DOI:
10.1039/C7RA11414A
(Paper)
RSC Adv., 2017,
7, 52496-52502
Bifunctional N-heterocyclic carbene ligands for Cu-catalyzed direct C–H carboxylation with CO2†
Received
16th October 2017
, Accepted 7th November 2017
First published on 13th November 2017
Abstract
Diethylene glycol-functionalized imidazo[1,5-a]pyridin-3-ylidenes (DEG-ImPy) have been developed as bifunctional N-heterocyclic carbene ligands. The DEG-ImPy Cu(I) complexes efficiently catalyzed the direct C–H carboxylation of benzoxazole with CO2, showing higher isolated yields than those with the N,N′-(2,6-diisopropylphenyl)imidazolylidene Cu catalyst.
Introduction
The use of N-heterocyclic carbenes (NHCs) as strong σ-donating ligands has become increasingly popular in homogeneous catalysis.1 Numerous structural variations on the prototypical imidazolylidene skeleton have been used to modulate the electronic and steric properties of NHC ligands. Imidazo[1,5-a]pyridin-3-ylidene (ImPy) ligands, first developed by Glorius2 and Lassaletta3 in 2005, are a rigid bicyclic variant of NHCs. ImPy ligands are strong σ-donors3,4 as the extended π-system can increase the electron density at the carbene center.5 Substituents on the bicyclic ImPy can be projected into the metal coordination sphere, often resulting in bonding interactions with the metal.3,6 Synthesis of ImPy precursors is concise and often allows late-stage incorporation of diverse functional substituents.7 Owing to this feature, a number of ImPy-metal catalysts have been developed for various organic transformations (Fig. 1).8 We envision that ImPy ligands could serve as a versatile framework for bifunctional NHC ligands.9 Polyether units such as polyethylene glycol (PEG) are known as a CO2-philic building block and have been utilized in ionic liquids for CO2 capture10 and as catalysts for CO2 conversion.11 Thus we were wondering whether introduction of diethylene glycol unit (DEG) into the ligand scaffold could render the ImPy bifunctional. Herein, we report the synthesis of diethylene glycol-functionalized imidazo[1,5-a]pyridin-3-ylidene (DEG-ImPy) copper complexes and their application in direct C–H carboxylation of benzoxazoles with CO2. DEG-ImPy Cu exhibits higher catalytic activity than non-functionalized NHC–Cu catalysts.
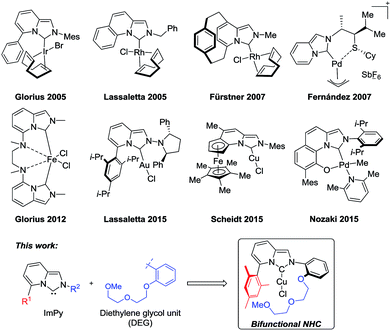 |
| Fig. 1 Representative examples of metal complexes containing imidazo[1,5-a]pyridin-3-ylidene (ImPy) ligands. | |
Results and discussion
Synthesis of ligands and catalysts
Synthesis of imidazopyridinium salts (4a–4g) was accomplished following a modified procedure of the reported method (Scheme 1). 6-Mesitylpicolinaldehyde (3) was prepared by Bouveault aldehyde synthesis,12 followed by Suzuki–Miyaura coupling.13 ImPy precursors (4a–4g) were then synthesized from the functionalized anilines, formalin, and aldehyde 3 by Aron's method.7 Among the synthesized ImPy ligands, imidazopyridinium salts (4a–4c, 4g) were converted to the corresponding ImPy–copper(I) complexes (5a–5c, 5g) by the reaction with copper chloride(I) in the presence of sodium tert-butoxide or through Ag transmetalation in reasonable isolated yields.
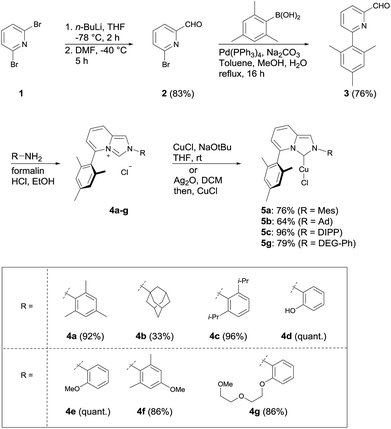 |
| Scheme 1 Synthesis of DEG-ImPy ligands and ImPy–Cu(I) complexes. | |
X-ray crystallography
The structure of ImPy–Cu complex 5a and 5g were determined by X-ray diffraction analysis (Fig. 2). As with recently reported ImPy–copper complexes,8j geometry of both complexes is linear; C(1)–Cu(1)–Cl(1) 173.5(1)° (5a) and 173.7(4)° (5g). In case of 5g, the mesityl group is in close proximity to the Cu metal center as the observed atom distance Cu(1)–C(8) is shorter (2.887 Å) than that of 5a (2.957 Å). The aryl group on the nitrogen atom in 5a is oriented nearly perpendicular to the NHC plane, resulting in a dihedral angle of 80.64° whereas the dihedral angle between the ImPy plane and the DEG-phenyl in 5g is 50.84°.
