown in Table 1. Initial experiments in DMSO as the choice of solvent along with the sequential addition of substrates, catalyst, and oxidant(s) gave 5c in low yield (entry 1 and 2, Table 1). Attempts were made to improve the yields by changing the solvents (entries 2–4, Table 1), however, failed. IBX as the oxidant, also soluble in DMSO, showed good efficiency for this one-pot protocol at rt (entry 5, Table 1) and at 50 °C (entry 6, Table 1). Gratifyingly, an additional improvement in yields (80%) was observed when IBX-oxidation was carried out at 70 °C for 4 h (entry 7, Table 1). However, additional efforts to increase the reaction yield by further increment in reaction temperature (entry 8, Table 1), reduction in catalyst loading (entry 9, Table 1), varying the reaction medium (entries 10 and 11, Table 1), and changing the catalytic system (entry 12, Table 1) were ineffective. Thus, we prefer to perform this one-pot sequential transformation with the optimized conditions (entry 7, Table 1).
Table 1 Optimization of reaction conditionsa
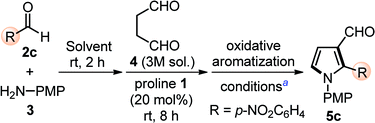
|
Entry |
Solvent |
Conditionsa |
Yieldb (%) |
Unless otherwise indicated, the reaction was carried out with (i) aldehyde 2 (0.3 mmol), p-anisidine 3 (0.3 mmol), succinaldehyde 4 (3 M aqueous sol., 0.9 mmol), proline 1 (20 mol%), solvent (3.0 mL); (ii) IBX (1.2 equiv.). Isolated yield of 5c refers to 2c. Proline 1 (10 mol%). EtOAc (3.0 mL) was added during IBX-mediated oxidative aromatization. Pyrrolidine (20 mol%), PhCO2H (20 mol%) were used in place of proline 1. |
1 |
DMSO |
K2S2O8 (1.2 equiv.), rt, 8 h |
35 |
2 |
DMSO |
Oxone (1.2 equiv.), rt, 24 h |
40 |
3 |
DMF |
Oxone (1.2 equiv.), rt, 24 h |
30 |
4 |
CH3CN |
Oxone (1.2 equiv.), rt, 24 h |
<20 |
5 |
DMSO |
IBX (1.2 equiv.), rt, 6 h |
50 |
6 |
DMSO |
IBX (1.2 equiv.), 50 °C, 6 h |
64 |
7 |
DMSO |
IBX (1.2 equiv.), 70 °C, 4 h |
80 |
8 |
DMSO |
IBX (1.2 equiv.), 90 °C, 4 h |
75 |
9c |
DMSO |
IBX (1.2 equiv.), 70 °C, 4 h |
58 |
10 |
DMF |
IBX (1.2 equiv.), 70 °C, 4 h |
43 |
11d |
CH3CN |
IBX (1.2 equiv.), 70 °C, 4 h |
35 |
12e |
DMSO |
IBX (1.2 equiv.), 70 °C, 4 h |
48 |
The scope of the reaction was examined by employing various aromatic aldehydes and the results are summarized in Table 2. This one-pot sequential multicomponent protocol works well in case of aromatic aldehydes decorated with various groups (e.g. –NO2, –F, –Cl, –Br, –CN and CF3) at the ortho-, meta-, or para-positions (entries 5a–5l, Table 2) and resulted in 2-aryl-pyrrole-3-carbaldehydes in good to high yields (65–80%). The reaction works well with in situ generated simple aryl imine (entry 5m, Table 2), as well as with hetero-aryl imines (entries 5n–5o, Table 2) with good yields. The feasibility of this protocol was also examined at gram scale of 2c (1.0 g) with other reactants under standardized conditions and corresponding product 5c was obtained without much variation in yields (1.60 g, 78%). The structure of 5c was further confirmed with single crystal X-ray diffraction analysis (Scheme 2).15
Table 2 Substrate scope with respect to various Ar/HetAr-aldehydes 2a
Unless otherwise indicated, the reaction was carried out with (i) aldehyde 2 (0.3 mmol), p-anisidine 3 (0.3 mmol), DMSO (3.0 mL), rt, 2 h, (ii) succinaldehyde 4 (3 M aqueous sol., 0.9 mmol), proline 1 (20 mol%), 8 h, (iii) IBX (1.2 equiv.), 70 °C, 4 h. Isolated yield of 5 refers to 2 (≤10% of aldehyde 2 was recovered in all the cases). |
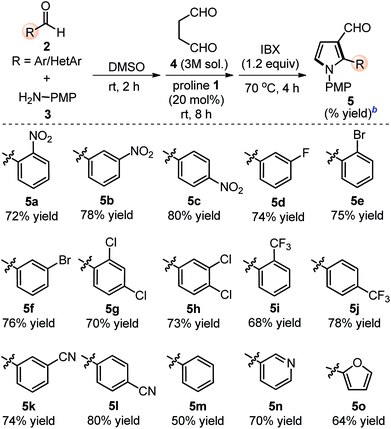 |
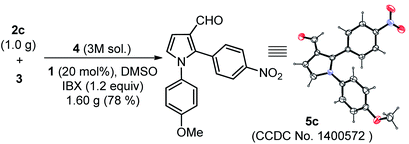 |
| Scheme 2 Single-crystal X-ray analysis of 5c. Thermal ellipsoids are drawn at the 40% probability level. | |
Indolyl-pyrroles synthesis
The scope of this one-pot protocol was further was examined by employing various in situ generated imines derived from indole-3-aldehydes 6. This extension to imines derived from indole-3-aldehydes could be motivating as these units have not been utilized for similar direct Mannich reaction and can lead to new structural scaffolds. Indole and its derivatives are an important family of alkaloid compounds and most abundant heterocycles found in nature, which possess interesting biological activities.16 Moreover, indole-tethered pyrrole derivatives (for example IV and V in Fig. 1) found in several synthetic compounds and marine alkaloids that showed remarkable bioactivities,17 therefore, the synthesis of these compounds is quite interesting. In this context, a series of indolyl-pyrrole-3-carbaldehydes 7a–7p were obtained with moderate to good yields, when N-Ts, Ms, SO2Ph, Boc protected indole-3-aldehydes 6 were employed for this transformation with p-anisidine 3 and succinaldehyde 4 (entries 7a–7p, Table 3). In addition, electron donating or withdrawing substitution on indole-ring did not alter the course of this transformation. Further, the reaction works quite well when other aryl-amines such as 2-aminophenol and 4-chloroaniline (entries 7q–7r, Table 3) were employed instead of p-anisidine 3 for this one-pot transformation with N-Ts-indol-3-aldehyde 6a and succinaldehyde 4. However, reaction failed when the similar transformation was performed with N-benzyl-indole-3-aldehyde, probably because of low reactivity of imine (entry 7s, Table 3). All compounds were well characterized by 1H and 13C-NMR and mass-analysis. Single crystal X-ray diffraction analysis of 7e further established the product structure (Fig. 2).15 Based on our study, a stepwise mechanism is proposed to account for this reaction. As shown in Scheme 3, the enamine A in situ generated from succinaldehyde 4 and catalyst 1, reacts with in situ generated N-PMP-imine B via a direct Mannich reaction model C to produced Mannich product D. The intermediate D underwent intramolecular cyclization to dihydropyrrole E with the simultaneous release of catalyst 1. In the same pot cyclic enamine-intermediate E underwent IBX-mediated oxidative aromatization to afford pyrrole-3-carboxaldehyde 4.
