DOI:
10.1039/C7RA11900C
(Paper)
RSC Adv., 2017,
7, 56239-56246
Microbial oil production by Mortierella isabellina from corn stover under different pretreatments
Received
31st October 2017
, Accepted 8th December 2017
First published on 12th December 2017
Abstract
Mixed culture of Trichoderma reesei and Aspergillus niger was employed to accomplish on-site cellulase production where cellulases were applied directly to the enzymatic hydrolysis of pretreated corn stover. We comprehensively compared the five pretreatments including sodium hydroxide, steam explosion, aqueous ammonia, lime and diluted sulfuric acid in the enzymatic hydrolysis and then in the whole processes from corn stover to single cell oil (SCO). The results were not completely but roughly the same. However, it is conclusive that sodium hydroxide pretreatment was the best one. The process with sodium hydroxide pretreatment could produce 23.5 g dry cell biomass harboring 13.7 g single cell oil (SCO) from 146.2 g corn stover. The total yields of cell biomass and SCO were 0.161 and 0.094 g g−1 corn stover, respectively. This process was proven the most efficient. Through this work, we established efficient SCO production process from corn stover.
1. Introduction
As the second largest producer of corn stover in the world,1 China produces up to 0.3 billion metric tons of corn stover annually. However, the corn stover is often burned, causing serious environmental problem. Nonetheless, it is an important resource that could be utilized to produce valuable products in an environment-friendly and sustainable way. This is good for China's environment protection and rural economy.
Microbial lipids, regarded as single cell oils (SCO), are more renewable than plant oils or animal fats. They have much shorter production period, don't compete with food production and are much easier to scale up. Many microorganisms can accumulate high content of lipids, among which Mortierella isabellina is able to accumulate more than 80% lipids of its dry cell biomass.2–4 M. isabellina's excellence in SCO production and its good tolerance towards the inhibitors derived from the pretreatment of lignocelluloses suggest that M. isabellina is a strong candidate for SCO production from cheap agricultural wastes.5,6
Before enzymatic hydrolysis and subsequent fermentation, agricultural residues should be pretreated to crack down the recalcitrance for better enzymatic digestibility and more efficient conversion. Pretreatment is one of the most expensive single unit operation in lignocellulose-based biorefineries.7,8 It is not only one of the major contributor to high production costs, but also affects the subsequent processes owing to the inhibitors form during pretreatments. Hitherto many different pretreatments have been developed, including physical, chemical, physico-chemical, and biological methods, etc9,10. Different pretreatments have different mechanisms to break the recalcitrant lignocellulosic biomass. However, those mechanisms could be summarized as follows: removing lignin, reducing crystallinity of cellulose, hydrolyzing hemicellulose, disrupting linkage between hemicellulose and lignin, increasing specific surface areas of substrates and so on. Furthermore, different lignocellulosic biomasses have different physico-chemical characteristics,8 therefore a suitable pretreatment technology should be established for a specific lignocellulose material to increase efficiency of lignocellulose-based bioprocesses.
The biggest challenge to the SCO production from agricultural wastes is its high production cost, making it economically uncompetitive. The priority for the researches is how to reduce the costs by increasing the bioconversion efficiency. In order to achieve it, we sought efficient pretreatment and adopted the concept of on-site cellulase production since it is well known that pretreatment, enzymatic hydrolysis and cellulase production are the most expensive single unit operations in lignocellulose-based biorefineries.7
In this work, the leading pretreatments including steam explosion pretreatment, sodium hydroxide pretreatment, diluted sulfuric acid pretreatment, lime pretreatment and aqueous ammonia pretreatment were applied and compared in the bioprocess from corn stover to SCO to look for the most suitable pretreatment for corn stover in the context of SCO production. Though some of them had been compared in the enzymatic hydrolysis of corn stover,11 it's the first we compared them in the SCO production by M. isabellina from cron stover. On-site cellulase production by the mixed culture of Trichoderma reesei and Aspergillus niger6,12,13 was carried out to realize cellulase autarky due to its advantages as described by Culbertson et al.14 such as cost-saving. Another purpose of this work is to establish an efficient process from corn stover to SCO.
2. Materials and methods
2.1 Corn stover and pretreatments
Corn stover was collected from Kaifeng area, Henan Province, in the autumn of 2014. It was dried in the sun and cut to a suitable size (5–10 cm) before storage at room temperature. Prior to the pretreatments except steam explosion, corn stover was milled using a laboratory hammer mill to a size less than 2 mm. The materials after pretreatments were washed using tap water and distilled water until neutral pH. Then they were collected and stored at 4 °C. The compositions of the corn stover materials before and after pretreatments are listed in Table 1.