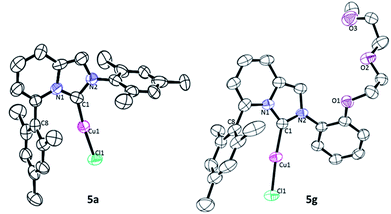 |
| Fig. 2 ORTEP of molecular structure of ImPy–Cu complexes. Thermal ellipsoids are drawn at the 50% probability level. Hydrogen atoms have been omitted for clarity. Selected bond lengths (Å) and bond angles (°): 5a Cu(1)–C(1) = 1.83(1), N(1)–C(1) = 1.38(1), C(1)–N(2) = 1.39(1), Cu(1)–Cl(1) = 2.135(5); N(1)–C(1)–N(2) = 102.4(8), C(1)–Cu(1)–Cl(1) = 173.7(4); 5g Cu(1)–C(1) = 1.83(1), N(1)–C(1) = 1.38(1), C(1)–N(2) = 1.39(1), Cu(1)–Cl(1) = 2.135(5); N(1)–C(1)–N(2) = 103.5(3), C(1)–Cu(1)–Cl(1) = 173.5(1). | |
Catalytic properties of ImPy–Cu complexes
With the various ImPy ligands in hand, the catalytic activities of the ImPy–Cu complexes were evaluated in direct C–H carboxylation of benzoxazole using CO2 (Table 1). Synthetic utilization of carbon dioxide (CO2) as a sustainable C1 feedstock has attracted much attention recently.14 Catalytic direct C–H carboxylation with CO2 is an atom-economical transformation that affords carboxylic acid derivatives. Various C–H bonds of alkynes,15 alkenes/arenes,16,17 and alkanes,18 can be substituted with CO2H. Coinage–metal complexes with NHC ligands are efficient catalysts for direct C–H carboxylation. Following the procedure reported in the literature,16c initial studies were carried out using isolated NHC–Cu complexes (entries 1–4). ImPy–Cu complex with a 2,6-diisopropyl phenyl substituent (5c) showed higher catalytic activity in producing the corresponding ester than IPrCuCl under the same conditions (entry 4 vs. entry 1). It was examined whether the catalyst could be generated in situ from CuCl and NHC·HCl salt under the same reaction conditions. In most cases, in situ-formed catalysts from CuCl and NHC ligands showed better yields than the isolated Cu complexes (entries 5–7 vs. entries 1–3). Note that in situ-generated Cu catalysts from alkoxy-functionalized ImPy ligands exhibited very good yields (entries 10–12). While the hydroxy group (4d) was rather detrimental (entry 9), a methoxy group (4e and 4f) resulted in increased yield (entries 10 and 11). ImPy ligand bearing a diethylene glycol moiety (4g, DEG-ImPy) exhibited excellent activity and afforded the desired product in quantitative yield (entry 12).
Table 1 Optimization of the C–H carboxylation conditionsa
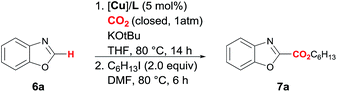
|
Entry |
Metal salt |
Ligand |
Yield of 7ab (%) |
Reaction conditions: benzoxazole (0.5 mmol), CuCl (5 mol%), ligand (5 mol%), KOtBu (1.15 equiv.), THF (2.5 mL), CO2 (1 atm), 80 °C, 14 h, then evaporation of THF under vacuum, DMF (2.5 mL), C6H13I (1.0 mmol), 80 °C, 6 h. Yields of the isolated product (average of two runs). [(IPr)CuCl] = 1,3-bis(2,6-di-isopropylphenyl) imidazolium copper(I) chloride. |
1 |
IPr-CuClc |
— |
80 |
2 |
5a |
— |
52 |
3 |
5b |
— |
63 |
4 |
5c |
— |
95 |
5 |
CuCl |
IPr·HCl |
84 |
6 |
CuCl |
4a |
95 |
7 |
CuCl |
4b |
95 |
8 |
CuCl |
4c |
81 |
9 |
CuCl |
4d |
44 |
10 |
CuCl |
4e |
92 |
11 |
CuCl |
4f |
94 |
12 |
CuCl |
4g |
99 |
Using the optimal DEG-ImPy ligand (4g), the heteroarene substrate scope of the direct C–H carboxylation was examined under the same conditions (Scheme 2). Overall, reactions with a variety of substituted benzoxazoles proceeded smoothly. Methyl-substituted benzoxazoles (7b–7d), regardless of the position of methyl group on the heterocyclic ring, gave high yields (92–98%). From the reactions with benzoxazoles containing electron donating (R = OMe, Ph) and withdrawing group such as halide (R = Cl, Br), the corresponding esters were obtained with yields of over 90%. The reaction also works for other heteroarene compounds, as the reaction with 2-phenyl-1,3,4-oxadizole afforded product 7j with an 83% yield. However, N-methylbenzoimidazole afforded the product 7k with a 6% yield.
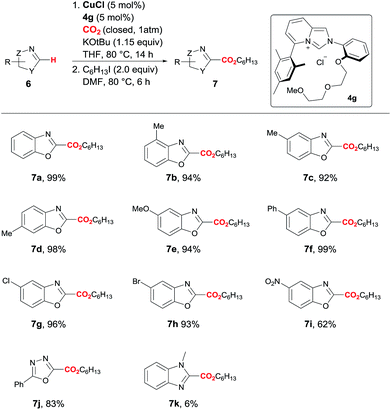 |
| Scheme 2 Substrate scopes of heteroarenes. | |
As shown in Scheme 3, the Cu catalyst with DEG-ImPy·HCl (4g) exhibits superior catalytic activity than that with IPr·HCl as well as plain ImPy·HCl (4a). Use of ImPy ligand and introduction of a potential cation-binding DEG unit in the ligand framework resulted in improving the catalytic activity in the direct C–H carboxylation with CO2.
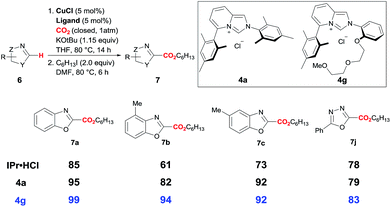 |
| Scheme 3 Ligand comparison: isolated yields of the products from direct C–H carboxylation with CO2. | |
Conclusions
Diethylene glycol-functionalized imidazo[1,5,a] pyridin-3-ylidenes (DEG-ImPy) ligands have been developed as a bifunctional NHC ligand. Cu catalyst generated in situ with the DEG-ImPy·HCl salts efficiently catalyzed the direct C–H carboxylation of various heterocyclic compounds with CO2, resulting in higher yields than those with the imidazolylidene carbene ligand (IPr). Further studies of DEG-ImPy ligands relating to cation-binding capabilities as well as application in other catalysis are currently under way in our laboratory.
Experimental sections
General remarks
All reactions were carried out under an inert argon atmosphere using the Schlenk technique or a glovebox. All reactions using CO2 were conducted in a 30 mL Schlenk flask equipped with Teflon-valve. Nuclear magnetic resonance (NMR) spectra were recorded on a JEOL spectrometer, operating at 400 MHz or 300 MHz for 1H NMR and at 100 MHz or 75 MHz for 13C NMR. All chemical shifts for 1H and 13C NMR spectroscopy were assigned to residual signals from CDCl3 (1H) at 7.26 ppm and (13C) at 77.16 ppm, CD2Cl2 (1H) at 5.32 ppm and (13C) at 53.84 ppm, or DMSO-d6 (1H) at 2.50 ppm and (13C) at 39.52 ppm. High resolution GC mass spectra were recorded using a JEOL JMS-700 MStation mass spectrometer. Infrared spectra were obtained on a Nicolet iS10 FT-IR spectrometer with an ATR unit and recorded in wave numbers (cm−1).