Table 3 Substrate scope with respect to various indole-3-aldehydes 6a
Unless otherwise indicated, the reaction was carried out with (i) aldehyde 6 (0.3 mmol), p-anisidine 3 (0.3 mmol), DMSO (3.0 mL), rt, 2 h, (ii) succinaldehyde 4 (3 M aqueous sol., 0.9 mmol), proline 1 (20 mol%), 8 h, (iii) IBX (1.2 equiv.), 70 °C, 4 h. Isolated yield refers to 6 (≤10% of aldehyde 6 was recovered in all the cases). |
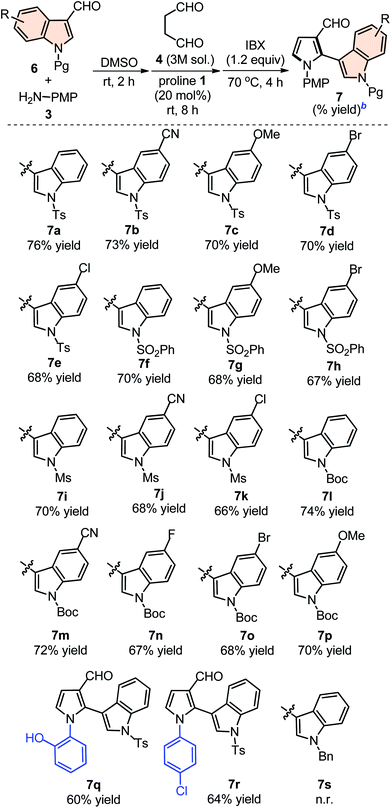 |
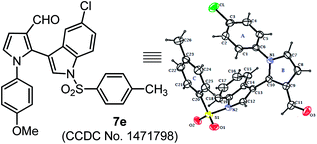 |
| Fig. 2 Single-crystal X-ray analysis of 7e. Thermal ellipsoids are drawn at the 40% probability level. | |
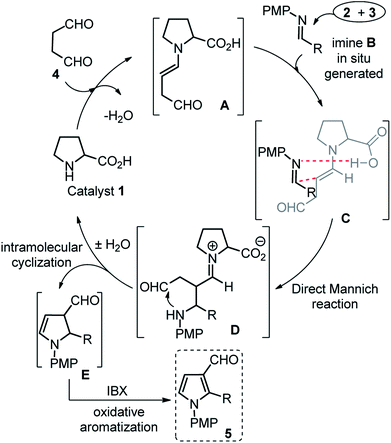 |
| Scheme 3 Proposed reaction mechanism for this study. | |
Synthetic applications
Substituted pyrrole-3-aldehydes could participate as suitable intermediates for further functionalization to many important and complex scaffolds, therefore, we developed interesting and useful synthetic applications of these compounds. In this context, a rapid synthesis of pyrrolo[3,2-c]quinoline 8 was developed through reductive cyclization. This reaction proceeded via in situ amine formation through reduction of nitro-group of 5a Fe/NH4Cl in EtOH
:
H2O (4
:
1), which underwent intramolecular cyclization with aldehyde group in the same pot with good yields (eqn (1), Scheme 4). In another approach, the synthesis of pyrrole-dihydroquinoline 10 was accomplished through the reductive amination of 5e with p-anisidine 3 in presence of NaBH4 to generate in situ amine 9, which was further utilized for CuI-catalyzed intramolecular coupling (C–N) without purification to furnished 10 with high yield (87%) over two steps (eqn (2), Scheme 4).18 The pyrroloquinoline moiety was found to be present in many natural/synthetic molecule with interesting bioactivity and our protocol may be better alternative to the previous procedure.19 The synthesized hybrid scaffolds resemble with various biologically active molecules such as pyrrolo[3,2-c]-quinoline derivative, an ATP-ase inhibitor,20a pyrrolo[2,3-c]-quinoline derivative, a natural product with acetylcholinesterase-inhibiting activity,20b and pyrrolo[3,4-c]quinoline derivative, a potent 5-HT4R antagonist with analgesic action.20c
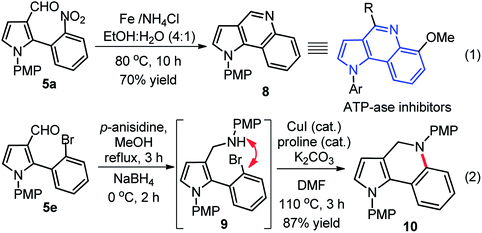 |
| Scheme 4 Synthesis of pyrroloquinoline 8 and pyrrolo-dihydroquinoline 10 scaffolds. | |
Further applications of our method were shown; (i) synthesis of pyrrole-oxadiazole 11 in good yield (76%) over two steps from compound 5c which was initially condensed with 4-nitrophenylhydrazide, followed by iodobenzene diacetate (IBD) mediated oxidative cyclization under the standardized conditions (eqn (1), Scheme 5),21 and (ii) rapid and high yielding (78%) synthesis of pyrrole-phenanthridine 13 from 5e through intramolecular C–C bond formation in presence Pd(OAc)2, PPh3 and K2CO3 in DMF at 130 °C as shown in (eqn (2), Scheme 5). Interestingly, 13 might exhibit interesting biological activities because phenanthridines serve as the core structure of natural products from Amaryllidaceae plants and received considerable attention from both chemists and biological scientists.22
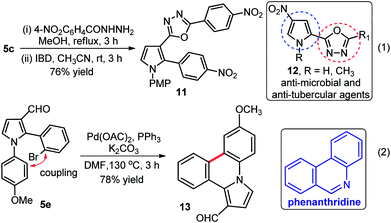 |
| Scheme 5 Synthesis of pyrrole-oxadiazole 11, and pyrrole-phenanthridine 13 moieties. | |
Conclusions
In summary, we have developed a straight forwarded sequential multicomponent synthesis of substituted N-aryl-pyrrole-3-carbaldehydes. This one-pot protocol proceeds through proline-catalyzed Mannich reaction-cyclization sequence between succinaldehyde and imines, in situ generated from Ar/HetAr/indole-aldehydes with aromatic amines, followed by IBX-mediated oxidative aromatization under mild conditions. Easy access to the starting materials and direct synthesis of pyrrole-3-carbaldehydes under metal-free conditions renders this method potentially useful in organic synthesis. Synthetic applicability of the developed method was established through; (i) at gram-scale synthesis, and (ii) the rapid access to the biologically important natural products analogous like-pyrrolo-quinoline, pyrrolo-oxadiazole, dihydro pyrroloquinoline, and pyrrolo-phenanthridine.
Experimental
General remarks
Unless otherwise stated, all reagents were purchased from commercial suppliers and used without further purification. All solvents employed in the reactions were distilled from appropriate drying agents prior to use. All reactions under standard conditions were monitored by thin-layer chromatography (TLC) on Merck silica gel 60 F254 pre-coated plates (0.25 mm). The column chromatography was performed on silica gel (100–200) using a mixture of hexane/EtOAc. Chemical yields refer to pure isolated substances. 1H-NMR spectra were recorded on a BRUKER-AV400 (400 MHz) spectrometer. Chemical shifts are reported in ppm from tetramethylsilane with the solvent resonance as the internal standard (CDCl3 = δ 7.26 for 1H, and 77.0 for 13C-NMR). Data are reported as follows: chemical shift, multiplicity (s = singlet, d = doublet, dd = doublet of doublet, t = triplet, q = quartet, br = broad, m = multiplet), coupling constants (Hz) and integration. 13C-NMR spectra were recorded on a BRUKER-AV400 (75 MHz) spectrometer with complete proton decoupling. HRMS were performed employing an ESI+ ionization method and TOF as an analyzer. Infrared (FT-IR) spectra were recorded on an ABB Bomen MB 3000 FTIR Spectrophotometer system using KBr pellets. Melting points were determined in open capillary tubes with an EZ-Melt automated melting point apparatus and may be incorrect.
Typical procedure for the synthesis of pyrrole-3-carboxaldehydes
To a stirred solution of Ar/HetAr-aldehyde 2 (0.3 mmol) or N-protected indole-3-aldehyde 6 (0.3 mmol) in DMSO (3.0 mL) was added p-anisidine 3 (0.3 mmol) and stirred initially for 2 h at rt. To this in situ generated-imine solution was added succinaldehyde 4 (0.3 mL, 0.9 mmol, 3 M solution) and proline 1 (7.0 mg, 0.06 mmol) at the same temperature. The combined reaction mixture was stirred further for 8 h at rt. At that time, IBX (100 mg, 0.36 mmol, 1.2 equiv.) was added to the reaction mixture and heated at 70 °C for additional 3 h. The reaction was cooled to room temperature quenched with NaHCO3 solution (10% solution, 5 mL) and extracted with EtOAc (3 × 6 mL). The combined organic extracts were washed with brine, dried over Na2SO4 anhydrous, and concentrated under reduced pressure. Purification through silica gel column chromatography by eluting the mixture of hexane/EtOAc, gave pyrrole-3-carbaldehydes 5 or 7 with 50–80% yields. In almost all the cases, we also obtained about <10% initial starting aldehyde due to cleavage of corresponding imine under these conditions.
1-(4-Methoxyphenyl)-2-(2-nitrophenyl)-1H-pyrrole-3-carbaldehyde (5a). Reddish pasty liquid (70 mg, 72%); 1H NMR (400 MHz, CDCl3) δ 3.77 (s, 3H), 6.77 (d, J = 8.9 Hz, 2H), 6.87 (d, J = 3.0 Hz, 1H), 6.94 (d, J = 3.0 Hz, 1H), 7.02 (d, J = 8.9 Hz, 2H), 7.45 (d, J = 6.5 Hz, 1H), 7.54 (t, J = 7.8 Hz, 1H), 7.62 (t, J = 6.6 Hz, 1H), 7.97 (d, J = 8.1 Hz, 1H), 9.60 (s, 1H); 13C NMR (75 MHz, CDCl3) δ 55.4, 109.1, 114.3 (2C), 124.4, 124.8, 125.1, 125.2, 126.9 (2C), 130.1, 130.8, 132.7, 134.1, 135.8, 149.3, 159.1, 185.5; IR (KBr)/cm−1, 2932, 1666, 1520, 1350, 1296, 1034; HRMS (ESI): calcd for C18H14N2O4 (MH+) 323.1032; found 323.1033.
1-(4-Methoxyphenyl)-2-(3-nitrophenyl)-1H-pyrrole-3-carbaldehyde (5b). Semi-solid (75 mg, 78%); 1H NMR (400 MHz, CDCl3) δ 3.73 (s, 3H), 6.77 (d, J = 8.8 Hz, 2H), 6.82 (d, J = 3.0 Hz, 1H), 6.87 (d, J = 3.0 Hz, 1H), 6.97 (d, J = 8.0 Hz, 2H), 7.41–7.49 (m, 2H), 8.01 (t, J = 1.4 Hz, 1H), 8.11 (d, J = 8.0 Hz, 1H), 9.65 (s, 1H); 13C NMR (75 MHz, CDCl3) δ 55.5, 108.8, 114.6 (2C), 123.2, 124.9, 125.5, 125.8, 127.3 (2C), 129.2, 130.8, 131.1, 136.6, 138.2, 147.9, 159.3, 186.0; IR (KBr)/cm−1 2920, 1746, 1680, 1244, 1172; HRMS (ESI): calcd for C18H14N2O4 (MH+) 323.1032; found 323.1033.
1-(4-Methoxyphenyl)-2-(4-nitrophenyl)-1H-pyrrole-3-carbaldehyde (5c). Pale yellow solid (mp = 102–104 °C) (74 mg, 80%); 1H NMR (400 MHz, CDCl3) δ 3.82 (s, 3H), 6.84 (d, J = 8.8 Hz, 2H), 6.89 (d, J = 3.0 Hz, 1H), 6.94 (d, J = 3.0 Hz, 1H), 7.01 (d, J = 8.8 Hz, 2H), 7.35 (d, J = 8.8 Hz, 2H), 8.16 (d, J = 8.8 Hz, 2H), 9.73 (s, 1H); 13C NMR (75 MHz, CDCl3) δ 55.4, 109.0, 114.6 (2C), 123.4 (2C), 125.1, 126.1, 127.1 (2C), 130.9, 131.6 (2C), 135.9, 138.2, 147.4, 159.3, 186.0; IR (KBr)/cm−1 2933, 1724, 1660, 1249, 1174; HRMS (ESI): calcd for C18H14N2O4 (MH+) 323.1032; found 323.1028.
2-(3-Fluorophenyl)-1-(4-methoxyphenyl)-1H-pyrrole-3-carbaldehyde (5d). Yellow pasty liquid (65 mg, 74%); 1H NMR (400 MHz, CDCl3) δ 3.80 (s, 3H), 6.83 (d, J = 8.9 Hz, 2H), 6.86 (d, J = 3.1 Hz, 1H), 6.89 (d, J = 2.8 Hz, 1H), 6.91 (t, J = 1.8 Hz, 1H), 7.00–7.06 (m, 4H), 7.28–7.32 (m, 1H), 9.70 (s, 1H); 13C NMR (75 MHz, CDCl3) δ 55.4, 107.8, 114.3 (2C), 115.7, 117.9, 124.6, 125.2, 126.8, 127.0, (2C), 129.8, 129.9, 131.3, 140.4, 158.9, 161.2, 186.6; IR (KBr)/cm−1, 2962, 1720, 1512, 1247, 1172; HRMS (ESI): calcd for C18H14FNO2 (MH+) 296.1087; found 296.1070.