Table 1 Compositions of the raw material and the materials with different pretreatments
Material |
Glucan (%) |
Xylan (%) |
Lignin (%) |
Others (%) |
Aqueous ammonia pretreatment was conducted in the tandem with HCl pretreatment (Chen et al., 2009). |
Corn stover |
39.5 ± 0.8 |
14.7 ± 0.6 |
19.2 ± 0.5 |
26.6 ± 0.5 |
Steam exploded corn stover |
52.5 ± 1.0 |
7.2 ± 0.3 |
22.8 ± 0.7 |
17.5 ± 0.6 |
Sodium hydroxide pretreated corn stover |
62.9 ± 1.2 |
15.2 ± 0.5 |
8.7 ± 0.3 |
13.2 ± 0.4 |
Diluted sulfuric acid pretreated corn stover |
60.8 ± 1.0 |
5.8 ± 0.4 |
28.1 ± 0.8 |
5.3 ± 0.3 |
Lime pretreated corn stover |
54.4 ± 1.1 |
18.5 ± 0.9 |
18.2 ± 0.7 |
8.9 ± 0.3 |
Aqueous ammonia pretreated corn stovera |
63.6 ± 1.5 |
8.7 ± 0.3 |
20.9 ± 0.6 |
6.8 ± 0.4 |
2.1.1 Sodium hydroxide pretreatment. Corn stover with the size of less than 2 mm was soaked in 2% NaOH aqueous solution with a solid-to-liquid ratio of 1
:
8 (g:mL), mixed thoroughly and kept at 120 °C for 30 min.11,15
2.1.2 Steam explosion pretreatment. Corn stover with the size of 5–10 cm was steam exploded under the following conditions: temperature 200 °C, pressure 1.6 MPa, pressure maintained time 7 min, substrate loading 100 g (dry material).12,13
2.1.3 Aqueous ammonia pretreatment. Corn stover with the size of less than 2 mm was mixed with 10% NH4OH aqueous solution with a solid-to-liquid ratio of 1
:
10 (g:mL) and incubated in a shaker at 26 °C for 24 h. Then it was filtered and washed with tap water 3 times. Subsequently, it was further treated with 0.3 mol L−1 HCl at 100–108 °C for 1 h.11
2.1.4 Lime pretreatment. Corn stover with the size of less than 2 mm was mixed with calcium hydroxide with a ratio of 1
:
2.5 (g:g). Then it was added with distilled water with a water versus corn stover ratio of 10
:
1 (g:g) and kept at 120 °C for 4 h under non-oxidative condition.11
2.1.5 Diluted sulfuric acid pretreatment. Corn stover with the size of less than 2 mm was mixed with 1.5% H2SO4 aqueous solution with a solid-to-liquid ratio of 1
:
10 (g:mL) and maintained at 106–108 °C for 6 h.11
2.2 Mixed culture of T. reesei and A. niger and relevant media
T. reesei and A. niger were pre-cultured in the seed medium for 36 h and 48 h, respectively. Mixed culture of T. reesei and A. niger was performed in 250 mL Erlenmeyer flasks with a working volume of 50 mL (90% the fermentation medium for the mixed culture and 10% the total inoculums of T. reesei and A. niger). The delay time of A. niger inoculation and the inoculum ratio of T. reesei versus A. niger were 48 h and 5
:
1, respectively.12,13
The seed medium consisted of 10 g L−1 glucose, 1 g L−1 peptone, 5 mL Mandels nutrients salts solution, 2.5 mL 1 M citrate buffer, 0.05 mL Mandels trace elements solution, 0.1 g L−1 Tween 80.
The fermentation medium had a following composition (g L−1): pretreated corn stover (dry material) 30, glucose 1, (NH4)2SO4 6, KH2PO4 2, CaCl2 0.3, MgSO4 0.3, FeSO4 0.005, MnSO4 0.0016, ZnSO4 0.0014, and CoCl2 0.0037. The initial pH was adjusted to 4.8 using 1 M citrate buffer.
The media were autoclaved at 121 °C for 20 or 30 min (20 min for the medium without pretreated corn stover and 30 min for the media with pretreated corn stover).
2.3 Enzymatic hydrolysis of pretreated corn stover
The enzymatic hydrolysis of pretreated corn stover was performed in 250 mL Erlenmeyer flasks with a working volume 50 mL containing 2.5 mL 1 M citrate buffer solution (pH 4.8), 100 g L−1 pretreated corn stover (dry material), 25 FPIU g−1 glucan the cellulase from the mixed culture described above which was harvested after 5 d fermentation, and a supplementary amount of distilled water to make up 50 mL. After the crude enzyme in the fermentation broth collected by centrifugation (3000 rpm, 5 min) were added and mixed evenly, flasks were incubated in an orbital shaker (140 rpm) at 50 °C for 48 h. Samples were taken at 2, 4, 6, 12, 24, 48 h during enzymatic hydrolysis for further analyses.
2.4 Fermentation of enzymatic hydrolysates by M. isabellina
M. isabellina spores on the PDA slants were suspended with sterile water and counted by Fisher Scientific hemacytometer. The fermentation medium (50 mL) in 250 mL Erlenmeyer flasks was inoculated with 1 × 108 spores and incubated at 28 °C with a shaking of 160 rpm. Samples were taken once a day to monitor the growth of M. isabellina and the lipid accumulation during the fermentation.
Fermentation medium was composed of the enzymatic hydrolysate, 0.5 g L−1 (NH4)2SO4, 0.5 g L−1 yeast extract, 0.5 g L−1, 7 g L−1 KH2PO4, 2 g L−1 Na2HPO4, 1.5 g L−1 MgSO4·7H2O, 0.1 g L−1 CaCl2·2H2O, 0.008 g L−1 FeCl3·6H2O, 0.001 g L−1 ZnSO4·7H2O, 0.0001 g L−1 CuSO4·5H2O, 0.0001 g L−1 Co(NO3)2·H2O, 0.0001 g L−1 MnSO4·5H2O. The initial pH was adjusted to 5.5. The enzymatic hydrolysate was autoclaved at 121 °C for 30 min and the mixture of salts was sterilized by filtering through 0.22 μm membrane (Millipore, MA, USA). Then they were blended before use.