Materials
Tetrahydrofuran (THF), dichloromethane (CH2Cl2), N,N-dimethylformamide (DMF) and diethyl ether (Et2O) were dried under a positive pressure of dry nitrogen using a J. C. Meyer Solvent Purification System prior to use. Toluene and n-hexane were distilled from calcium hydride and ethanol was dried over 4 Å molecular sieves. Unless specified, all the other chemicals were purchased from Sigma-Aldrich Co., Acros Organics, TCI, Alfa Aesar, and Strem Chemicals Inc. and were used as received without further purification. Commercial carbon dioxide (99.99%) was purchased by Sinil Gas Co. and used without further purification. Benzoxazole, 5-methylbenzoxazole, and 5-chlorobenzoxazole were purchased and purified by flash column chromatography. Other benzoxazole derivatives19 and oxadiazole20 were synthesized as reported previously. 6-Bromopicolinaldehyde (2),12 6-mesitylpicolinaldehyde (3),13 4-Methoxy-2,6-dimethylaminine,21 IPr·HCl,22 and (IPr)CuCl23 were prepared according to the literature.
Catalyst preparation
2,5-Dimesityl imidazo[1,5-a]pyridinium chloride (4a). The procedure was modified from a report by Aron et al.7 2,4,6-Trimethylaniline (84.8 μL, 0.604 mmol), 6-mesitylpicolinaldehyde 3 (136 mg, 0.604 mmol), formalin (65.0 μL, 0.900 mmol) and 1.25 M HCl in EtOH (546 μL, 0.660 mmol) were added to dry EtOH (1.20 mL) and stirred at room temperature for 12 hours. The crude product was purified by flash column chromatography (CH2Cl2
:
MeOH 10
:
1). The product was collected by re-precipitation using Et2O and CH2Cl2 to obtain a product 4a (216 mg, 92%) as an ivory powder. 1H NMR (400 MHz, CDCl3): δ 9.30 (s, 1H), 8.87 (d, J = 9.4 Hz, 1H), 7.93 (s, 1H), 7.46 (dd, J = 9.4, 6.9 Hz, 1H), 7.05 (m, 3H), 6.98 (s, 2H), 2.34 (s, 3H), 2.32 (s, 3H), 2.02 (s, 6H), 1.97 (s, 6H) ppm; 13C NMR (75 MHz, CDCl3): δ 141.7, 141.6, 136.8, 133.7, 133.3, 132.2, 130.9, 129.7, 129.6, 126.3, 125.6, 121.3, 120.4, 120.3, 118.8, 21.2, 21.0, 19.1, 17.2 ppm; IR (ATR): ν = 3058, 2919, 1652, 1610, 1553, 1154, 1034, 844, 829, 731, 679 cm−1; HR-MS (EI): calcd for C25H27N2 [M]+ 355.2174 found 355.2170.
2-((1S,3R)-Adamantanyl)-5-mesityl-imidazo[1,5-a]pyridinium chloride (4b). With the same method used in the synthesis of 4a, 4b (33%) was obtained as a white powder from 1-adamantylamine and 6-mesitylpicolinaldehyde 3. 1H NMR (400 MHz, CDCl3): δ 9.65 (s, 1H), 8.32 (d, J = 9.3 Hz, 1H), 7.94 (s, 1H), 7.29 (dd, J = 9.4, 6.8 Hz, 1H), 7.07 (s, 2H), 6.89 (d, J = 6.8 Hz, 1H), 2.38 (s, 3H), 2.29 (s, 9H), 1.99 (s, 6H), 1.77 (m, 6H) ppm; 13C NMR (75 MHz, CDCl3): δ 141.4, 137.2, 133.5, 131.6, 129.7, 126.6, 124.6, 119.7, 119.5, 117.8, 115.6, 62.5, 43.1, 35.2, 29.6, 21.4, 19.5 ppm; IR (ATR): ν = 3065, 2999, 2915, 2855, 1651, 1612, 1550, 1454, 1308, 1143, 1103, 854, 814, 714, 663 cm−1; HR-MS (EI): calcd for C26H31N2 [M]+ 371.2487 found 371.2488.
2-(2,6-Diisopropylphenyl)-5-mesityl imidazo[1,5-a]pyridinium chloride (4c). With the same method used in the synthesis of 4a, 4c (96%) was obtained as an ivory powder from 2,6-diisopropylaniline and 6-mesitylpicolinaldehyde 3. 1H NMR (400 MHz, CDCl3): δ 9.35 (s, 1H), 9.02 (m, 1H), 7.91 (s, 1H), 7.57–7.53 (m, 2H), 7.31 (m, 2H), 7.13 (d, J = 6.9 Hz, 1H), 7.07 (s, 2H), 2.35 (s, 3H), 2.09 (m, 2H), 2.03 (s, 6H), 1.25 (d, J = 6.8 Hz, 6H), 1.04 (d, J = 6.9 Hz, 6H) ppm; 13C NMR (100 MHz, CDCl3): δ 144.9, 141.8, 137.0, 133.0, 132.9, 132.5, 132.2, 130.7, 129.7, 126.5, 125.9, 124.7, 121.5, 120.8, 120.6, 28.8, 24.7, 23.9, 21.3, 19.1 ppm; IR (ATR): ν = 3073, 3041, 2958, 2924, 2867, 1652, 1551, 1449, 1300, 1172, 1109, 853, 799, 754, 681 cm−1; HR-MS (EI): calcd for C28H33N2 [M]+ 397.2643 found 397.2648.