2-(2-Bromophenyl)-1-(4-methoxyphenyl)-1H-pyrrole-3-carbaldehyde (5e). Red viscous liquid (80 mg, 75%); 1H NMR (400 MHz, CDCl3) δ 3.77 (s, 3H), 6.78 (d, J = 8.9 Hz, 2H), 6.86 (d, J = 3.1 Hz, 1H), 6.92 (d, J = 3.0 Hz, 1H), 6.95 (d, J = 8.9 Hz, 2H), 6.98–7.00 (m, 1H), 7.56 (dd, J = 7.9, 4.3 Hz, 2H), 7.64 (d, J = 8.3 Hz, 1H), 9.51 (s, 1H); 13C NMR (75 MHz, CDCl3) δ 55.4, 107.9, 114.2 (2C), 124.9, 125.1, 126.5 (2C), 126.9, 127.7, 129.7, 131.3, 134.0, 135.9, 136.1, 137.2, 158.9, 185.9; IR (KBr)/cm−1, 3016, 1720, 1519, 1226, 1026; HRMS (ESI): calcd for C18H14BrNO2 (MH+) 356.0286; found 356.0295.
2-(3-Bromophenyl)-1-(4-methoxyphenyl)-1H-pyrrole-3-carbaldehyde (5f). Brown pasty liquid (81 mg, 76%); 1H NMR (400 MHz, CDCl3) δ 3.81 (s, 3H), 6.84 (d, J = 2.1 Hz, 1H), 6.86 (d, J = 4.1 Hz, 2H), 6.89 (d, J = 3.0 Hz, 1H), 7.03 (d, J = 7.0 Hz, 2H), 7.08–7.11 (m, 1H), 7.17 (t, J = 7.8 Hz, 1H) 7.41 (t, J = 1.7 Hz, 1H) 7.45–7.48 (m, 1H), 9.69 (s, 1H); 13C NMR (75 MHz, CDCl3) δ 55.5, 108.0, 114.4 (2C), 122.2, 122.8, 124.7, 125.3, 127.1 (2C), 129.5, 129.7, 131.3, 131.6, 133.7, 137.2, 159.0, 186.5; IR (KBr)/cm−1, 2985, 1728, 1519, 1373, 1242, 1049; HRMS (ESI): calcd for C18H14BrNO2 (MH+) 356.0286; found 356.0288.
2-(2,4-Dichlorophenyl)-1-(4-methoxyphenyl)-1H-pyrrole-3-carbaldehyde (5g). Yellow oily liquid (77 mg, 70%); 1H NMR (400 MHz, CDCl3) δ 3.78 (s, 3H), 6.80 (d, J = 8.9 Hz, 2H), 6.86 (d, J = 3.1 Hz, 1H), 6.94 (d, J = 2.9 Hz, 1H), 7.03 (d, J = 8.9 Hz, 2H), 7.24 (d, J = 1.7 Hz, 2H),7.40 (s, 1H), 9.53 (s, 1H); 13C NMR (75 MHz, CDCl3) δ 55.4, 107.9, 114.2 (2C), 124.9, 125.1, 126.5 (2C), 126.9, 127.7, 129.7, 131.3, 134.0, 135.9, 136.1, 137.2, 158.9, 185.9; IR (KBr)/cm−1, 2954, 1668, 1514, 1469, 1246, 1031; HRMS (ESI): calcd for C18H13Cl2NO2 (MH+) 346.0401; found 346.0408.
2-(3,4-Dichlorophenyl)-1-(4-methoxyphenyl)-1H-pyrrole-3-carbaldehyde (5h). Yellow pasty liquid (80 mg, 73%); 1H NMR (400 MHz, CDCl3) δ 3.70 (s, 3H), 6.74 (d, J = 3.7 Hz, 2H), 6.75 (s, 1H), 6.78 (d, J = 3.1 Hz, 1H), 6.86–6.92 (m, 3H), 7.14 (s, 1H), 7.23 (t, J = 2.2 Hz, 2.0 Hz, 1H), 9.58 (s, 1H); 13C NMR (75 MHz, CDCl3) δ 55.4, 108.2, 114.5 (2C), 124.7, 125.5, 127.1 (2C), 129.3, 129.9, 130.2, 131.0, 132.5, 132.5, 133.0, 138.8, 159.1, 186.2; IR (KBr)/cm−1, 2962, 1697, 1514, 1253, 1031; HRMS (ESI): calcd for C18H13Cl2NO2 (MH+) 346.0401; found 346.0406.
1-(4-Methoxyphenyl)-2-(2-(trifluoromethyl)phenyl)-1H-pyrrole-3-carbaldehyde (5i). Yellow pasty liquid (75 mg, 68%); 1H NMR (400 MHz, CDCl3) δ 3.75 (s, 3H), 6.76 (d, J = 8.9 Hz, 2H), 6.85 (d, J = 3.1 Hz, 1H), 6.91 (d, J = 3.0 Hz, 1H), 7.03 (d, J = 8.8 Hz, 2H), 7.37 (t, J = 4.5 Hz, 1H), 7.51 (t, J = 4.9 Hz, 2H), 7.70 (t, J = 4.6 Hz, 1H), 9.41 (s, 1H); 13C NMR (75 MHz, CDCl3) δ 55.3, 107.5, 114.1 (2C), 114.6, 117.6, 120.1, 121.5, 122.0, 124.8, 126.5, 127.0 (2C), 129.5, 131.1, 131.4, 134.0, 158.9, 186.0; IR (KBr)/cm−1, 2955, 1666, 1520, 1311, 1250, 1119; HRMS (ESI): calcd for C19H14F3NO2 (MH+) 346.1055; found 346.1057.
1-(4-Methoxyphenyl)-2-(4-(trifluoromethyl)phenyl)-1H-pyrrole-3-carbaldehyde (5j). Yellow pasty liquid (85 mg, 78%); 1H NMR (400 MHz, CDCl3) δ 3.81 (s, 3H), 6.84 (d, J = 9.0 Hz, 2H), 6.89 (d, J = 3.1 Hz, 1H), 6.92 (d, J = 3.0 Hz, 1H), 7.02 (d, J = 8.9 Hz, 2H), 7.33 (d, J = 8.1 Hz, 2H), 7.58 (d, J = 8.1 Hz, 2H), 9.70 (s, 1H); 13C NMR (75 MHz, CDCl3) δ 55.4, 108.3, 114.5 (2C), 124.9, 125.1, 125.2 (3C), 125.6, 127.1 (2C), 131.1 (2C), 131.2 (2C), 133.0, 159.1, 186.4; IR (KBr)/cm−1, 2970, 1666, 1512, 1319, 1234; HRMS (ESI): calcd for C19H14F3NO2 (MH+) 346.1055; found 346.1053.
3-(3-Formyl-1-(4-methoxyphenyl)-1H-pyrrol-2-yl) benzonitrile (5k). Yellow pasty liquid (67 mg, 74%); 1H NMR (400 MHz, CDCl3) δ 3.81 (s, 3H), 6.84 (d, J = 8.8 Hz, 2H), 6.88 (d, J = 3.1 Hz, 1H), 6.92 (d, J = 3.0 Hz, 1H), 7.01 (d, J = 8.9 Hz, 2H), 7.42–7.49 (m, 3H), 7.41 (d, J = 7.0 Hz, 1H) 9.69 (s, 1H); 13C NMR (75 MHz, CDCl3) δ 55.4, 108.6, 112.6, 114.6 (2C), 118.0, 124.8, 125.7, 127.1 (2C), 129.1, 130.8, 130.9, 131.9, 134.0, 135.1, 138.4, 159.2, 186.0; IR (KBr)/cm−1, 2932, 2230, 1659, 1512, 1443, 1250; HRMS (ESI): calcd for C19H14N2O2 (MH+) 303.1134; found 303.1134.
4-(3-Formyl-1-(4-methoxyphenyl)-1H-pyrrol-2-yl) benzonitrile (5l). Pink pasty liquid (72 mg, 80%); 1H NMR (400 MHz, CDCl3) δ 3.81 (s, 3H), 6.85 (d, J = 8.9 Hz, 2H), 6.89 (d, J = 3.0 Hz, 1H), 6.94 (d, J = 2.5 Hz, 1H), 7.01 (d, J = 8.8 Hz, 2H), 7.32 (d, J = 8.3 Hz, 2H), 7.60 (d, J = 8.3 Hz, 2H), 9.72 (s, 1H); 13C NMR (75 MHz, CDCl3) δ 55.5, 108.9, 114.6 (2C), 118.2, 124.9, 125.0, 125.9, 127.1 (2C), 131.4 (2C), 131.9 (2C), 134.1, 138.8, 141.7, 159.3, 186.0; IR (KBr)/cm−1, 2962, 2229, 1712, 1519, 1242; HRMS (ESI): calcd for C19H14N2O2 (MH+) 303.1134; found 303.1134.
1-(4-Methoxyphenyl)-2-phenyl-1H-pyrrole-3-carbaldehyde (5m). Yellow pasty liquid (42 mg, 50%); 1H NMR (400 MHz, CDCl3) δ 3.77 (s, 3H), 6.80 (d, J = 8.8 Hz, 2H), 6.85 (d, J = 3.2 Hz, 1H), 6.87 (d, J = 3.2 Hz, 1H), 7.00 (d, J = 8.8 Hz, 2H), 7.18–7.20 (m, 2H), 7.28–7.32 (m, 3H), 9.67 (s, 1H); 13C NMR (75 MHz, CDCl3) δ 55.3, 107.6, 114.2 (2C), 124.3, 124.9, 127.0 (2C), 128.1 (2C), 128.4, 129.1, 130.9 (2C), 131.6, 142.4, 158.7, 187.0; IR (KBr)/cm−1, 2912, 1710, 1672, 1244, 1174; HRMS (ESI): calcd for C18H15NO2 (MH+) 278.1181; found 278.1189.
1-(4-Methoxyphenyl)-2-(pyridin-3-yl)-1H-pyrrole-3-carbaldehyde (5n). Red oily liquid (58 mg, 70%); 1H NMR (400 MHz, CDCl3) δ 3.81 (s, 3H), 6.85 (d, J = 8.9 Hz, 2H), 6.91 (d, J = 3.0 Hz, 1H), 6.96 (d, J = 2.9 Hz, 1H), 7.04 (d, J = 8.9 Hz, 2H), 7.28 (t, J = 3.7 Hz, 1H), 7.54 (d, J = 7.8 Hz, 1H), 8.5 (bs, 1H), 8.58 (d, J = 4.7 Hz, 1H), 9.72 (s, 1H); 13C NMR (75 MHz, CDCl3) δ 55.4, 108.4, 114.5 (2C), 122.9, 125.1, 125.7, 125.7, 127.3 (2C), 131.0, 137.8, 137.9, 149.4, 151.0, 159.2, 186.0; IR (KBr)/cm−1, 2954, 1666, 1512, 1242, 1033; HRMS (ESI): calcd for C17H14N2O2 (MH+) 279.1133; found 279.1140.