2.5 Analytical methods
2.5.1 Component analysis of raw and pretreated corn stover. The components of raw and pretreated corn stover, which were glucan, xylan and lignin, were analyzed using the method recommended by National Renewable Energy Laboratory (NREL), i.e. the NREL/TP-510-42618 procedure.
2.5.2 Enzymatic activities of cellulases. Filter paper activity (FPA) was measured using the standard method recommended by the International Union of Pure and Applied Chemistry (IUPAC) and expressed in international unit (IU).16 One IU of FPA (FPIU) was defined as the mount of enzyme required for releasing 1 μmol reducing sugars in 1 min.β-Glucosidase activity (BGA) was assayed using the method adapted from the standard FPA determination.16 The substrate was ρNPG (ρ-nitrophenyl-b-D-1,4-glucopiranoside) (Sigma-Aldrich, St. Louis, MO, USA) instead of cellobiose. The reaction mixture consisted of 0.1 mL the enzyme solution diluted properly and 0.9 mL 5 mM ρNPG solution. The reaction was conducted at 50 °C with a shaking of 80 rpm for 10 min and stopped with 2 mL 1 M Na2CO3. The product ρ-nitrophenol was measured spectrophotometrically at a wavelength of 400 nm. One IU of BGA was defined as the amount of enzyme which can produce 1 μmol ρ-nitrophenol in 1 min.
The measurements of cellobiohydrolase activity (CBA) and endoglucanase activity (EGA) were similar to the FPA assay16 except for the substrates, the reaction time, and the definition of enzymatic activity unit. The substrates for assaying CBA and EGA were microcrystalline cellulose PH101 and carboxymethyl cellulose, respectively, both of which were purchased from Sigma-Aldrich, St. Louis, MO, USA. They were used in terms of 1% (w/v) suspensions. The reaction time was 30 min. One Unit (1 U) of CBA and EGA was defined as the amount of enzyme required for producing 1 mg reducing sugars in 1 h.
The assay of xylanase activity (XLA) was similar to the standard method for FPA determination16 except for the substrate and the reaction time, which were birch xylan (Sigma-Aldrich, St. Louis, MO, USA) and 30 min, respectively. Birch xylan was used in terms of 1% (w/v) suspension. One IU of XLA was defined as the mount of enzyme required for releasing 1 μmol reducing sugars in 1 min.
2.5.3 Analysis of sugars. Sugars were measured by high-performance liquid chromatography (HPLC) using an Agilent 1100 system equipped with Bio-Rad Aminex HPX-87H (300 mm × 7.8 mm). Deionized and degassed water was used as the mobile phase at a flow rate of 0.6 mL min−1. The column temperature was fixed at 55 °C. The eluate was detected by a refractive index detector.
2.5.4 Determination of microbial lipids. The microbial cells (∼30 mg biomass in 1 mL water solution), 4.5 mL methanol, and 1 mL tridecanoic acid (internal standard) were added into a tube. The tube was capped and vortexed for 30 s. Add 0.2 mL 12 M H2SO4 into the tube and mix it using vortex. The tube was kept in 85 °C water bath for 15 min for esterification. Immediately the tube was cooled down with tap water. Add 2 mL H2O and mix using vortex. Add 2 mL hexane and mix again for fatty acid methyl esters (FAME) extraction. The hexane layer was moved to vial for analysis. The FAMEs were determined using capillary gas chromatography. A SP-2560, 100 m × 0.25 mm × 0.20 μm capillary column (Supelco) was installed on the Hewlett Packard 5890 gas chromatograph instrument equipped with a Hewlett Packard 3396 Series II integrator and a 7673 controller, a flame ionization detector, and split injection (Agilent Technologies Inc., Santa Clara, CA, USA).
2.6 Calculations
|
Enzymatic hydrolysis yield (%) = (glucose + xylose) (g) × 0.9 × 100/(glucan + xylan) (g)
| (1) |
|
Lipid content (%) = SCO (g) × 100/cell biomass (g)
| (2) |
|
Total yield of cell biomass (g g−1 corn stover) = cell biomass (g) × 100/g corn stover (g)
| (3) |
|
Total yield of SCO (g g−1 corn stover) = SCO (g) × 100/g corn stover (g)
| (4) |
|
Total productivity of cell biomass (g per day) = cell biomass (g)/total time (day)
| (5) |
|
Total productivity of SCO (g per day) = SCO (g)/total time (day)
| (6) |
3. Results and discussion
3.1 Pretreatment of corn stover
Corn stover was pretreated using steam explosion pretreatment, sodium hydroxide pretreatment, diluted sulfuric acid pretreatment, lime pretreatment and aqueous ammonia pretreatment, respectively. The compositions before and after pretreatments are listed in Table 1. Sodium hydroxide pretreated corn stover (SHPCS) had the lowest lignin content, 8.7 ± 0.3%, indicating that sodium hydroxide pretreatment removed a large fraction of lignin. Lime pretreated corn stover (LPCS) had the second lowest lignin content. Its lignin removal was weaker than sodium hydroxide pretreatment. Diluted sulfuric acid pretreated corn stover (DSAPCS) had the lowest xylan content, 5.8 ± 0.4%. This indicates diluted sulfuric acid pretreatment mainly cracked down the tough structure of corn stover by hydrolyzing hemicellulose. In addition to diluted sulfuric acid pretreatment, steam explosion and aqueous ammonia pretreatment degraded hemicellulose largely. After pretreatment, aqueous ammonia pretreated corn stover (AAPCS) had the highest glucan content (63.6 ± 1.5%). SHPCS had the second largest (62.9 ± 1.2%) and DSAPCS had the third largest (60.8 ± 1.0%).