2-(2-Hydroxyphenyl)-5-mesityl-2H-imidazo[1,5-a]pyridinium chloride (4d). Compound 4d was prepared analogously to 4a. 4d was synthesized from 2-aminophenol and 6-mesitylpicolinaldehyde 3. The crude product was purified by recrystallization using Et2O and CH2Cl2 to obtain a product 4d (quant.) as an ivory powder. 1H NMR (400 MHz, DMSO-d6): δ 11.46 (br s, 1H), 9.38 (s, 1H), 8.70 (s, 1H), 8.01 (d, J = 9.3 Hz, 1H), 7.59 (d, J = 7.9 Hz, 1H), 7.47 (dd, J = 9.3, 7.0 Hz, 1H), 7.39 (m, 2H), 7.18 (d, J = 6.8 Hz, 1H), 7.10 (s, 2H), 6.98 (m, 1H), 2.33 (s, 3H), 2.03 (s, 6H) ppm; 13C NMR (100 MHz, DMSO-d6): δ 151.1, 139.8, 137.1, 133.1, 131.4, 129.7, 128.9, 127.0, 126.6, 125.8, 125.1, 122.5, 119.3, 119.1, 117.4, 117.3, 115.9, 20.8, 18.9 ppm; IR (ATR): ν = 3179, 3166, 3179, 2914, 2688, 2571, 1654, 1607, 1551, 1518, 1464, 1350, 1227, 1173, 805, 752, 654 cm−1; HR-MS (FAB): calcd for C22H21N2O [M]+ 329.1654 found 329.1656.
5-Mesityl-2-(2-methoxyphenyl)-2H-imidazo[1,5-a]pyridinium chloride (4e). With the same method used in the synthesis of 4d, 4e (quant.) was obtained as a white powder from 2-methoxyaniline and 6-mesitylpicolinaldehyde 3. 1H NMR (400 MHz, CDCl3): δ 9.46 (d, J = 6.0 Hz, 1H), 8.58 (dd, J = 9.3, 3.8 Hz, 1H), 8.27 (s, 1H), 7.90 (m, 1H), 7.50 (t, J = 8.4 Hz, 1H), 7.35 (t, J = 9.3 Hz, 1H), 7.18 (t, J = 9.2 Hz, 1H), 7.08 (m, 3H), 6.97 (d, J = 6.8 Hz, 1H), 3.82 (s, 3H), 2.37 (s, 3H), 2.07 (s, 6H) ppm; 13C NMR (100 MHz, CDCl3): δ 151.5, 141.4, 137.1, 133.1, 132.4, 131.1, 129.5, 126.6, 126.4, 124.9, 123.4, 122.3, 122.1, 119.9, 119.8, 118.0, 112.6, 56.4, 21.2, 19.3 ppm; IR (ATR): ν = 3187, 2995, 2918, 2858, 1654, 1604, 1556, 1518, 1455, 1261, 1130, 1022, 850, 760, 654 cm−1; HR-MS (FAB): calcd for C23H23N2O [M]+ 343.1810 found 343.1802.
5-Mesityl-2-(4-methoxy-2,6-dimethylphenyl)-2H-imidazo[1,5-a]pyridinium chloride (4f). With the same method used in the synthesis of 4a, 4f (86%) was obtained a white powder from 4-methoxy-2,6-dimethylaniline and 6-mesitylpicolinaldehyde 3. 1H NMR (400 MHz, CD2Cl2): δ 9.00 (s, 1H), 8.54 (d, J = 9.4 Hz, 1H), 8.15 (s, 1H), 7.49 (dd, J = 9.4, 6.9 Hz, 1H), 7.10 (d, J = 6.8 Hz, 1H), 7.06 (s, 2H), 6.71 (s, 2H), 3.78 (s, 3H), 2.32 (s, 3H), 2.02 (s, 6H), 1.97 (s, 6H) ppm; 13C NMR (100 MHz, CD2Cl2): δ 161.2, 141.7, 137.3, 136.0, 133.8, 132.3, 129.7, 126.7, 126.6, 126.0, 122.6, 120.6, 119.7, 118.9, 114.3, 55.9, 21.2, 19.3, 17.8 ppm; IR (ATR): ν = 3126, 3021, 2955, 2914, 2871, 1651, 1596, 1548, 1494, 1457, 1321, 1295, 1215, 1195, 1178, 1081, 1059, 1034, 868, 849, 818, 686 cm−1; HR-MS (FAB): calcd for C25H27N2 O [M]+ 371.2123 found 371.2144.
5-Mesityl-2-(2-(2-(2-methoxyethoxy)ethoxy)phenyl)-2H-imidazo[1,5-a]pyridinium chloride (4g). With the same method used in the synthesis of 4a, 4g (86%) was obtained as a white powder from 2-(2-(2-methoxyethoxy)ethoxy)aniline 9 and 6-mesitylpicolinaldehyde 3. 1H NMR (400 MHz, CDCl3): δ 9.44 (s, 1H), 8.55 (s, 1H), 8.48 (d, J = 9.4 Hz, 1H), 7.91 (d, J = 8.0 Hz, 1H), 7.46 (t, J = 7.6 Hz, 1H), 7.35 (dd, J = 9.3, 6.8, Hz, 1H), 7.17 (t, J = 7.7 Hz, 1H), 7.10 (d, J = 8.5 Hz, 1H), 7.05 (s, 2H), 6.94 (d, J = 6.8 Hz, 1H), 4.22 (t, J = 4.5 Hz, 2H), 3.64 (t, J = 5.3 Hz, 2H), 3.41 (m, 2H), 3.30 (m, 2H), 3.17 (s, 3H), 2.35 (s, 3H), 2.05 (s, 6H) ppm; 13C NMR (100 MHz, CDCl3): δ 150.4, 141.1, 137.1, 133.2, 132.0, 130.8, 129.3, 126.4, 126.3, 124.9, 123.8, 122.7, 122.2, 119.8, 119.2, 117.4, 114.3, 71.3, 69.7, 68.3, 68.1, 58.6, 21.1, 19.3 ppm; IR (ATR): ν = 3371, 3182, 2975, 2914, 1654, 1603, 1556, 1516, 1459, 1312, 1295, 1261, 1209, 1131, 1110, 851, 754, 654 cm−1; HR-MS (FAB): calcd for C27H31N2O3 [M]+ 431.2335 found 431.2330.