2-(Furan-2-yl)-1-(4-methoxyphenyl)-1H-pyrrole-3-carbaldehyde (5o). Red oily liquid (51 mg, 64%); 1H NMR (400 MHz, CDCl3) δ 3.85 (s, 3H), 6.05 (d, J = 3.3 Hz, 1H), 6.37 (d, J = 3.2 Hz, 1H), 6.93 (d, J = 8.8 Hz, 2H), 7.18 (d, J = 8.8 Hz, 3H), 7.99 (d, J = 7.7 Hz, 1H), 8.05 (d, J = 7.9 Hz, 1H), 10.08 (s, 1H); 13C NMR (75 MHz, CDCl3) δ 55.5, 108.1, 111.2, 111.7, 114.3 (2C), 126.0, 127.2 (2C), 127.9, 131.8, 133.1, 141.7, 143.4, 159.4, 187.4; IR (KBr)/cm−1, 2970, 1682, 1582, 1466, 1265, 1011; HRMS (ESI): calcd for C16H13NO3 (MH+) 268.0974; found 268.0980.
1-(4-Methoxyphenyl)-2-(1-tosyl-1H-indol-3-yl)-1H-pyrrole-3-carbaldehyde (7a). Yellow solid (107 mg, 76%, mp = 119–121 °C); 1H NMR (400 MHz, CDCl3) δ 2.37 (s, 3H), 3.78 (s, 3H), 6.71 (d, J = 8.9 Hz, 2H), 6.9 (d, J = 3.1 Hz, 1H), 6.97 (d, J = 2.4 Hz, 1H), 7.02 (d, J = 8.9 Hz, 2H), 7.12–7.16 (m, 1H), 7.23 (d, J = 8 Hz, 3H), 7.28–7.32 (m, 1H), 7.44 (s, 1H), 7.63 (d, J = 8.3 Hz, 2H), 7.95 (d, J = 8.3 Hz, 1H), 9.58 (s, 1H); 13C NMR (75 MHz, CDCl3) δ 29.6, 55.4, 108.1, 111.5, 113.5, 114.3 (2C), 120.3, 123.9, 125.2, 125.7, 125.8, 126.5 (2C), 126.8 (2C), 127.1, 129.9 (2C), 130.2, 131.7, 133.3, 134.4, 134.7, 145.3, 158.9, 186.1; IR (KBr)/cm−1, 2924, 2854, 1659, 1597, 1512, 1173; HRMS (ESI): calcd for C27H22N2O4S (MH+) 471.1378; found 471.1382.
3-(3-Formyl-1-(4-methoxyphenyl)-1H-pyrrol-2-yl)-1-tosyl-1H-indole-5-carbonitrile (7b). Brown solid (101 mg, 73%, mp = 123–125 °C); 1H NMR (400 MHz, CDCl3) δ 2.40 (s, 3H), 3.79 (s, 3H), 6.73 (d, J = 8.9 Hz, 2H), 6.90 (d, J = 3.1 Hz, 1H), 7.00–703 (m, 3H), 7.28 (d, J = 8.0 Hz, 2H), 7.45 (d, J = 8.8 Hz, 1H), 7.52 (dd, J = 7.1 Hz, 1H), 7.64 (s, 1H), 7.67 (d, J = 8.4 Hz, 2H), 8.05 (d, J = 8.1 Hz, 1H), 9.60 (s, 1H); 13C-NMR (75 MHz, CDCl3) δ 29.6, 55.5, 109.1, 111.4, 114.4, 114.5 (2C), 125.4, 126.0, 126.5 (2C), 126.9 (2C), 128.1, 128.1, 129.1, 129.2, 129.8, 130.2, 130.3 (2C), 130.9, 131.3, 134.1, 136.0, 146.2, 159.1, 185.5; IR (KBr)/cm−1, 2924, 2854, 2230, 1720, 1666, 1512, 1173; HRMS (ESI): calcd for C28H21N3O4S (MH+) 496.1331; found 496.1336.
2-(5-Methoxy-1-tosyl-1H-indol-3-yl)-1-(4-methoxyphenyl)-1H-pyrrole-3-carbaldehyde (7c). Red solid (105 mg, 70%, mp = 127–129 °C); 1H NMR (400 MHz, CDCl3) δ 2.37 (s, 3H), 3.64 (s, 3H), 3.78 (s, 3H), 6.57 (d, J = 2.4 Hz, 1H), 6.71 (d, J = 8.9 Hz, 2H), 6.88–6.9 (m, 2H), 6.98 (d, J = 2.7 Hz, 1H), 7.02 (d, J = 8.9 Hz, 2H), 7.22 (d, J = 8.2 Hz, 2H), 7.43 (s, 1H), 7.62 (d, J = 8.4 Hz, 2H), 7.82 (d, J = 9.1 Hz, 1H), 9.58 (s, 1H); 13C-NMR (75 MHz, CDCl3) δ 21.5, 55.4, 55.5, 102.0, 108.2, 111.5, 114.3 (2C), 114.5, 115.0, 125.6, 125.8, 126.3 (2C), 126.7 (2C), 127.9, 129.1, 129.9 (2C), 131.1, 131.8, 132.4, 134.7, 145.2, 156.8, 158.9, 186.1; IR (KBr)/cm−1, 2924, 2854, 1720, 1659, 1512, 1173; HRMS (ESI): calcd forC28H24N2O5S (MH+) 501.1484; found 501.1488.
2-(5-Bromo-1-tosyl-1H-indol-3-yl)-1-(4-methoxyphenyl)-1H-pyrrole-3-carbaldehyde (7d). Yellow solid (115 mg, 70%, mp = 139–141 °C); 1H NMR (400 MHz, CDCl3) δ 2.38 (s, 3H), 3.79 (s, 3H), 6.72 (d, J = 8.9 Hz, 2H), 6.89 (d, J = 3.2 Hz, 1H), 6.97 (d, J = 2.5 Hz, 1H), 7.00 (d, J = 8.9 Hz, 2H), 7.24 (d, J = 8.0 Hz, 2H), 7.30 (d, J = 1.7 Hz, 2H), 7.37 (d, J = 7.0 Hz, 1H), 7.45 (s, 1H), 7.60 (d, J = 8.4 Hz, 2H), 7.81 (d, J = 8.8 Hz, 1H), 9.57 (s, 1H); 13C NMR (400 MHz, CDCl3) δ 29.6, 55.5, 108.5, 110.9, 114.4 (2C), 115.0, 117.5, 123.1, 125.8, 125.9, 126.6 (2C), 126.8 (2C), 128.3, 128.3, 130.1 (2C), 131.5, 131.8, 132.3, 133.1, 134.4, 145.7, 159.1, 185.8; IR (KBr)/cm−1, 2924, 2854, 1720, 1666, 1512, 1250, 1119; HRMS (ESI): calcd for C27H21BrN2O4S (MH+) 549.0483; found 549.0488.
2-(5-Chloro-1-tosyl-1H-indol-3-yl)-1-(4-methoxyphenyl)-1H-pyrrole-3-carbaldehyde (7e). Brown solid (102 mg, 68%, mp = 134–136 °C); 1H NMR (400 MHz, CDCl3) δ 2.17 (s, 3H), 3.58 (s, 3H), 6.52 (d, J = 8.9 Hz, 2H), 6.67 (d, J = 3.1 Hz, 1H), 6.76 (dd, J = 2.4 Hz, 1H), 6.80 (d, J = 8.9 Hz, 2H), 6.95 (d, J = 1.9 Hz, 1H), 7.02–7.05 (m, 4H), 7.40 (d, J = 8.4 Hz, 2H), 7.65 (d, J = 8.8 Hz, 1H), 9.36 (s, 1H); 13C NMR (75 MHz, CDCl3) δ 29.7, 55.5, 108.4, 111.0, 114.4 (2C), 114.6, 120.0, 125.6, 125.8, 125.9, 126.6 (2C), 126.8 (2C), 128.5, 130.0, 130.1 (2C), 131.4, 131.5, 132.5, 132.8, 134.5, 145.6, 159.1, 185.8; IR (KBr)/cm−1, 2924, 2854, 1666, 1512, 1250, 1173; HRMS (ESI): calcd for C27H21ClN2O4S (MH+) 505.0911; found 505.0916.
1-(4-Methoxyphenyl)-2-(1-(phenylsulfonyl)-1H-indol-3-yl)-1H-pyrrole-3-carbaldehyde (7f). Brown solid (96 mg, 70%, mp = 117–119 °C); 1H NMR (400 MHz, CDCl3), δ 3.77 (s, 3H), 6.69 (d, J = 8.93 Hz, 2H), 6.89 (d, J = 3.1 Hz, 1H), 6.96 (d, J = 3.3 Hz, 1H), 7.00 (d, J = 8.9 Hz, 2H), 7.12–7.16 (m, 1H), 7.23 (d, J = 7.6 Hz, 1H), 7.28–7.32 (m, 1H), 7.42–7.46 (m, 3H), 7.54–7.59 (m, 1H), 7.72–7.74 (m, 2H), 7.96 (d, J = 8.3 Hz, 1H), 9.58 (s, 1H); 13C NMR (75 MHz, CDCl3), δ 55.4, 108.1, 111.7, 113.4, 114.3 (2C), 120.3, 123.9, 125.3, 125.7, 126.5 (2C), 126.6 (2C), 127.1, 129.3 (2C), 130.2, 131.6, 132.2, 134.0, 134.4 (2C), 137.6, 158.8, 186.1; IR (KBr)/cm−1, 2932, 2839, 1720, 1666, 1512, 1225, 1180; HRMS (ESI): calcd for C26H20N2O4S (MH+) 457.1222; found 457.1226.
