DOI:
10.1039/C7RA10751J
(Paper)
RSC Adv., 2017,
7, 55088-55097
Characterizing the free ammonia exposure to the nutrients removal in activated sludge systems†
Received
28th September 2017
, Accepted 21st November 2017
First published on 5th December 2017
Abstract
As a by-product during liquid production, the liquor wastewater exhibits tremendous environmental risks and may cause undesirable effects to the biological systems due to the high concentration of ammonia. This study was carried out to characterize the effects of high concentration free ammonia (FA) exposure in liquor wastewater on the removal of nutrients (NH4+–N, NOx−–N, PO43− and organics) in sequencing batch reactors (SBRs). Experimental data demonstrated that the short-term exposure of FA had little effect on the removal of typical pollutants. In the long-term, however, it was found that the removal of NH4+–N was determined by the counteraction of negative effects caused by high concentration FA and the contribution brought by initial substrate concentration. Moreover, the high concentration FA had evident inhibitory effects on both the nitrification process and the phosphorus removal. The side-effect of high FA on organics removal was insignificant and the lower removal efficiency of apparent COD in high FA reactors was primarily attributed to the accumulation of NO2–N, which was commonly neglected in previous studies. Mechanism explorations indicated that the microbes in the SBRs could excrete additional protein-dominated extracellular polymeric substances (EPS) to resist the side-effects caused by high FA. This made the sludge structure more compact, while it blocked the transport of substrates in the process of pollutants removal. Furthermore, FA would also influence the microbial diversity and reduce the abundance of key microorganisms (i.e. ammonia-oxidizing bacteria, nitrite-oxidizing bacteria and phosphate-accumulating organism) responsible for nutrients removal; thus the corresponding removal efficiency subsequently decreased in the presence of high FA.
1. Introduction
As one of the most featured industry in China, the production of Chinese liquor is extremely high every year. Statistics show that the amount of liquor production was up to 13.6 million tons in 2016, which accounted for about 38% of the world's total production.1 Consequently, large amounts of liquor wastewater were generated. It has been evaluated that 1 ton liquor production would generate 15 tons of wastewater during the cleaning, fermentation and distillation processes.2 The wastewater, which contains high concentrations of organics and NH4+–N, was difficult to treat, which resulted in great environmental risks.
The common disposal of liquor wastewater includes electrodialysis, flocculation, ozonation, and biological treatment.2 In particular, the biological approach was the preferred way considering its environmental friendliness, effectiveness and low costs.3,4 However, the high concentration of NH4+–N, which could also be present in the form of un-ionized ammonia (free ammonia, NH3 (FA)), would cause a huge shock to the biological systems and influence their treatment ability and capacity.5,6 Chen has demonstrated that the ammonium oxidation ability was greatly inhibited and the removal efficiency of ammonia nitrogen decreased significantly at FA > 15 mg L−1.7 The subsequent nitrite oxidation could also be affected by the high concentration of FA as the process has been reported to be completely inhibited when FA exceeded 16 mg L−1.8 In addition, it was found that FA had severe inhibitory effects on the phosphorus uptake during aerobic process and ultimately resulted in a phosphate excess in effluents.9 Moreover, the removal of organics was also reported to be affected in the presence of FA. The chemical oxygen demand (COD) removal rate and the concentration of dehydrogenase, which were directly related with the removal rate of organics, were both observed to gradually decrease with the increase in FA.10,11 However, most of the previous studies primarily focused on the effects of FA, on the removal of individual pollutant and its specific removal efficiency.9–11 In general, pollutants co-exist in wastewater and the degradation of one particular pollutant would exhibit competitive or synergistic effects with other pollutants and ultimately influence their respective removal efficiency. Thus, it was very important and urgent to investigate the influence of FA exposure to the removal of nutrients and their internal connection in biological systems. Moreover, though previous studies have disclosed that FA had adverse effects on the microbial activity as well as the stability of sludge, which led to the inhibition of pollutants removal,12 the information on detailed mechanisms for changes in sludge characteristics and shifts of microbial community caused by high concentration FA was limited.