(2,5-Dimesityl imidazo[1,5-a]pyridinyl)copper(I) chloride (5a). 2,5-Dimesityl imidazo[1,5-a]pyridinium chloride 4a (39.1 mg, 0.100 mmol), copper chloride(I) (9.90 mg, 0.100 mmol), and sodium tert-butoxide (10.6 mg, 0.110 mmol) were dissolved in THF (2.50 mL). The reaction mixture was stirred at room temperature overnight. The crude product was purified by flash column chromatography (n-hexane
:
ethyl acetate 3
:
1) and concentrated under reduced pressure to obtain a product 5a as a green powder with 76% yield. Single crystals suitable for crystallography were obtained by liquid diffusion of n-hexane into a saturated ethyl acetate solution at room temperature. 1H NMR (400 MHz, CDCl3): δ 7.43 (d, J = 9.2 Hz, 1H), 7.25 (s, 1H), 7.04 (m, 3H), 6.92 (s, 2H), 6.53 (d, J = 6.6 Hz, 1H), 2.40 (s, 3H), 2.30 (s, 3H), 2.08 (s, 6H), 1.94 (s, 6H) ppm; 13C NMR (100 MHz, CDCl3): δ 141.0, 139.5, 139.0, 136.3, 136.2, 134.0, 131.5, 130.2, 129.6, 129.4, 123.5, 116.5, 115.1, 112.6, 21.5, 21.2, 19.73, 17.7 ppm (signal from the carbon bonded metal was not detected); IR (ATR): ν = 3136, 2953, 2915, 2855, 1651, 1491, 1441, 1359, 1315, 1196, 1155, 1032, 851, 792, 784, 736, 682 cm−1; HR-MS (EI): calcd for C25H26N2CuCl [M] 452.1081 found 452.1081.
(2-Adamantyl-5-mesityl imidazo[1,5-a]pyridinyl) copper(I) chloride (5b). With the same method used in the synthesis of 5a, compound 5b was obtained as a white powder with 64% yield from 4b. 1H NMR (400 MHz, CDCl3): δ 7.50 (s, 1H), 7.34 (d, J = 9.2 Hz, 1H), 7.05 (s, 2H), 6.92 (dd, J = 9.2, 6.6 Hz, 1H), 6.42 (d, J = 6.5 Hz, 1H), 2.46 (s, 6H), 2.42 (s, 3H), 2.24 (s, 3H), 2.01 (s, 6H), 1.75 (m, 6H) ppm; 13C NMR (100 MHz, CDCl3): δ 140.9, 138.8, 136.3, 130.1, 129.9, 129.7, 122.5, 116.6, 114.8, 108.2, 59.6, 45.0, 35.8, 30.0, 21.6, 19.7 ppm (signal from the carbon bonded metal was not detected); IR (ATR): ν = 3181, 3045, 2911, 2850, 1650, 1452, 1304, 1156, 1081, 866, 780, 737, 705, 669 cm−1; HR-MS (EI): calcd for C26H30N2CuCl [M] 468.1394 found 468.1398.
(2-(2,6-Diisopropylphenyl)-5-mesityl imidazo[1,5-a]pyridinyl)copper(I) chloride (5c). With the same method used in the synthesis of 5a, compound 5c was obtained as a pale yellow powder with 96% yield from 4c. 1H NMR (300 MHz, CDCl3): δ 7.46 (m, 2H), 7.30 (s, 1H), 7.22 (d, J = 7.8 Hz, 2H), 7.08 (m, 3H), 6.56 (d, J = 6.6 Hz, 1H), 2.40 (s, 3H), 2.18 (m, 2H), 2.09 (s, 6H), 1.18 (d, J = 6.8 Hz, 6H), 1.12 (d, J = 6.8 Hz, 6H) ppm; 13C NMR (100 MHz, CDCl3): δ 145.1, 141.2, 139.0, 136.2, 135.5, 131.2, 130.6, 130.1, 129.7, 124.0, 123.8, 116.5, 115.3, 113.8, 28.5, 24.9, 24.2, 21.5, 19.6 ppm (signal from the carbon bonded metal was not detected); IR (ATR): ν = 3144, 2960, 2922, 2864, 1739, 1651, 1468, 1366, 1313, 1178, 846, 808, 797, 787, 767, 731, 684 cm−1; HR-MS (EI): calcd for C28H32N2CuCl [M] 494.1550 found 494.1552.
(5-Mesityl-2-(2-(2-(2-methoxyethoxy)ethoxy)phenyl)-2,3-dihydroimidazo[1,5-a]pyridin-3-yl)copper(I) chloride (5g). 5-Mesityl-2-(2-(2-(2-methoxyethoxy)ethoxy)phenyl)-2H-imidazo[1,5-a]pyridinium chloride 4g (149 mg, 0.320 mmol) and silver(II)oxide (74 mg, 0.320 mmol) were dissolved in CH2Cl2 (7.40 mL). The reaction mixture was stirred at room temperature overnight in the dark. The mixture was then filtered through a Celite pad and washed with CH2Cl2. The crude product was dissolved in CH2Cl2 (14 mL) and copper chloride(I) (63 mg, 0.640 mmol) was added in one portion. The reaction was stirred at room temperature overnight. The mixture was then filtered through a Celite pad and washed with CH2Cl2. The crude product was purified by flash column chromatography (n-hexane
:
ethyl acetate 1
:
6) and concentrated under reduced pressure to obtain a product 5g as an ivory powder with 79% yield. Single crystals suitable for crystallography were obtained by liquid diffusion of n-hexane into a saturated benzene or THF solution at room temperature. 1H NMR (400 MHz, CDCl3): δ 7.75 (s, 1H), 7.57 (d, J = 7.9 Hz, 1H), 7.41 (d, J = 9.3 Hz, 1H), 7.33 (t, J = 7.1 Hz, 1H), 7.04 (s, 2H), 6.98 (m, 3H), 6.46 (d, J = 6.5 Hz, 1H), 4.18 (t, J = 4.4 Hz, 2H), 3.74 (t, J = 4.8 Hz, 2H), 3.56 (m, 2H), 3.48 (m, 2H), 3.34 (s, 3H), 2.40 (s, 3H), 2.08 (s, 6H) ppm; 13C NMR (100 MHz, CDCl3): δ 168.6, 152.1, 140.8, 138.5, 136.3, 130.7, 130.4, 130.1, 129.5, 129.4, 128.1, 122.9, 121.3, 116.7, 115.0, 114.4, 113.6, 71.9, 70.6, 69.3, 68.3, 59.0, 21.5, 19.7 ppm; IR (ATR): ν = 3146, 2882, 2226, 1651, 1601, 1505, 1452, 1359, 1291, 1104, 911, 847, 788, 729 cm−1; HR-MS (FAB): calcd for C27H30N2CuClO3 [M] 528.1241 found 528.1221.