2-(5-Methoxy-1-(phenylsulfonyl)-1H-indol-3-yl)-1-(4-methoxyphenyl)-1H-pyrrole-3-carbaldehyde (7g). Yellow viscous liquid (99 mg, 68%); 1H NMR (400 MHz, CDCl3) δ 3.64 (s, 3H), 3.77 (s, 3H), 6.56 (d, J = 2.4 Hz, 1H), 6.70 (d, J = 8.9 Hz, 2H), 6.88–6.91 (m, 2H), 6.98 (d, J = 2.4 Hz, 1H), 7.00 (d, J = 8.9 Hz, 2H), 7.42–7.45 (m, 3H), 7.54–7.59 (m, 1H), 7.71–7.73 (m, 2H), 7.84 (d, J = 9.1 Hz, 1H), 9.58 (s, 1H); 13C NMR (75 MHz, CDCl3) δ 55.4, 55.5, 102.0, 108.2, 111.8, 114.3 (2C), 114.5, 115.0, 125.6, 125.8, 126.3 (2C), 126.6 (2C), 127.8, 129.1, 129.3 (2C), 131.1, 131.7, 133.3, 134.0, 137.6, 156.9, 158.9, 186.1; IR (KBr)/cm−1, 2924, 2854, 1728, 1666, 1512, 1466, 1250, 1180; HRMS (ESI): calcd for C27H22N2O5S (MH+) 487.1327; found 487.1332.
2-(5-Bromo-1-(phenylsulfonyl)-1H-indol-3-yl)-1-(4-methoxyphenyl)-1H-pyrrole-3-carbaldehyde (7h). Brownish yellow gummy liquid (107 mg, 67%); 1H NMR (400 MHz, CDCl3) δ 3.82 (s, 3H), 3.76 (d, J = 8.9 Hz, 2H), 6.91 (d, J = 3.1 Hz, 1H), 7.00 (d, J = 3.1 Hz, 1H), 7.3 (d, J = 8.9 Hz, 2H), 7.35 (d, J = 1.5 Hz, 1H), 7.42 (d, J = 6.9 Hz, 1H), 7.48–7.51 (m, 3H), 7.60–7.65 (m, 1H), 7.74 (d, J = 7.3 Hz, 2H), 7.86 (d, J = 8.8 Hz, 1H), 9.60 (s, 1H); 13C NMR (75 MHz, CDCl3) δ 55.5, 108.4, 111.1, 114.4 (2C), 114.9, 117.6, 123.1, 123.9, 125.8, 126.6 (2C), 126.7 (2C) 128.2, 128.4, 129.5 (2C), 131.4, 131.8, 132.1, 133.1, 134.3, 137.3, 159.0, 185.8; IR (KBr)/cm−1, 2924, 2854, 1666, 1572, 1443, 1250, 1180; HRMS (ESI): calcd for C26H19BrN2O4S (MH+) 535.0319; found 535.0325.
1-(4-Methoxyphenyl)-2-(1-(methylsulfonyl)-1H-indol-3-yl)-1H-pyrrole-3-carbaldehyde (7i). Yellow pasty liquid (82 mg, 70%); 1H NMR (400 MHz, CDCl3) δ 3.07 (s, 3H), 3.75 (s, 3H), 6.77 (d, J = 9.0 Hz, 2H), 6.91 (d, J = 3.1 Hz, 1H), 7.01 (d, J = 2.4 Hz, 1H), 7.08 (d, J = 9.0 Hz, 2H), 7.21–7.25 (m, 1H), 7.31–7.34 (m, 2H), 7.35–7.39 (m, 1H), 7.90 (d, J = 8.4 Hz, 1H), 9.70 (s, 1H); 13C NMR (75 MHz, CDCl3) δ 40.9, 55.4, 108.4, 113.0, 114.3 (2C), 120.7, 124.2, 125.3, 125.6, 125.7, 125.9, 126.5 (2C), 126.9, 130.2, 131.7, 132.9, 134.6, 159.1, 186.1; IR (KBr)/cm−1 2924, 2854, 1659, 1443, 1373, 1134; HRMS (ESI): calcd for C21H18N2O4S (MH+) 395.1065; found 395.1070.
3-(3-Formyl-1-(4-methoxyphenyl)-1H-pyrrol-2-yl)-1-(methylsulfonyl)-1H-indole-5-carbonitrile (7j). Brownish solid (85 mg, 68%, mp = 114–116 °C); 1H NMR (400 MHz, CDCl3) δ 3.17 (s, 3H), 3.77 (s, 3H), 6.8 (d, J = 8.9 Hz, 2H), 6.93 (d, J = 3.1 Hz, 1H), 7.03 (d, J = 2.6 Hz, 1H), 7.09 (d, J = 9.0 Hz, 2H), 7.53 (m, 1H), 7.58 (d, J = 7.1 Hz, 1H), 7.99–8.03 (m, 2H), 9.73 (s, 1H); 13C NMR (75 MHz, CDCl3) δ 41.8, 55.5, 107.9, 109.6, 111.5, 114.1, 114.6 (2C), 118.6, 121.5, 125.8, 126.0, 126.5 (2C), 128.0, 128.4, 129.0, 131.3, 137.1, 141.7, 159.4, 185.6; IR (KBr)/cm−1, 2924, 2854, 2230, 1659, 1512, 1381, 1180; HRMS (ESI): calcd for C22H17N3O4S (MH+) 419.0940; found 419.0946.
2-(5-Chloro-1-(methylsulfonyl)-1H-indol-3-yl)-1-(4-methoxyphenyl)-1H-pyrrole-3-carbaldehyde (7k). Yellowish solid (85 mg, 66%, mp = 126–128 °C); 1H NMR (400 MHz, CDCl3) δ 3.07 (s, 3H), 3.77 (s, 3H), 6.79 (d, J = 8.9 Hz, 2H), 6.91 (d, J = 3.1 Hz, 1H), 7.01 (d, J = 2.8 Hz, 1H), 7.08 (d, J = 8.9 Hz, 2H), 7.25 (d, J = 1.9 Hz, 1H), 7.31 (d, J = 6.8 Hz, 1H), 7.36 (s, 1H), 7.82 (d, J = 8.8 Hz, 1H), 9.70 (s, 1H); 13C NMR (75 MHz, CDCl3) δ 40.1, 54.5, 107.8, 113.2, 113.4 (2C), 119.3, 121.6, 124.8, 124.9, 125.6 (2C), 126.0, 127.2, 129.2, 130.2, 130.4, 131.9, 135.6, 158.2, 184.8; IR (KBr)/cm−1, 2924, 2854, 1666, 1572, 1443, 1250, 1180; HRMS (ESI): calcd for C21H17ClN2O4S (MH+) 429.0676; found 429.0682.
tert-Butyl-3-(3-formyl-1-(4-methoxyphenyl)-1H-pyrrol-2-yl)-1H-indole-1-carboxylate (7l). White solid, (92 mg, 74%, mp = 117–119 °C); 1H NMR (400 MHz, CdCl3), δ 1.67 (s, 9H), 3.74 (s, 3H), 6.66 (d, J = 9.0 Hz, 2H), 6.92 (d, J = 3.2 Hz, 1H), 6.98 (d, J = 2.5 Hz, 1H), 7.07–7.16 (m, 5H), 7.28–7.30 (m, 1H), 7.57 (s, 1H), 8.12 (d, J = 8.1 Hz, 1H), 9.36 (s, 1H); 13C NMR (75 MHz, CDCl3) δ 28.1 (3C), 29.7, 55.44, 84.5, 108.0, 109.7, 114.3 (2C), 115.1, 120.1, 123.2, 124.9, 125.5, 125.9, 126.3 (2C), 127.2, 132.0, 134.7, 139.3, 149.2, 158.8, 186.7; IR (KBr)/cm−1, 2934, 2860, 1726, 1666, 1512, 1250, 1157; HRMS (ESI): calcd for C25H24N2O4 (MH+) 417.1814; found 417.1820.
tert-Butyl-5-cyano-3-(3-formyl-1-(4-methoxyphenyl)-1H-pyrrol-2-yl)-1H-indole-1-carboxylate (7m). Reddish brown solid (95 mg, 72%, mp = 120–122 °C); 1H NMR (400 MHz, CDCl3) δ 1.05 (s, 9H), 3.59 (s, 3H), 6.53 (d, J = 8.9 Hz, 2H), 6.70 (d, J = 3.1 Hz, 1H), 6.82 (d, J = 9.0 Hz, 3H), 7.07 (d, J = 3.7 Hz, 1H), 7.09 (s, 1H), 7.32 (d, J = 1.4 Hz, 1H), 7.84 (d, J = 8.7 Hz, 1H), 9.40 (s, 1H); 13C NMR (75 MHz, CDCl3) δ 29.7 (3C), 31.3, 55.5, 107.5, 109.1, 111.3, 114.5 (2C), 118.7, 125.5, 126.0, 126.5 (2C), 126.9, 128.1, 129.2, 129.9, 130.3, 131.0, 131.3, 134.1, 136.0, 146.2, 159.1, 185.6; IR (KBr)/cm−1, 2932, 2862, 2230, 1666, 1512, 1173, 1250; HRMS (ESI): calcd for C26H23N3O4 (MH+) 442.1767; found 442.1774.
tert-Butyl-5-fluoro-3-(3-formyl-1-(4-methoxyphenyl)-1H-pyrrol-2-yl)-1H-indole-1-carboxylate (7n). Yellow solid (87 mg, 67%, mp = 119–121 °C); 1H NMR (400 MHz, CDCl3) δ 1.66 (s, 9H), 3.75 (s, 3H), 6.74–6.79 (m, 3H), 6.90 (d, J = 3.1 Hz, 1H), 6.97–7.00 (m, 2H), 7.13 (d, J = 8.9 Hz, 2H), 7.62 (s, 1H), 8.07 (d, J = 5.9 Hz, 1H), 9.68 (s, 1H), 13C-NMR (75 MHz, CDCl3), δ 28.1 (3C), 29.7, 55.4, 84.8, 105.6, 105.8, 108.2, 109.6, 112.8, 113.0, 114.4 (2C), 116.2, 125.6, 126.3 (2C), 128.6, 131.9, 134.0, 149.0, 158.9, 160.3, 186.5; IR (KBr)/cm−1, 2924, 2854, 1736, 1666, 1450, 1366, 1250, 1172; HRMS (ESI): calcd for C25H23FN2O4 (MH+) 435.1720; found 435.1726.