These pretreatments are widely used in lignocellulose-based biorefineries.9,10 In subsequent work, therefore, we compared these pretreatments in SCO production from corn stover by M. isabellina.
3.2 Cellulase production from pretreated corn stover
The pretreated materials, steam exploded corn stover (SECS), SHPCS, DSAPCS, LPCS and AAPCS, were used as substrate and inducer for cellulase production by the mixed culture of T. reesei and A. niger as reported by the previous work.12,13 The results of cellulase production are shown in Fig. 1. It was found that SHPCS had the best induction for cellulase production, leading to the highest FPA which was 3.85 ± 0.23 FPIU mL−1. This suggests that sodium hydroxide pretreatment changed corn stover to let it be the best inducer. Besides, SHPCS induced the second highest BGA, 1.08 ± 0.17 IU mL−1, which was just slightly lower than the biggest BGA from SECS, 1.16 ± 0.15 IU mL−1. This inconspicuous difference could not prove anything. Therefore, we chose the SHPCS as the best substrate and inducer for cellulase production by the mixed culture of T. reesei and A. niger.
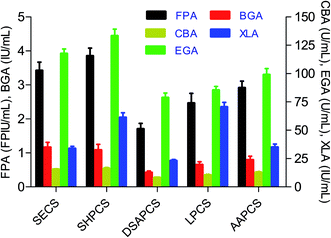 |
| Fig. 1 Enzymatic activities of the cellulases produced by the mixed culture of Trichoderma reesei and Aspergillus niger after 5 d fermentation induced by different pretreated materials including steam exploded corn stover (SECS), sodium hydroxide pretreated corn stover (SHPCS), diluted sulfuric acid pretreated corn stover (DSAPCS), lime pretreated corn stover (LPCS) and aqueous ammonia pretreated corn stover (AAPCS). The fermentation was carried out in n 250 mL Erlenmeyer flasks with 50 mL of the fermentation medium. A. niger was inoculated 48 h later than T. reesei and the inoculum ratio of T. reesei versus A. niger was 5 : 1. FPA: filter paper activity; CBA: cellobiohydrolase activity; EGA: endoglucanase activity; BGA: β-glucosidase activity; XLA: xylanase activity. Data shown are average values of triplicate samples and error bars are standard deviations. | |
These cellulases were applied to the subsequent experiments of enzymatic hydrolysis respectively, i.e. the cellulase induced by SHPCS was used in the enzymatic hydrolysis of SHPCS, the cellulase induced by SECS was used in the enzymatic hydrolysis of SECS, and so forth. The first reason is that we hope the whole process from corn stover to SCO just uses one kind of pretreatment. More than one pretreatments employed in the process signifies more single unit operations, more complicacy, and higher production cost. The second reason is that use of lignocellulosic biomass as substrate to induce cellulase production has an increased enzymatic hydrolysis specificity for the substrate itself than others.14,17,18 This is so-called on-site enzyme production for lignocellulose-based biorefineries.6,14
3.3 Enzymatic hydrolysis of pretreated corn stover
The pretreated materials, SECS, SHPCS, DSAPCS, LPCS and AAPCS, were enzymatically hydrolyzed by the cellulase induced by and produced from themselves. The results are shown in Fig. 2. The enzymatic hydrolysis of SHPCS by the cellulase produced from SHPCS was found to be the most efficient, achieving the highest yield of 87.9 ± 2.5%. It produced 61.5 g L−1 glucose and 14.8 g L−1 xylose after 48 h enzymatic hydrolysis from 100 g L−1 dry SHPCS. The second highest yield, 81.5 ± 1.2%, was from the enzymatic hydrolysis of SECS by the cellulase produced from SECS. This process produced 47.6 g L−1 glucose and 6.5 g L−1 xylose after 48 h enzymatic hydrolysis from 100 g L−1 dry SECS. Next in line was the enzymatic hydrolysis of AAPCS by the cellulase produced from AAPCS which led to a yield of 75.2 ± 2.7%, i.e. 44.8 g L−1 glucose and 8.3 g L−1 xylose from 100 g L−1 dry AAPCS. The penultimate, the enzymatic hydrolysis of LPCS by the cellulase produced from LPCS, gave rise to a yield of 66.5 ± 1.8%, producing 39.2 g L−1 glucose and 14.7 g L−1 xylose from 100 g L−1 dry LPCS. The process with the lowest yield of 48.0 ± 2.1% was the enzymatic hydrolysis of DSAPCS by the cellulase produced from DSAPCS, which released 31.4 g L−1 glucose and 4.1 g L−1 xylose from 100 g L−1 dry DSAPCS.