2-(2-Methoxyethoxy)ethyl 4-methylbenzenesulfonate (8). Diethylene glycol monomethyl ether (6.01 g, 50 mmol), p-toluene sulfonyl chloride (11.4 g, 60 mmol), and pyridine (8.09 mL, 100 mmol) were combined at 0 °C, then stirred for 6 h at the same temperature. The reaction mixture was extracted with Et2O and water, and washed with 1 N HCl. The organic phase was dried over anhydrous MgSO4, filtered, and concentrated in a vacuum. The crude product was purified by flash column chromatography (n-hexane
:
ethyl acetate 3
:
1) to give a product 8 (90%) as a colorless oil. 1H NMR (400 MHz, CDCl3): δ 7.79 (d, J = 8.2 Hz, 2H), 7.32 (d, J = 8.4 Hz, 2H), 4.15 (m, 2H), 3.67 (m, 2H), 3.56 (m, 2H), 3.46 (m, 2H), 3.33 (s, 3H), 2.43 (s, 3H) ppm; 13C NMR (100 MHz, CDCl3) 144.8, 132.8, 129.8, 127.9, 71.7, 70.6, 69.2, 68.6, 59.0, 21.6 ppm.
2-(2-(2-Methoxyethoxy)ethoxy)aniline (9). To a solution of 2-nitrophenol (139 mg, 1.00 mmol) and potassium carbonate (138 mg, 1.00 mmol) in DMF (3.50 mL) was added a solution of 2-(2-methoxyethoxy)ethyl 4-methylbenzenesulfonate 8 (274 mg, 1.00 mmol) in DMF (2.00 mL) and allowed to reflux overnight. Afterward, the mixture was cooled to room temperature. The solvent was evaporated and the residue was dissolved in ethyl acetate. The organic phase was washed with water, dried over anhydrous MgSO4, then filtered and concentrated in a vacuum. The crude mixture was subjected to hydrogenation over Pd/C in MeOH (8.00 mL) at room temperature under H2 atmosphere for 24 h. Pd/C was filtered through a Celite pad and the filtrate was concentrated under reduced pressure. The crude product was purified by flash column chromatography (n-hexane
:
ethyl acetate 2
:
1) to give a product 9 (84%, 2 steps) as a dark orange oil. 1H NMR (400 MHz, CDCl3): δ 6.82–6.78 (m, 2H), 6.73–6.67 (m, 2H), 4.16 (m, 2H), 3.86 (m, 2H), 3.71 (m, 2H), 3.57 (m, 2H), 3.39 (s, 3H) ppm; 13C NMR (100 MHz, CDCl3): δ 146.1, 136.8, 121.5, 118.0, 115.0, 112.5, 71.7, 70.4, 69.6, 68.0, 58.9 ppm.; IR (ATR): ν = 3458, 3358, 3058, 2924, 2876, 2822, 1616, 1505, 1458, 1277, 1218, 1105, 1053, 927, 848, 738 cm−1; HR-MS (EI): calcd for C11H17NO3 [M]+ 211.12 found 211.1209.
General procedure for catalytic direct C–H carboxylation with CO2
General procedures for ImPy Cu-catalyst generated in situ for direct C–H carboxylation of heterocyclic compound. General procedures were adapted from those used by Hou et al.16c Ligand (5 mol%), copper chloride(I) (2.50 mg, 5 mol%), and potassium tert-butoxide (65.1 mg, 0.580 mmol) were added to a 30 mL Schlenk flask equipped with Teflon-valve in a glove box. THF (2.50 mL) was added to the reaction flask and stirred at room temperature for 4 hours. Under an Ar atmosphere, a heterocyclic compound (59.6 mg, 0.500 mmol) was added to the reaction mixture. The mixture was degassed through three freeze–pump–thaw cycles and CO2 (1 atm) was charged into the reaction flask. The sealed Schlenk flask was stirred at 80 °C for 14 hours. After the reaction mixture was cooled to room temperature, the solvent was removed under reduced pressure. DMF (2.50 mL) and 1-iodohexane (0.150 mL, 1.00 mmol) were added to the mixture under an Ar atmosphere and stirred at 80 °C for 6 hours. The reaction mixture was dissolved in ethyl acetate and washed with water. The organic layer was dried over anhydrous Na2SO4, filtered, and concentrated in a vacuum. The crude product was purified by flash column chromatography (n-hexane
:
ethyl acetate 8
:
1) to give the product.
General procedures for ImPy Cu-catalyzed carboxylation of benzoxazole with CO2. ImPy–Cu complexes (5 mol%) and potassium tert-butoxide (61.7 mg, 0.550 mmol) were added to a 30 mL Schlenk flask equipped with Teflon-valve in a glove box and THF (2.50 mL) was charged into the reaction flask. Benzoxazole (59.6 mg, 0.500 mmol) was added and the mixture was degassed through three freeze–pump–thaw cycles. CO2 (1 atm) was charged into the reaction flask. The reaction mixture was stirred at 80 °C for 14 hours. After the reaction mixture was cooled to room temperature, the solvent was removed under reduced pressure. DMF (2.50 mL) and 1-iodohexane (0.150 mL, 1.00 mmol) were added to the mixture under an Ar atmosphere and stirred at 80 °C for 6 hours. Subsequent procedures remained the same as described above.All compounds were reported materials16c except for 5-methoxy-benzoxazole-2-carboxylic acid hexyl ester (7e).
5-Methoxy-benzoxazole-2-carboxylic acid hexyl ester (7e). Yellow oil; 1H NMR (400 MHz, CDCl3): δ 7.49 (dd, J = 9.0, 2.3 Hz, 1H), 7.25 (t, J = 2.4 Hz, 1H), 7.08 (dt, J = 9.1, 2.5 Hz, 1H), 4.43 (td, J = 6.8, 2.0 Hz, 2H), 3.82 (d, J = 2.3 Hz, 3H), 1.85–1.77 (m, 2H), 1.43–1.28 (m, 6H), 0.85 (m, 3H) ppm; 13C NMR (75 MHz, CDCl3): δ 158.2, 156.6, 153.4, 145.6, 141.5, 118.0, 112.0, 103.5, 67.3, 56.0, 31.4, 28.5, 25.5, 22.5, 14.0 ppm; IR (ATR): ν = 2932, 2856, 1732, 1609, 1544, 1484, 1438, 1325, 1260, 1205, 1152, 1025, 853, 815 cm−1; HR-MS (EI): calcd for C15H19NO4 [M] 277.1314 found 277.1312.
Conflicts of interest
There are no conflicts to declare.