tert-Butyl-5-bromo-3-(3-formyl-1-(4-methoxyphenyl)-1H-pyrrol-2-yl)-1H-indole-1-carboxylate (7o). White solid (82 mg, 68%, mp = 129–131 °C); 1H NMR (400 MHz, CDCl3) δ 1.66 (s, 9H), 3.02 (s, 3H), 6.79 (d, J = 8.98 Hz, 2H), 6.90 (d, J = 3.12 Hz, 1H), 6.98 (d, J = 3.9 Hz, 1H), 7.12 (d, J = 8.9 Hz, 2H), 7.22 (d, J = 1.7 Hz, 1H), 7.36 (d, J = 6.9 Hz, 1H), 7.75 (s, 1H), 7.99 (d, J = 8.4 Hz, 1H), 9.68 (s, 1H), 13C NMR (75 MHz, CDCl3), δ 28.1 (3C), 29.7, 55.5, 85.8, 108.2, 109.1, 114.4 (2C), 116.6, 122.8, 125.6, 125.9, 126.4 (2C) 127.8, 128.2, 131.1, 131.8, 133.7, 133.8, 148.8, 159.0, 186.3; IR (KBr)/cm−1, 2932, 2862, 1736, 1680, 1458, 1373, 1157; HRMS (ESI): calcd for C25H23BrN2O4 (MH+) 495.0919; found 405.0924.
tert-Butyl-3-(3-formyl-1-(4-methoxyphenyl)-1H-pyrrol-2-yl)-5-methoxy-1H-indole-1-carboxylate (7p). Dark brown solid (89 mg, 70%, mp = 124–126 °C); (400 MHz, CDCl3), δ 1.66 (s, 9H), 3.64 (s, 3H), 3.75 (s, 3H), 6.51 (d, J = 2.4 Hz, 1H), 6.78 (d, J = 8.9 Hz, 2H), 6.87 (d, J = 6.6 Hz, 1H), 6.93 (d, J = 3.2 Hz, 1H), 7.00 (d, J = 2.4 Hz, 1H), 7.15 (d, J = 9 Hz, 2H), 7.57 (s, 1H), 7.99 (d, J = 8.7 Hz, 1H), 9.71 (s, 1H); 13C-NMR (100 MHz, CDCl3) δ 28.1 (3C), 29.6, 55.4, 55.5, 84.3, 102.0, 108.0, 109.5, 114.3 (2C), 115.9, 125.4, 125.8, 126.1 (2C) 127.7, 129.6, 130.2, 132.2, 134.8, 149.1, 156.1, 158.8, 186.6; IR (KBr)/cm−1, 2932, 2862, 1736, 1666, 1512, 1250, 1157; HRMS (ESI): calcd for C26H26N3O5 (MH+) 425.1171; found 425.1176.
1-(2-Hydroxyphenyl)-2-(1-tosyl-1H-indol-3-yl)-1H-pyrrole-3-carbaldehyde (7q). Light yellow solid (82 mg, 60%, mp = 118–120 °C); 1H NMR (400 MHz, CDCl3) δ 2.42 (s, 3H), 6.95 (d, J = 3.2 Hz, 1H), 7.07–7.01 (m, 3H), 7.22–7.15 (m, 3H), 7.25 (d, J = 7.4 Hz, 1H), 7.30 (d, J = 8.5 Hz, 2H), 7.36–7.38 (m, 1H), 7.46 (s, 1H), 7.67 (d, J = 8.4 Hz, 2H), 8.02 (d, J = 8.4 Hz, 1H), 9.63 (s, 1H); 13C NMR (75 MHz, CDCl3) δ 21.6, 108.7, 111.0, 113.6, 120.1, 124.02, 125.2, 125.4, 126.3, 126.4 (2C), 126.7 (2C), 126.9, 127.0, 129.3 (2C), 130.0 (2C), 132.8, 133.5, 134.4, 134.6, 137.1, 145.5, 186.0; IR (KBr)/cm−1, 3458, 2924, 2854, 1659, 1497, 1443, 1088; HRMS (ESI): calcd for C26H20N2O4S (MH+) 457.1222; found 457.1227.
1-(4-Chlorophenyl)-2-(1-tosyl-1H-indol-3-yl)-1H-pyrrole-3-carbaldehyde (7r). Light pinkish solid (91 mg, 64%, mp = 132–134 °C) 1H NMR (400 MHz, CDCl3) δ 2.35 (s, 3H), 6.70 (m, 1H), 6.78 (s, 1H), 6.87 (m, 2H), 6.97 (d, J = 7.9 Hz, 1H), 7.25–7.10 (m, 4H), 7.37–7.28 (m, 2H), 7.40 (d, J = 7.9 Hz, 1H), 7.56–7.50 (m, 2H), 7.90 (d, J = 8.4 Hz, 1H), 9.43 (s, 1H), 13C NMR (75 MHz, CDCl3) δ 21.6, 108.4, 110.7, 113.4, 117.3, 120.0, 120.4, 123.9, 125.2, 125.2, 125.6, 126.1, 126.7 (2C), 127.0, 128.2, 129.9 (2C), 130.1, 130.3, 134.2, 134.6, 135.1, 145.1, 151.8, 186.0; IR (KBr)/cm−1, 2924, 2854, 1651, 1504, 1443, 1173; HRMS (ESI): calcd for C26H19ClN2O3S (MH+) 475.0883; found 475.0888.
1-(4-Methoxyphenyl)-1H-pyrrolo[3,2-c] quinoline (8). To a stirred solution of 5a (50 mg, 0.15 mmol) in EtOH
:
H2O (5 mL, 4
:
1) was added Fe-powder (86.9 mg, 1.55 mmol, 10.0 equiv.) and NH4Cl (100 mg, 1.8 mmol, 12.0 equiv.) and combined mixture was heated at 80 °C for 10 h. The reaction progress was monitored by TLC, cooled and concentrated under reduced pressure once completed. The crude residue was extracted between EtOAc/NaHCO3 solutions. Organic layer dried over Na2SO4 and evaporated under reduced pressure followed by silica-gel chromatography purification by eluting the mixture of hexane/EtOAc, gave pure product 8 as pasty yellow liquid (28 mg, 67% yield). 1H NMR (400 MHz, CDCl3) δ 3.95 (s, 3H), 6.87 (d, J = 3.1 Hz, 1H), 7.09 (d, J = 8.6 Hz, 2H), 7.21 (d, J = 3.1 Hz, 1H), 7.23 (m, 1H), 7.38 (d, J = 9.1 Hz, 1H), 7.43 (d, J = 8.8 Hz, 2H), 7.53 (t, J = 7.0 Hz, 1H), 8.19 (d, J = 8.3 Hz, 1H), 9.21 (s, 1H); 13C NMR (75 MHz, CDCl3) δ 55.6, 103.3, 114.8 (2C), 118.2, 120.6, 121.6, 125.3, 126.4, 128.5 (2C), 129.9, 130.2, 133.2, 134.6, 144.2, 146.1, 159.9; IR (KBr)/cm−1, 2924, 1713, 1512, 1366, 1250, 1034; HRMS (ESI): calcd for C18H14N2O (MH+) 275.1185; found 275.1190.
1,5-Bis(4-methoxyphenyl)-4,5-dihydro-1H-pyrrolo[3,2-c] quinoline (10). A mixture of 5e (0.1 g, 0.28 mmol, 1.0 equiv.) and p-anisidine 3 (0.030 g, 0.28 mmol, 1.0 equiv.) in methanol (3 mL) was refluxed for 2 h at 80 °C and followed by reductive amination in the presence of NaBH4 at 0 °C to obtain intermediate product. This crude intermediate 9 (0.1 g, 0.22 mmol, 1.0 equiv.) was taken in oven-dried round-bottom flask dissolved in DMF (2 mL), followed by the addition of base K2CO3 (61 mg, 0.44 mmol, 2.0 equiv.), CuI (9 mg, 20 mol%), L-proline as ligand (10 mg, 40 mol%). The resulting solution was stirred at 110 °C for 3 h under an N2 atmosphere. On completion, the residue was cooled to ambient temperature and then diluted with water (5 mL) and extracted with EtOAc (2 × 5 mL). The combined organic layers were dried over anhydrous Na2SO4 and evaporated to dryness. The crude residue was purified by column chromatography by eluting the mixture of hexane/EtOAc, afford 10 as yellow pasty liquid (70 mg, 87% yield). 1H NMR (400 MHz, CDCl3) δ 7.67 (dd, J = 7.8, 1.7 Hz, 1H), 7.56 (dd, J = 7.9, 1.2 Hz, 1H), 7.28–7.33 (m, 1H), 7.23 (d, J = 9.0 Hz, 2H), 7.08–7.14 (m, 1H), 6.93 (d, J = 2.4 Hz, 1H), 6.90 (d, J = 9.0 Hz, 2H), 6.74 (d, J = 1.8 Hz, 1H), 6.72 (d, J = 9.0 Hz, 2H), 6.47 (d, J = 8.9 Hz, 2H), 6.25 (d, J = 1.8 Hz, 1H), 5.80 (s, 1H), 3.81 (s, 3H), 3.70 (s, 3H); 13C NMR (75 MHz, CDCl3) δ 55.5, 55.7, 56.2, 109.2, 114.2 (2C), 114.5 (2C), 114.7 (2C), 118.1, 119.9, 121.9 (2C), 123.5, 126.7, 127.8, 128.1, 128.4, 132.9, 134.1, 141.3, 142.2, 151.9, 157.6; HRMS (ESI): calcd for C25H22N2O2 (MH+) 383.1759; found 383.1765.
2-(1-(4-Methoxyphenyl)-2-(4-nitrophenyl)-1H-pyrrol-3-yl)-5-(4-nitrophenyl)-1,3,4-oxadiazole (11). A mixture of 5c (0.08 g, 0.24 mmol, 1.0 equiv.) and 4-nitrobenzohydrazide (0.044 g, 0.24 mmol, 1.0 equiv.) in methanol (3.0 mL) was stirred for 2 hours under 80 °C and then concentrated in vacuo. The crude material was taken in CH3CN (3.0 mL) and IBD (0.08 g, 0.24 mmol, 1.0 equiv.) was added stirred at room temperature for one hour. The mixture was then concentrated in vacuo. To the residue was added H2O (10 mL) and the resulting mixture was extracted with ethylacetate (10 mL × 3). The organic layer was dried over Na2SO4 and concentrated. The residue was purified by column chromatography on silica gel by eluting the mixture of hexane/EtOAc, afford product 11 as yellow solid (92 mg, 76% yield). 1H NMR (400 MHz, CDCl3) (mp = 112–115 °C) δ 8.33 (d, J = 9.0 Hz, 2H), 8.17 (d, J = 8.9 Hz, 2H), 8.08 (d, J = 9.0 Hz, 2H), 7.52 (d, J = 8.9 Hz, 2H), 7.07 (d, J = 3.0 Hz, 1H), 7.04 (d, J = 9.0 Hz, 2H), 7.01 (d, J = 3.0 Hz, 1H), 6.84 (d, J = 9.0 Hz, 2H), 3.81 (s, 3H); 13C NMR (75 MHz, CDCl3) δ 55.5, 108.0, 109.8, 114.5 (2C), 123.0 (2C), 124.3 (2C), 125.9, 127.1 (2C), 127.3 (2C), 129.4, 131.3, 132.0 (2C), 132.4, 137.0, 147.3, 149.2, 159.2, 161.6, 162.7; HRMS (ESI): calcd for C25H17N5O6 (MH+) 484.1258; found 484.1263.