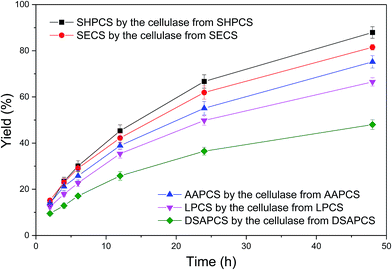 |
| Fig. 2 Enzymatic hydrolysis of different substrates using the cellulases induced by themselves. Enzymatic hydrolysis was performed in 250 mL Erlenmeyer flasks with a volume of 50 mL. The cellulase dosage was 25 FPIU g−1 dry substrate. The temperature, agitation and initial pH were 50 °C, 140 rpm and 4.8, respectively. SHPCS: sodium hydroxide pretreated corn stover; SECS: steam exploded corn stover; AAPCS: aqueous ammonia pretreated corn stover; DSAPCS: diluted sulfuric acid pretreated corn stover; LPCS: lime pretreated corn stover. Data shown are average values of triplicate samples and error bars are standard deviations. | |
Sodium hydroxide pretreatment was proven here to be the most suitable for pretreating corn stover using enzymatic hydrolysis yield as criterion, which led to the highest yield. This is probably because this pretreatment had the highest lignin removal,11 making the substrate most enzymatically digestible.9,10,19 Diluted sulfuric acid pretreatment mainly depolymerized hemicelluloses but had no effect on lignin, thus resulting in the highest lignin content. Therefore, it makes sense that it caused the lowest yield. Then we compared the effect of pretreatment on the fermentation process for SCO production.
3.4 Fermentation of enzymatic hydrolysate for SCO production
The enzymatic hydrolysates were fermented by M. isabellina to produce SCO. Only glucose and xylose were taken into consideration because other sugars were not fermentable or trace. The fermentation results are shown in Fig. 3.
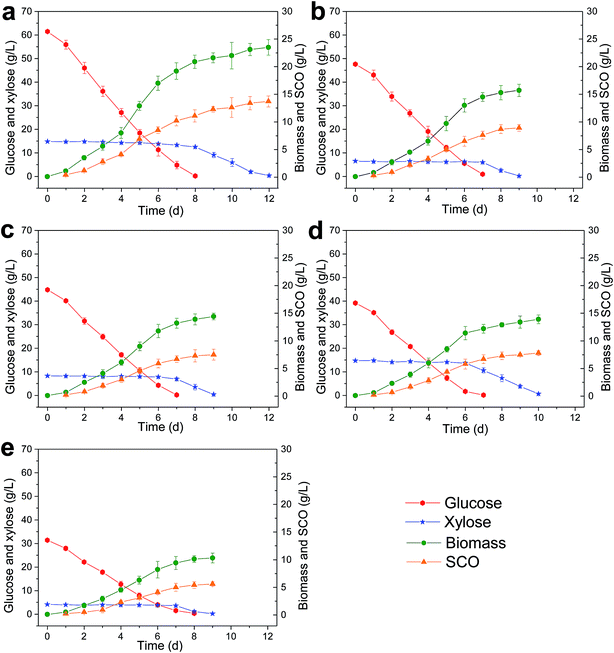 |
| Fig. 3 Time courses of SCO production by M. isabellina in the enzymatic hydrolysates of SHPCS (sodium hydroxide pretreated corn stover) (a) containing 61.5 g L−1 glucose and 14.8 g L−1 xylose, SECS (steam exploded corn stover) (b) containing 47.6 g L−1 glucose and 6.5 g L−1 xylose, AAPCS (aqueous ammonia pretreated corn stover) (c) containing 44.8 g L−1 glucose and 8.3 g L−1 xylose, LPCS (lime pretreated corn stover) (d) containing 39.2 g L−1 glucose and 14.7 g L−1 xylose, and DSAPCS (diluted sulfuric acid pretreated corn stover) (e) containing 31.4 g L−1 glucose and 4.1 g L−1 xylose. The SCO production was performed in 250 mL Erlenmeyer flasks with 50 mL of the fermentation medium inoculated with 1 × 108 spores and incubated in a rotary shaker at 160 rpm and 28 °C. Data shown are average values of triplicate samples and error bars are standard deviations. | |
The hydrolysates from the differently pretreated corn stover contained different concentrations of glucose and xylose which led to different time or productivities for the fermentation processes (Fig. 3a–e). It's in the same pattern that during the all the fermentation processes, M. isabellina fermented glucose first and xylose since then. This is normal because of glucose effect. As shown in Fig. 1a, M. isabellina spent 12 d depleting 61.5 g L−1 glucose and 14.8 g L−1 in the enzymatic hydrolysate of SHPCS and it produced 23.5 ± 1.4 g L−1 cell biomass (dry cell weight, the same below) encompassing 13.7 ± 1.0 g L−1 SCO. In the fermentation of the SECS enzymatic hydrolysate containing 47.6 g L−1 glucose and 6.5 g L−1 xylose (Fig. 3b), M. isabellina produced 15.7 ± 1.1 g L−1 cell biomass encompassing 8.9 ± 0.6 g L−1 SCO after 9 d fermentation. Fig. 3c shows the fermentation of the AAPCS enzymatic hydrolysate containing 44.8 g L−1 glucose and 8.3 g L−1 xylose and M. isabellina produced 14.4 ± 0.6 g L−1 cell biomass encompassing 7.5 ± 1.0 g L−1 SCO after 9 d fermentation. Fig. 3d presents the fermentation of the LPCS enzymatic hydrolysate involving 39.2 g L−1 glucose and 14.7 g L−1 xylose, which resulted in 13.9 ± 0.8 g L−1 cell biomass and 7.8 ± 0.4 g L−1 SCO after 10 d fermentation. In the fermentation of DSAPCS enzymatic hydrolysate that had 31.4 g L−1 glucose and 4.1 g L−1 xylose (Fig. 3e), M. isabellina accumulated 10.3 ± 0.9 g L−1 cell biomass harboring 5.6 ± 0.4 g L−1 SCO after 9 d fermentation.