Acknowledgements
This work is supported by the Korea CCS R&D Center (Korea CCS 2020 Project) grant funded by the Korea government (Ministry of Science, ICT & Future Planning) in 2016 (NRF-2014M1A8A1049301), and by the “GIST-Caltech Research Collaboration” grant funded by the GIST in 2017.
Notes and references
- For recent reviews see:
(a) S. Díez-González, N. Marion and S. P. Nolan, Chem. Rev., 2009, 109, 3612 CrossRef PubMed;
(b) T. Dröge and F. Glorius, Angew. Chem., Int. Ed., 2010, 49, 6940 CrossRef PubMed;
(c) S. Gaillard, C. S. J. Cazin and S. P. Nolan, Acc. Chem. Res., 2012, 45, 778 CrossRef CAS PubMed;
(d) C. Valente, S. Çalimsiz, K. H. Hoi, D. Mallik, M. Sayah and M. G. Organ, Angew. Chem., Int. Ed., 2012, 51, 3314 CrossRef CAS PubMed;
(e) D. J. Nelson and S. P. Nolan, Chem. Soc. Rev., 2013, 42, 6723 RSC;
(f) M. N. Hopkinson, C. Richter, M. Schedler and F. Glorius, Nature, 2014, 510, 485 CrossRef CAS PubMed.
- C. Burstein, C. W. Lehmann and F. Glorius, Tetrahedron, 2005, 61, 6207 CrossRef CAS.
- M. Alcarazo, S. J. Roseblade, A. R. Cowley, R. Fernández, J. M. Brown and J. M. Lassaletta, J. Am. Chem. Soc., 2005, 127, 3290 CrossRef CAS PubMed.
- A. Fürstner, M. Alcarazo, H. Krause and C. W. Lehmann, J. Am. Chem. Soc., 2007, 129, 12676 CrossRef PubMed.
- M. Nonnenmacher, D. Kunz, F. Rominger and T. Oeser, J. Organomet. Chem., 2007, 692, 2554 CrossRef CAS.
-
(a) S. J. Roseblade, A. Ros, D. Monge, M. Alcarazo, E. Álvarez, J. M. Lassaletta and R. Fernández, Organometallics, 2007, 26, 2570 CrossRef CAS;
(b) C. Grohmann, T. Hashimoto, R. Fröhlich, Y. Ohki, K. Tatsumi and F. Glorius, Organometallics, 2012, 31, 8047 CrossRef CAS.
- J. T. Hutt and Z. D. Aron, Org. Lett., 2011, 13, 5256 CrossRef CAS PubMed.
-
(a) J.-L. Zhang, L.-A. Chen, R.-B. Xu, C.-F. Wang, Y.-P. Ruan, A.-E. Wang and P.-Q. Huang, Tetrahedron: Asymmetry, 2013, 24, 492 CrossRef CAS;
(b) A. Schmidt, N. Grover, T. K. Zimmermann, L. Graser, M. Cokoja, A. Pöthig and F. E. Kühn, J. Catal., 2014, 319, 119 CrossRef CAS;
(c) A. Tronnier, D. Schleicher and T. Strassner, J. Organomet. Chem., 2015, 775, 155 CrossRef CAS;
(d) F. Grande-Carmona, J. Iglesias-Sigüenza, E. Álvarez, E. Díez, R. Fernández and J. M. Lassaletta, Organometallics, 2015, 34, 5073 CrossRef CAS;
(e) C. T. Check, K. P. Jang, C. B. Schwamb, A. S. Wong, M. H. Wang and K. A. Scheidt, Angew. Chem., Int. Ed., 2015, 54, 4264 CrossRef CAS PubMed;
(f) M. Espina, I. Rivilla, A. Conde, M. M. Díaz-Requejo, P. J. Pérez, E. Álvarez, R. Fernández and J. M. Lassaletta, Organometallics, 2015, 34, 1328 CrossRef CAS;
(g) F. Grande-Carmona, J. Iglesias-Sigüenza, E. Álvarez, E. Díez, R. Fernández and J. M. Lassaletta, Organometallics, 2015, 34, 5073 CrossRef CAS;
(h) R. Nakano and K. Nozaki, J. Am. Chem. Soc., 2015, 137, 10934 CrossRef CAS PubMed;
(i) W.-j. Tao, R. Nakano, S. Ito and K. Nozaki, Angew. Chem., Int. Ed., 2016, 55, 2835 CrossRef CAS PubMed;
(j) Y. Kim, Y. Kim, M. Y. Hur and E. Lee, J. Organomet. Chem., 2016, 820, 1 CrossRef CAS;
(k) J. Iglesias-Sigüenza, C. Izquierdo, E. Díez, R. Fernández and J. M. Lassaletta, Dalton Trans., 2016, 45, 10113 RSC;
(l) Y. Koto, F. Shibahara and T. Murai, Org. Biomol. Chem., 2017, 15, 1810 RSC;
(m) W. Tao, S. Akita, R. Nakano, S. Ito, Y. Hoshimoto, S. Ogoshib and K. Nozaki, Chem. Commun., 2017, 53, 2630 RSC.
-
(a) E. Peris, Chem. Rev., DOI:10.1021/acs.chemrev.6b00695;
(b) D. J. Nelson, A. Collado, S. Manzini, S. Meiries, A. M. Z. Slawin, D. B. Cordes and S. P. Nolan, Organometallics, 2014, 33, 2048 CrossRef CAS;
(c) B. Ramasamy and P. Ghosh, Eur. J. Inorg. Chem., 2016, 1448 CrossRef CAS.
-
(a) X. Li, M. Hou, Z. Zhang, B. Han, G. Yang, X. Wang and L. Zou, Green Chem., 2008, 10, 879 RSC;
(b) Z.-Z. Yang, Y.-N. Zhao, L.-N. He, J. Gao and Z.-S. Yin, Green Chem., 2012, 14, 519 RSC;
(c) Z. Z. Yang, Q. W. Song and L. N. He, Capture and Utilization of Carbon Dioxide with Polyethylene Glycol, Springer, 2012 Search PubMed.
-
(a) R. Luo, X. Zhou, S. Chen, Y. Li, L. Zhou and H. Ji, Green Chem., 2014, 16, 1496 RSC;
(b) K.-i. Fujita, J. Sato, K. Inoue, T. Tsuchimoto and H. Yasuda, Tetrahedron Lett., 2014, 55, 3013 CrossRef CAS;
(c) R. Luo, W. Zhang, Z. Yang, X. Zhou and H. Ji, J. CO2 Util., 2017, 19, 257 CrossRef CAS.