7-Methoxypyrrolo [1,2-f] phenanthridine-1-carbaldehyde (13). A clean oven-dried 10 mL round-bottom flask was charged with 5e (70 mg, 0.19 mmol, 1.0 equiv.) in DMF (2 mL), K2CO3 (54 mg, 0.39 mmol, 2.0 equiv.), ligand PPh3 (10 mg, 20 mol%), and Pd(OAc)2 (5 mg, 10 mol%). The resulting solution was stirred at 130 °C for 3 h under an N2 atmosphere. On completion, the reaction mass was cooled to ambient temperature and then diluted with water (5 mL) and extracted with EtOAc (2 × 5 mL). The combined organic layers were dried over anhydrous Na2SO4 and evaporated to dryness. The crude residue so obtained was purified by column chromatography by eluting the mixture of hexane/EtOAc, afford 13 as white solid (43 mg, 78% yield). 1H NMR (400 MHz, CDCl3) δ 10.26 (s, 1H), 9.49 (dd, J = 7.1, 2.3 Hz, 1H), 8.33 (dd, J = 7.3, 2.2 Hz, 1H), 7.88 (d, J = 9.2 Hz, 1H), 7.85 (d, J = 2.7 Hz, 1H), 7.80 (d, J = 3.3 Hz, 1H), 7.62–7.70 (m, 2H), 7.24 (d, J = 3.3 Hz, 1H), 7.22 (dd, J = 9.1, 2.8 Hz, 1H), 3.99 (s, 3H); 13C NMR (75 MHz, CDCl3) δ 55.7, 106.6, 114.0, 115.1, 117.0, 117.2, 117.7, 119.0, 120.5, 122.2, 123.9, 125.3, 127.2, 127.4, 128.5, 128.7, 157.2, 185.1; HRMS (ESI): calcd for C18H13BrNO2 (MH+) 276.1024; found 276.1029.
Conflicts of interest
There are no conflicts to declare.
Acknowledgements
This work was supported by OPERA-grant of BITS Pilani and Department of Science & Technology, Science and Engineering Board (DST-SERB) New Delhi (EMR/2016/005599). AS thanks to UGC New Delhi for Research Fellowships. Authors are also grateful for generous support from the DST-FIST support to Department of Chemistry at BITS-Pilani.
Notes and references
-
(a) J. A. Joule and K. Mills, Heterocyclic Chemistry, Blackwell, Oxford, 4th edn, 2000 Search PubMed;
(b) T. Eicher and S. Hauptmann, The Chemistry of Heterocycles, Wiley-VCH, Weinheim, 2003 CrossRef;
(c) A. R. Katrizky and A. F. Pozharskii, Handbook of Heterocyclic Chemistry, Pergamon, Amsterdam, 2nd edn, 2000 Search PubMed;
(d) I. S. Young, P. D. Thornton and A. Thompson, Nat. Prod. Rep., 2010, 27, 1801 RSC;
(e) M. Baumann, I. R. Baxendale, S. V. Ley and N. Nikbin, Beilstein J. Org. Chem., 2011, 7, 442 CrossRef CAS PubMed.
-
(a) Pyrroles, The Chemistry of Heterocyclic Compounds part I., ed. R. A. Jones, Wiley, New York, 1990, vol. 48 Search PubMed;
(b) H. Fan, J. Peng, M. T. Hamann and J.-F. Hu, Chem. Rev., 2008, 108, 264 CrossRef CAS PubMed;
(c) V. Bhardwaj, D. Gumber, V. Abbot, S. Dhiman and P. Sharma, RSC Adv., 2015, 46, 15233 RSC.
-
(a) M. E. Mason, B. Johnson and M. Hamming, J. Agric. Food Chem., 1966, 14, 454 CrossRef CAS;
(b) M. M. M. Raposo, A. M. C. Fonseca, M. C. R. Castro, M. Belsley, M. F. S. Cardoso, L. M. Carvalho and P. J. Coelho, Dyes Pigm., 2011, 91, 62 CrossRef CAS;
(c) M. Takase, N. Yoshida, T. Narita, T. Fujio, T. Nishinaga and M. Iyoda, RSC Adv., 2012, 2, 3221 RSC;
(d) M. M. Wienk, M. Turbiez, J. Gilot and R. A. J. Janssen, Adv. Mater., 2008, 20, 2556 CrossRef CAS;
(e) Y. Wang, G. A. Sotzing and R. A. Weiss, Chem. Mater., 2008, 20, 2574 CrossRef CAS;
(f) S. K. Ibrahim, X. M. Liu, C. Tard and C. J. Pickett, Chem. Commun., 2007, 1535 RSC;
(g) S. J. Higgins, Chem. Soc. Rev., 1997, 26, 247 RSC;
(h) C.-F. Lee, L.-M. Yang, T.-Y. Hwu, A.-S. Feng, J.-C. Tseng and T.-Y. Luh, J. Am. Chem. Soc., 2000, 122, 4992 CrossRef CAS;
(i) C. D'Silva and D. A. Walker, J. Org. Chem., 1998, 63, 6715 CrossRef;
(j) A. Berlin, B. Vercelli and G. Zotti, Polym. Rev., 2008, 48, 493 CrossRef CAS.
- For recent reviews, see
(a) V. Estevez, M. Villacampa and J. C. Menendez, Chem. Soc. Rev., 2010, 39, 4402 RSC;
(b) J. Bergman and T. Janosik, Mod. Heterocycl. Chem., 2011, 1, 269 CAS;
(c) X. Qi, X. Xu and C.-M. Park, Chem. Commun., 2012, 48, 3996 RSC.
- A. Padwa and W. H. Pearson, Synthetic Applications of 1,3-Dipolar Cycloaddition Chemistry Toward Heterocycles and Natural Products, Wiley, New York, 2002, vol. 59 Search PubMed.
- For reviews on multicomponent reactions, see
(a) V. Estèvez, M. Villacampa and J. C. Menéndez, Chem. Soc. Rev., 2010, 39, 4402 RSC;
(b) G. Balme, D. Bouyssi and N. Monteiro, Heterocycles, 2007, 73, 87 CrossRef CAS.
- For recent review see; A. V. Gulevich, A. S. Dudnik, N. Chernyak and V. Gevorgyan, Chem. Rev., 2013, 113, 3084 CrossRef CAS PubMed.
-
(a) S. Chiba, Y. Wang, G. Lapointe and K. Narasaka, Org. Lett., 2008, 10, 313 CrossRef CAS PubMed;
(b) G. Minetto, L. F. Raveglia, A. Sega and M. Taddei, Eur. J. Org. Chem., 2005, 5277 CrossRef CAS;
(c) S. Thirumalairajan, B. M. Pearce and A. Thompson, Chem. Commun., 2010, 46, 1797 RSC;
(d) S. Ngwerume and J. E. Camp, Chem. Commun., 2011, 47, 1857 RSC;
(e) O. A. Attanasi, G. Favi, F. Mantellini, G. Moscatelli and S. Santeusanio, J. Org. Chem., 2011, 76, 2860 CrossRef CAS PubMed;
(f) M. Zhang and J. Zhang, Chem. Commun., 2012, 48, 6399 RSC;
(g) L. Meng, K. Wu, C. Liu and A. Lei, Chem. Commun., 2013, 49, 5853 RSC;
(h) V. Estevez, M. Villacampa and J. C. Menendez, Chem. Commun., 2013, 49, 591 RSC;
(i) T. Wu, L. Pan, X. Xu and Q. Liu, Chem. Commun., 2014, 50, 1797 RSC;
(j) Y. H. Xu, T. He, Q. C. Zhang and T. P. Loh, Chem. Commun., 2014, 50, 2784 RSC;
(k) C. Zhou and D. Ma, Chem. Commun., 2014, 50, 3085 RSC;
(l) H. P. Kalmode, K. S. Vadagaonkar, K. Murugan and A. C. Chaskar, New J. Chem., 2015, 39, 4631 RSC;
(m) W. W. Tan and N. Yoshikai, Chem. Sci., 2015, 6, 6448 RSC;
(n) X. Wu, K. Li, S. Wang, C. Liu and A. Lei, Org. Lett., 2016, 18, 56 CrossRef CAS PubMed;
(o) T. J. Cogswell, C. S. Donald and R. Marquez, Org. Biomol. Chem., 2016, 14, 183 RSC.
-
(a) C. P. Andrieux, P. Audebert, A. Merz and R. Schwarz, New J. Chem., 1990, 14, 637 CAS;
(b) J. M. Méndez, B. Flores, F. León, M. E. Martinez, A. Vazquez, G. A. Garcia and M. Salmón, Tetrahedron Lett., 1996, 37, 4099 CrossRef;
(c) N. P. Pavri and M. L. Trudell, J. Org. Chem., 1997, 62, 2649 CrossRef CAS PubMed;
(d) J. E. Beecher and D. A. Tirrell, Tetrahedron Lett., 1998, 39, 3927 CrossRef CAS;
(e) A. Bunrit, S. Sawadjoon, S. Tšupova, P. J. R. Sjöberg and J. S. M. Samec, J. Org. Chem., 2016, 81, 1450 CrossRef CAS PubMed.
-
(a) B. L. Bray, P. H. Mathies, R. Naef, D. R. Solas, T. T. Tidwell, D. R. Artis and J. M. Muchowski, J. Org. Chem., 1990, 55, 6317 CrossRef CAS; For direct method
(b) A. Hamdan and J. W. F. Wasley, Synth. Commun., 1983, 13, 741 CrossRef CAS; For indirect methods
(c) H. Dumoulin, F. Fabis, P. Dallemagne, S. Roult and M. Robba, Synth. Commun., 1994, 24, 1855 CrossRef;
(d) P. Haldar, J. Guin and J. K. Ray, Tetrahedron Lett., 2005, 46, 1071 CrossRef CAS;
(e) A. R. Kelly, M. H. Kerrigan and P. J. Walsh, J. Am. Chem. Soc., 2008, 130, 4097 CrossRef CAS PubMed.