It was inconclusive here that sodium hydroxide pretreatment was the best approach just based on the fermentation of hydrolysates for SCO production because those fermentation processes were incomparable when the fermentable sugars were in different concentrations. Therefore, we would compare the entire processes from corn stover to SCO and the relevant details to find out the most suitable pretreatment among the pretreatment methods we adopted in the work for SCO production from corn stover by M. isabellina.
3.5 Comparison of the processes from corn stover to SCO
Fig. 4 illustrates the technological process flows from corn stover to SCO and the details of each process are given in Table 2. As shown in Fig. 4, we developed different processes from corn stover to SCO involving different pretreatments. It was found that sodium hydroxide pretreatment used the smallest amount of starting material, 146.2 g corn stover, but produced the largest amounts of cell biomass and SCO, 23.5 g and 13.7 g respectively. This means Process a had the highest yields and productivities, as listed in Table 2. Thus, it's conclusive that sodium hydroxide pretreatment was the best suited for SCO production from corn stover by M. isabellina among the five pretreatment methods compared in this work. The superiority of sodium hydroxide pretreatment over the others was ascribed to its most potent capability of lignin removal and less formation of inhibitors.11,20 Lignin hinders the contact between cellulase and its substrate cellulose, as well as the contact between hemicellulases and its substrate hemicellulose.21 In addition, the presence of lignin causes nonproductive enzyme adsorption.19,22 The inhibitors derived from pretreatments are inhibitory or even toxic to downstream enzymes and organisms.9,10 Thus, it is reasonable that sodium hydroxide pretreatment performed the best. These five pretreatments are widely used in pretreating lignocellulosic materials,6,8,9,11,15,23–25 indicating that these are leading pretreatment technologies. Sodium hydroxide pretreatment, therefore, should be the desirable option when carrying out the production of lignocellulosic SCO.
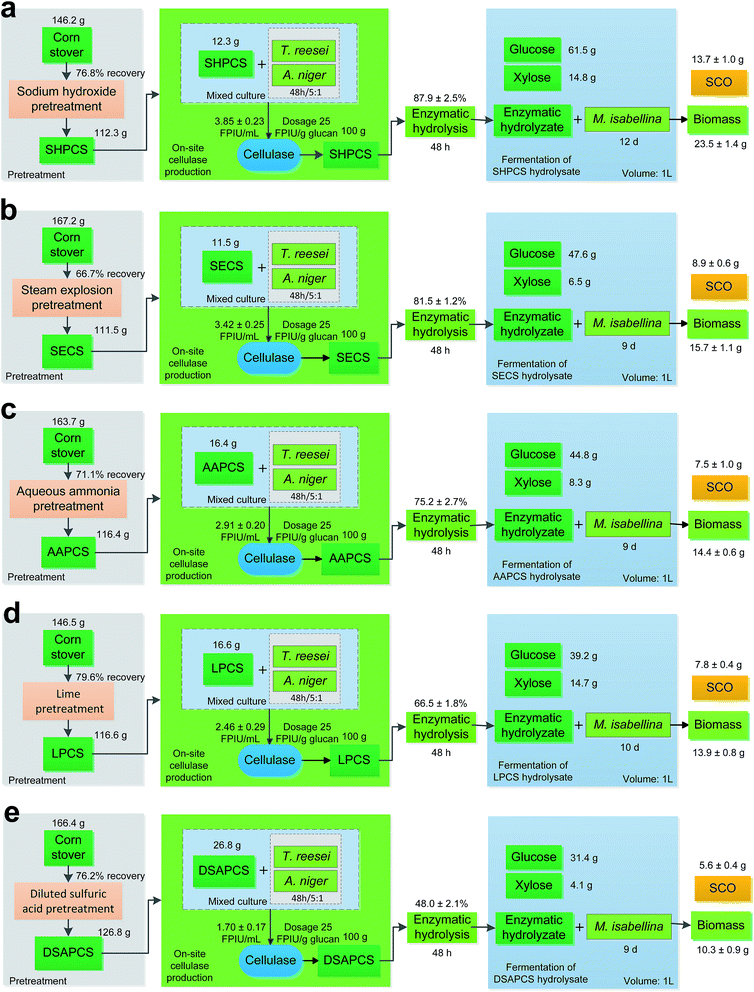 |
| Fig. 4 Comparison of different processes from corn stover to SCO involving different pretreatments including sodium hydroxide pretreatment (a), steam explosion pretreatment (b), aqueous ammonia pretreatment (c), lime pretreatment (d) and diluted sulfuric acid pretreatment (e). SHPCS: sodium hydroxide pretreated corn stover; SECS: steam exploded corn stover; AAPCS: aqueous ammonia pretreated corn stover; DSAPCS: diluted sulfuric acid pretreated corn stover; LPCS: lime pretreated corn stover. Detailed information is given here to facilitate the comparison. | |
Table 2 Comparison of different processes from corn stover to single cell oil (SCO)
Process a used sodium hydroxide pretreatment; b steam explosion pretreatment; c aqueous ammonia pretreatment; d lime pretreatment; e diluted sulfuric acid pretreatment. Total time was the sum of enzymatic hydrolysis time and fermentation time. |
Processa |
a |
b |
c |
d |
e |
Corn stover (g) |
146.2 |
167.2 |
163.7 |
146.5 |
166.4 |
Pretreated corn stover (g) |
112.3 |
111.5 |
116.4 |
116.6 |
126.8 |
Pretreated corn stover for cellulase production (g) |
12.3 |
11.5 |
16.4 |
16.6 |
26.8 |
Pretreated corn stover for saccharification (g) |
100 |
100 |
100 |
100 |
100 |
Glucose (g) |
61.5 |
47.6 |
44.8 |
39.2 |
31.4 |
Xylose |
14.8 |
6.5 |
8.3 |
14.7 |
4.1 |
Cell biomass (g dry cell weight) |
23.5 |
15.7 |
14.4 |
13.9 |
10.3 |
SCO (g) |
13.7 |
8.9 |
7.5 |
7.8 |
5.6 |
Lipid content (%) |
58.3 |
56.7 |
52.1 |
56.1 |