-
(a) D. W. Cai, D. L. Hughes and T. R. Verhoeven, Tetrahedron Lett., 1996, 37, 2537 CrossRef CAS;
(b) R. G. Hicks, B. D. Koivisto and M. T. Lemaire, Org. Lett., 2004, 6, 1887 CrossRef CAS PubMed.
- C.-Y. Wang, Y.-H. Liu, S.-M. Peng and S.-T. Liu, J. Organomet. Chem., 2006, 691, 4012 CrossRef CAS.
- Selected reviews:
(a) T. Sakakura, J.-C. Choi and H. Yasuda, Chem. Rev., 2007, 107, 2365 CrossRef CAS PubMed;
(b) K. Huang, C.-L. Sun and Z.-J. Shi, Chem. Soc. Rev., 2011, 40, 2435 RSC;
(c) M. Cokoja, C. Bruckmeier, B. Rieger, W. A. Herrmann and F. E. Kühn, Angew. Chem., Int. Ed., 2011, 50, 8510 CrossRef CAS PubMed;
(d) R. Martín and A. W. Kleij, ChemSusChem, 2011, 4, 1259 CrossRef PubMed;
(e) Y. Tsuji and T. Fujihara, Chem. Commun., 2012, 48, 9956 RSC;
(f) C. Maeda, Y. Miyazaki and T. Ema, Catal. Sci. Technol., 2014, 4, 1482 RSC;
(g) M. Aresta, A. Dibenedetto and A. Angelini, Chem. Rev., 2014, 114, 1709 CrossRef CAS PubMed;
(h) D. Yu, S. P. Teong and Y. Zhang, Coord. Chem. Rev., 2015, 293–294, 279 CrossRef CAS, Börjesson, M.; Moragas, T.; Gallego, D.;
(i) Q. Liu, L. Wu, R. Jackstell and M. Beller, Nat. Commun., 2015, 6, 5933 CrossRef PubMed;
(j) M. Börjesson, T. Moragas, D. Gallego and R. Martin, ACS Catal., 2016, 6, 6739 CrossRef PubMed.
- Selected examples of C(sp)–H carboxylation:
(a) W.-Z. Zhang, W.-J. Li, X. Zhang, H. Zhou and X.-B. Lu, Org. Lett., 2010, 12, 4748 CrossRef CAS PubMed;
(b) F. Manjolinho, M. Arndt, K. Gooßen and L. J. Gooßen, ACS Catal., 2012, 2, 2014 CrossRef CAS;
(c) S. Li, J. Sun, Z. Zhang, R. Xie, X. Fang and M. Zhou, Catal. Sci. Technol., 2013, 3, 912 RSC.
- Selected examples of C(sp2)–H carboxylation:
(a) I. I. F. Boogaerts and S. P. Nolan, J. Am. Chem. Soc., 2010, 132, 8858 CrossRef CAS PubMed;
(b) I. I. F. Boogaerts, G. C. Fortman, M. R. L. Furst, C. S. J. Cazin and S. P. Nolan, Angew. Chem., Int. Ed., 2010, 49, 8674 CrossRef CAS PubMed;
(c) L. Zhang, J. Cheng, T. Ohishi and Z. Hou, Angew. Chem., Int. Ed., 2010, 49, 8670 CrossRef CAS PubMed;
(d) L. Ackermann, Angew. Chem., Int. Ed., 2011, 50, 3842 CrossRef CAS PubMed;
(e) I. I. F. Boogaerts and S. P. Nolan, Chem. Commun., 2011, 47, 3021 RSC;
(f) H. Inomata, K. Ogata, S.-i. Fukuzawa and Z. Hou, Org. Lett., 2012, 14, 3986 CrossRef CAS PubMed.
- Selected examples of directed C–H carboxylation:
(a) H. Mizuno, J. Takaya and N. Iwasawa, J. Am. Chem. Soc., 2011, 133, 1251 CrossRef CAS PubMed;
(b) K. Sasano, J. Takaya and N. Iwasawa, J. Am. Chem. Soc., 2013, 135, 10954 CrossRef CAS PubMed; selected examples of C–H carboxylation with simple arene:
(c) T. Suga, H. Mizuno, J. Takaya and N. Iwasawa, Chem. Commun., 2014, 50, 14360 RSC.
-
(a) K. Michigami, T. Mita and Y. Sato, J. Am. Chem. Soc., 2017, 139, 6094 CrossRef CAS PubMed;
(b) Y. Masuda, N. Ishida and M. Murakami, J. Am. Chem. Soc., 2015, 137, 14063 CrossRef CAS PubMed;
(c) N. Ishida, Y. Masuda, S. Uemoto and M. Murakami, Chem.–Eur. J., 2016, 22, 6524 CrossRef CAS PubMed;
(d) H. Seo, M. H. Katcher and T. F. Jamison, Nat. Chem., 2017, 9, 453 CrossRef CAS PubMed;
(e) Y.-Y. Gui, W.-J. Zhou, J.-H. Ye and D.-G. Yu, ChemSusChem, 2017, 10, 1337 CrossRef CAS PubMed.
- S. H. Cho, J. Y. Kim, Y. Lee and S. Chang, Angew. Chem., Int. Ed., 2009, 48, 9127 CrossRef CAS PubMed.
- T. Kawano, K. Hirano, T. Satoh and M. Miura, J. Am. Chem. Soc., 2010, 132, 6900 CrossRef CAS PubMed.
-
(a) M. Micksch, M. Tenne and T. Strassner, Eur. J. Org. Chem., 2013, 6137 CrossRef CAS;
(b) A. P. Blum, T. Ritter and R. H. Grubbs, Organometallics, 2007, 26, 2122 CrossRef CAS.
- X. Bantreil and S. P. Nolan, Nat. Protoc., 2011, 6, 69 CrossRef CAS PubMed.
- V. Jurkauskas, J. P. Sadighi and S. L. Buchwald, Org. Lett., 2003, 5, 2417 CrossRef CAS PubMed.
Footnote |
† Electronic supplementary information (ESI) available. CCDC 1576746 and 1576622. For ESI and crystallographic data in CIF or other electronic format see DOI: 10.1039/c7ra11414a |
|
This journal is © The Royal Society of Chemistry 2017 |
Click here to see how this site uses Cookies. View our privacy policy here.