-
(a) H. Jendralla, E. Baader, W. Bartmann, G. Beck, A. Bergmann, E. Granzer, B. Kerekjarto, K. Kesseler, R. Krause, W. Schubert and G. Wess, J. Med. Chem., 1990, 33, 61 CrossRef CAS PubMed;
(b) D. Dumoulin, S. Rault and M. Robba, J. Heterocycl. Chem., 1996, 33, 255 CrossRef;
(c) R. He, L.-F. Zeng, Y. He, L. Wu, A. M. Gunawan and Z.-Y. Zhang, Chem. Commun., 2013, 49, 2064 RSC;
(d) S. Valente, S. Tomassi, G. Tempera, S. Saccoccio, E. Agostinelli and A. Mai, J. Med. Chem., 2011, 54, 8228 CrossRef CAS PubMed;
(e) W. M. Sigmund, G. Weerasekera, C. Marestin, S. Styron, H. Zhou, M. Z. Elsabee, J. Rühe, G. Wegner and R. S. Duran, Langmuir, 1999, 15, 6423 CrossRef CAS;
(f) F. Martinnelli, A. Palmieri and M. Petrini, Chem.–Eur. J., 2011, 17, 7183 CrossRef PubMed;
(g) L. J. Perez, T. K. Karagounis, A. Hurley, B. L. Bassle and M. F. Semmelhack, Chem. Sci., 2014, 5, 151 RSC;
(h) M. R. Rivero and S. L. Buchwald, Org. Lett., 2007, 9, 973 CrossRef CAS PubMed;
(i) M. S. Butler, J. Nat. Prod., 2004, 67, 2141 CrossRef CAS PubMed;
(j) M. Toganoh, Y. Kawabe and H. Furuta, J. Org. Chem., 2011, 76, 7618 CrossRef CAS PubMed.
- I. Kumar, P. Ramaraju, N. A. Mir and A. Singh, Org. Biomol. Chem., 2015, 13, 1280 CAS.
-
(a) I. Kumar, N. A. Mir, V. K. Gupta and Rajnikant, Chem. Commun., 2012, 48, 6975 RSC;
(b) I. Kumar, N. A. Mir, P. Ramaraju and B. P. Wakhloo, RSC Adv., 2012, 2, 8922 RSC;
(c) I. Kumar, P. Ramaraju, N. A. Mir, D. Singh, V. K. Gupta and Rajnikant, Chem. Commun., 2013, 49, 5645 RSC;
(d) I. Kumar, N. A. Mir, P. Ramaraju, D. Singh, V. K. Gupta and Rajnikant, RSC Adv., 2014, 4, 34548 RSC;
(e) P. Ramaraju, N. A. Mir, D. Singh, V. K. Gupta, R. Kant and I. Kumar, Org. Lett., 2015, 17, 5582 CrossRef CAS PubMed;
(f) N. A. Mir, S. Choudhary, P. Ramaraju, D. Singh and I. Kumar, RSC Adv., 2016, 6, 39741 RSC;
(g) P. Ramaraju, N. A. Mir, D. Singh and I. Kumar, RSC Adv., 2016, 6, 60422 RSC;
(h) P. Ramaraju, N. A. Mir, D. Singh, P. Sharma, R. Kant and I. Kumar, Eur. J. Org. Chem., 2017, 3461 CrossRef CAS.
-
(a) A. Shaabani, A. Maleki, H. Mofakham and H. R. Khavasi, J. Comb. Chem., 2008, 10, 883 CrossRef CAS PubMed;
(b) G. Guillena, D. J. Ramon and M. Yus, Tetrahedron: Asymmetry, 2007, 18, 693 CrossRef CAS;
(c) D. Tejedor, D. Gonzalez-Cruz, A. Santos-Exposito, J. J. Marrero-Tellado, P. de-Armas and F. Garcıa-Tellado, Chem.–Eur. J., 2005, 11, 3502 CrossRef CAS PubMed;
(d) B. B. Toure and D. G. Hall, Chem. Rev., 2009, 109, 4439 CrossRef CAS PubMed;
(e) A. Dömling, W. Wang and K. Wang, Chem. Rev., 2012, 112, 3083 CrossRef PubMed;
(f) T. Guntreddi, B. K. Allam and K. N. Singh, Synlett, 2012, 2635 CAS;
(g) S. R. Roy, P. S. Jadhavar, K. Seth, K. K. Sharma and A. K. Chakraborti, Synthesis, 2011, 2261 CAS;
(h) H. Benjamin, S. Z. Rotstein, V. Rai and K. Y. Andrei, Chem. Rev., 2014, 114, 8323 CrossRef PubMed.
- See ESI.†.
-
(a) R. J. Sundberg, The Chemistry of Indoles, Academic Press, New York, 1970 Search PubMed;
(b) G. W. Gribble, in Comprehensive Heterocyclic Chemistry II, ed. A. R. Katritzky, C. W. Ress, E. F. V. Scriven and C. W. Bird, Pergamon Press, Oxford, 1996, vol. 2, p. 207 Search PubMed;
(c) D. J. Faulkner, Nat. Prod. Rep., 2002, 19, 1 CAS;
(d) G. Bartoli, G. Bencivenni and R. Dalpozzo, Chem. Soc. Rev., 2010, 39, 4449 RSC;
(e) W. Gul and M. T. Hamann, Life Sci., 2005, 78, 442 CrossRef CAS PubMed;
(f) A. J. Kochanowska-Karamyan and M. T. Hamann, Chem. Rev., 2010, 110, 4489 CrossRef CAS PubMed.
-
(a) K. A. McArthur, S. S. Mitchell, G. Tsueng, A. Rheingold, D. J. White, J. Grodberg, K. S. Lam and B. C. M. Potts, J. Nat. Prod., 2008, 71, 1732 CrossRef CAS PubMed;
(b) D. S. Black, M. C. Bowyer and N. Kumar, Tetrahedron, 1997, 53, 8573 CrossRef CAS;
(c) J. Lehuédé, B. Fauconneau, L. Barrier, M. Ourakow, A. Piriou and J. M. Vierfond, Eur. J. Med. Chem., 1999, 34, 991 CrossRef;
(d) M. S. Mohamed, R. Kamel and S. S. Fatahala, Eur. J. Med. Chem., 2011, 46, 3022 CrossRef CAS PubMed;
(e) N. R. Williamson, P. C. Fineran, T. Gristwood, S. R. Chawrai, F. J. Leeper and G. P. C. Salmond, Future Microbiol., 2007, 2, 605 CrossRef CAS PubMed;
(f) J. Lavrado, R. Moreira and A. Paulo, Curr. Med. Chem., 2010, 17, 2348 CrossRef CAS PubMed;
(g) A. Carbone, B. Parrino, P. Barraja, V. Spanò, G. Cirrincione, P. Diana, A. Maier, G. Kelter and H.-H. Fiebig, Mar. Drugs, 2013, 11, 643 CrossRef CAS PubMed;
(h) W. Zhang, Z. Liu, S. Li, T. Yang, Q. Zhang, L. Ma, X. Tian, H. Zhang, C. Huang, S. Zhang, J. Ju, Y. Shen and C. Zhang, Org. Lett., 2012, 14, 3364 CrossRef CAS PubMed.
-
(a) F. Zhou, J. Guo, J. Liu, K. Ding, S. Yu and Q. Cai, J. Am. Chem. Soc., 2012, 134, 14326 CrossRef CAS PubMed;
(b) Z. Wang, W. Bao and Y. Jiang, Chem. Commun., 2005, 2849 RSC.
- R. L. Goff, A. M. Lawson, A. Daich and S. Comesse, Org. Biomol. Chem., 2013, 11, 1818 Search PubMed.
-
(a) T. H. Brown, R. J. Ife, D. J. Keeling, S. M. Laing, C. A. Leach, M. E. Parsons, C. A. Price, D. R. Reavill and K. J. Wiggall, J. Med. Chem., 1990, 33, 527 CrossRef CAS PubMed;
(b) Y. Sangnoi, O. Sakulkeo, S. Yuenyongsawad, A. Kanjanaopas, K. Ingkaninan, A. Plubrukarn and K. Suwanborirux, Mar. Drugs, 2008, 6, 578 CrossRef CAS PubMed;
(c) G. Furlotti, M. A. Alisi, C. Apicella, A. C. de Joannon, N. Cazzolla, R. Costi, G. C. Crucitti, B. Garrone, A. Iacovo, G. Magarò, G. Mangano, G. Miele, R. Ombrato, L. Pescatori, L. Polenzani, F. Rosi, M. Vitiello and R. Di Santo, J. Med. Chem., 2012, 55, 9446 CrossRef CAS PubMed.
-
(a) R. Y. Yang and L. X. Dai, J. Org. Chem., 1993, 58, 3301 Search PubMed;
(b) R. A. Rane, B. Pavankumar, S. D. Borhade and P. K. Khandare, Eur. J. Med. Chem., 2013, 70, 49 CrossRef CAS PubMed;
(c) A. Idhayadhulla, R. S. Kumar, A. J. A. Nasser and A. Manilal, Bull. Chem. Soc. Ethiop., 2012, 26, 429 CrossRef CAS.
-
(a) S. Ghosal, K. S. Saini and A. W. Frahm, Phytochemistry, 1983, 22, 2305 CrossRef CAS;
(b) S. Ghosal, Y. Kumar, D. K. Chakrabarti, J. Lal and S. K. Singh, Phytochemistry, 1986, 25, 1097 CrossRef CAS;
(c) Z. Jin, Nat. Prod. Rep., 2009, 26, 363 RSC;
(d) K. Merz, T. Muller, S. Vanderheiden, G. Eisenbrand, D. Marko and S. Brase, Synlett, 2006, 3461 CAS;
(e) T. Ishikawa, Med. Res. Rev., 2001, 21, 61 CrossRef CAS PubMed;
(f) H. Tsukamoto, S. Kondo, Y. Mukudai, T. Nagumo, A. Yasuda, Y. Kurihara, T. Kamatani and S. Shintani, Anticancer Res., 2011, 31, 2841 CAS.
Footnotes |
† Electronic supplementary information (ESI) available. CCDC 1400572 and 1471798. For ESI and crystallographic data in CIF or other electronic format see DOI: 10.1039/c8ra01637b |
‡ Both the authors contributed equally. |
|
This journal is © The Royal Society of Chemistry 2018 |