54.4 |
Total yield of cell biomass (g g−1 corn stover) |
0.161 |
0.094 |
0.088 |
0.095 |
0.062 |
Total yield of SCO (g g−1 corn stover) |
0.094 |
0.053 |
0.046 |
0.053 |
0.034 |
Total time (d)b |
14 |
11 |
11 |
12 |
11 |
Total productivity of cell biomass (g per day) |
1.68 |
1.43 |
1.31 |
1.16 |
0.94 |
Total productivity of SCO (g per day) |
0.98 |
0.81 |
0.68 |
0.65 |
0.51 |
In the comparison of the processes from corn stover to SCO, it was found that the proper order from good to bad was sodium hydroxide pretreatment, steam explosion pretreatment, lime pretreatment, aqueous ammonia pretreatment, and diluted sulfuric acid pretreatment. This order is not exactly the same as that concluded from the enzymatic hydrolysis experiment (Fig. 2). Though aqueous ammonia pretreatment was obviously better than lime pretreatment in the enzymatic hydrolysis, the result was contrary in the fermentation for SCO production where the yields of Process d with lime pretreatment were higher than those of Process c with aqueous ammonia pretreatment. This suggests that enzymatic hydrolysis yield could not adopted as single criterion to evaluate pretreatment and the effects on subsequent bioprocesses, although the orders were grosso modo the same—sodium hydroxide pretreatment the first and diluted sulfuric acid pretreatment the last. Hence, we should take both enzymatic hydrolysis and fermentation into consideration and, more importantly, emphasize the whole process from raw material to product. The contrary results of lime pretreatment and aqueous ammonia pretreatment in enzymatic hydrolysis and in the whole process may be caused by the different recovery rates of pretreatments and different contents of glucan and xylan after pretreatment. Moreover, the inhibitors derived from pretreatment and their influence on subsequent bioprocesses23,26 may fuzz up the results. Though we washed pretreated corn stover until neutral pH, there were still some remanent inhibitors (data not shown) which could be released gradually during enzymatic hydrolysis and cause detrimental impact on fermentation. Further work should be conducted to elucidate the phenomenon.
This work highlights sodium hydroxide pretreatment as the best among the five pretreatments. Moreover, the efficient process from corn stover to SCO was established successfully here. In the future, we will compare it with novel or more advanced pretreatments27–29 in a holistic way, and improve it for more perfect pretreatment of corn stover.30,31
4. Conclusions
Mixed culture of T. reesei and A. niger was employed to achieve cellulase autarky, which was proven more efficient than other cellulases in the enzymatic saccharification of corn stover. The five pretreatments inducing sodium hydroxide pretreatment, steam explosion pretreatment, aqueous ammonia pretreatment, lime pretreatment and diluted sulfuric acid pretreatment were compared comprehensively in the SCO production by M. isabellina from corn stover. Among the five pretreatments, sodium hydroxide pretreatment was proved to be the best one, leading to the highest yield of SCO and the highest process efficiency from corn stover.
Conflicts of interest
There are no conflicts of interest to declare.
Acknowledgements
This work was financially supported by the first-class General Financial Grant (2016M600815) and the second-class General Financial Grant (2017M613213) from the China Postdoctoral Science Foundation, Youth Fund from Jiangsu Province (BK20150130), the Fundamental Research Funds (2452017157), the Fund for Doctoral Scientific Research (Z109021632) and the Start-up Fund for Talent Introduction (Z111021602) from Northwest A&F University.
References
- X. Fang, Y. Shen, J. Zhao, X. Bao and Y. Qu, Bioresour. Technol., 2010, 101, 4814–4819 CrossRef CAS PubMed.
- D. Gao, J. Zeng, Y. Zheng, X. Yu and S. Chen, Bioresour. Technol., 2013, 133, 315–321 CrossRef CAS PubMed.
- R. Subramaniam, S. Dufreche, M. Zappi and R. Bajpai, J. Ind. Microbiol. Biotechnol., 2010, 37, 1271–1287 CrossRef CAS PubMed.
- A. Chatzifragkou, S. Fakas, M. Galiotou-Panayotou, M. Komaitis, G. Aggelis and S. Papanikolaou, Eur. J. Lipid Sci. Technol., 2010, 112, 1048–1057 CrossRef CAS.
- Y. Zheng, X. Yu, J. Zeng and S. Chen, Biotechnol. Biofuels, 2012, 5, 50 CrossRef CAS PubMed.
- H. Fang, C. Zhao and S. Chen, Bioresour. Technol., 2016, 216, 988–995 CrossRef CAS PubMed.
- C. E. Wyman, Trends Biotechnol., 2007, 25, 153–157 CrossRef CAS PubMed.
- P. Alvira, E. Tomas-Pejo, M. Ballesteros and M. J. Negro, Bioresour. Technol., 2010, 101, 4851–4861 CrossRef CAS PubMed.
- M. Galbe and G. Zacchi, Biomass Bioenergy, 2012, 46, 70–78 CrossRef CAS.
- B. Yang and C. E. Wyman, Biofuels, Bioprod. Biorefin., 2008, 2, 26–40 CrossRef CAS.
- M. Chen, J. Zhao and L. Xia, Biomass Bioenergy, 2009, 33, 1381–1385 CrossRef CAS.
- H. Fang, C. Zhao, X.-Y. Song, M. Chen, Z. Chang and J. Chu, Biotechnol. Bioprocess Eng., 2013, 18, 390–398 CrossRef CAS.
- H. Fang, C. Zhao and X. Y. Song, Bioresour. Technol., 2010, 101, 4111–4119 CrossRef CAS PubMed.
- A. Culbertson, M. Jin, L. da Costa Sousa, B. E. Dale and V. Balan, RSC Adv., 2013, 3, 25960 RSC.
- H. Fang, C. Zhao, Q. Kong, Z. Zou and N. Chen, Energy, 2016, 116, 177–189 CrossRef CAS.
- T. K. Ghose, Pure Appl. Chem., 1987, 59, 257–268 CAS.
- T. Juhász, Z. Szengyel, K. Réczey, M. Siika-Aho and L. Viikari, Process Biochem., 2005, 40, 3519–3525 CrossRef.
- V. Rana, A. D. Eckard, P. Teller and B. K. Ahring, Bioresour. Technol., 2014, 154, 282–289 CrossRef CAS PubMed.
- U. Bornscheuer, K. Buchholz and J. Seibel, Angew. Chem., 2014, 53, 10876–10893 CrossRef CAS PubMed.
- Q. Z. Zhang and W. M. Cai, Biomass Bioenergy, 2008, 32, 1130–1135 CrossRef CAS.
- C. Sanchez, Biotechnol. Adv., 2009, 27, 185–194 CrossRef CAS PubMed.
- J. Börjesson, M. Engqvist, B. Sipos and F. Tjerneld, Enzyme Microb. Technol., 2007, 41, 186–195 CrossRef.
- X. Yu, Y. Zheng, K. M. Dorgan and S. Chen, Bioresour. Technol., 2011, 102, 6134–6140 CrossRef CAS PubMed.
- B. C. Saha, L. B. Iten, M. A. Cotta and Y. V. Wu, Biotechnol. Prog., 2005, 21, 816–822 CrossRef CAS PubMed.
- Y. Sun and J. J. Cheng, Bioresour. Technol., 2005, 96, 1599–1606 CrossRef CAS PubMed.
- X. Yu, J. Zeng, Y. Zheng and S. Chen, Process Biochem., 2014, 49, 457–465 CrossRef CAS.
- G. Banerjee, S. Car, J. S. Scott-Craig, D. B. Hodge and J. D. Walton, Biotechnol. Biofuels, 2011, 4, 16 CrossRef CAS PubMed.
- C. Wan and Y. Li, Biotechnol. Adv., 2012, 30, 1447–1457 CrossRef CAS PubMed.
- V. B. Agbor, N. Cicek, R. Sparling, A. Berlin and D. B. Levin, Biotechnol. Adv., 2011, 29, 675–685 CrossRef CAS PubMed.
- M. A. Kabel, G. Bos, J. Zeevalking, A. G. Voragen and H. A. Schols, Bioresour. Technol., 2007, 98, 2034–2042 CrossRef CAS PubMed.
- D. Mishima, M. Tateda, M. Ike and M. Fujita, Bioresour. Technol., 2006, 97, 2166–2172 CrossRef CAS PubMed.
|
This journal is © The Royal Society of Chemistry 2017 |