In this study, the effects of FA on the simultaneous removal of typical pollutants including NH4+–N, NOx−–N, PO43− and organics were first investigated in the sequencing batch reactors (SBRs). Then, the particular influenced stages for every specific pollutant by FA and their mutual interactions in the removal process were explored. Furthermore, variations of the structure and characteristics of activated sludge in SBRs with different concentrations of FA and the related mechanisms are discussed in detail. In addition, the shifts of microbial communities responsible for the removal of pollutants in SBRs were studied using high-throughput pyrosequencing.
2. Materials and methods
2.1. Inoculated sludge and inlet wastewater
The inoculated sludge was taken from the secondary sedimentation tank of wastewater treatment plant in Nanjing, China. Prior to the experiment, the sludge was rinsed with distilled water for 9 h to remove the effects of the original substrate on the test. The initial suspended solids concentration (MLSS) in SBRs was 4000 mg L−1. According to the survey, the concentration of NH4+–N in the liquor wastewater ranges from 80 mg L−1 to 400 mg L−1 in different seasons (Table S1†); thus the influent concentrations of NH4+–N in four reactors (R1–R4) were set as 50, 100, 200 and 350 mg L−1, respectively.
2.2. Experimental set-up and procedure
The experimental work was performed in four identical lab-scale SBRs with an effective volume of 2.5 L. The SBRs were operated by alternating anoxic and aerobic reactions with a cycle time of 24 h and consisted of five phases: 4 h for stirring under anoxic conditions, 16 h for aeration, 1 h for precipitation, 10 min for drainage and the remaining time for idling. The batch tests (on cycle) were initially implemented in SBRs to investigate the short-term effects of FA on the performance of SBRs. Then, the chronic toxic effects of FA were also studied with long-term exposure. The hydraulic retention time and the sludge age were about 48 h and 10 cycles, respectively. To create an anoxic environment, argon was stirred into the influent for 20 min before entering the water. During aeration stage, the dissolved oxygen concentration was controlled at about 3 mg L−1 by the air blower and the gas flowmeter. The room temperature was maintained at 27 ± 1 °C.
2.3. Analytical methods
The concentrations of NO3−–N, NO2−–N, NH4+–, COD, PO43−, MLSS, and MLVSS were assayed according to Chinese SEPA Standard Methods (SEPA, 2002). The pH and DO values were determined with pH meter (HACH-sension2) and portable dissolved oxygen meter (HACH-HQ30d), respectively. The surface structure of sludge was measured by scanning electron microscope (JEOLJEM-1400, JAPAN).
According to Anthonisen et al.,5 the concentration of free ammonia (FA, which was related to NH4+–N concentration, pH and temperature (T)), was calculated by the following equation (eqn (1)):
|
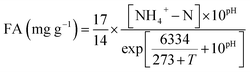 | (1) |
According to the eqn (1), the FA concentrations of R1, R2, R3 and R4 were as follows: 2.9, 5.6, 11.1, and 19.5 mg L−1 (pH 7.85).
The nitrite accumulation ratio (NAR) of the reactor was calculated according to the following equation (eqn (2)):
|
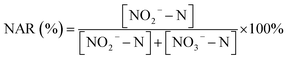 | (2) |
2.4. EPS analysis
The method to extract EPS was based on cation exchange using a cation exchange resin (CER) reported by Dubois et al.13 The anthrone-sulfuric acid method was used to measure the polysaccharides (PS) concentration,14 and the modified Lowry method was used to measure proteins (PN) concentration.15 The detailed substances in EPS were explored by 3D fluorescence luminescence spectrometer (Hitachi, F7000) at cycle 1 and cycle 27. The excitation wavelength (Ex) and emission wavelength (Em) of EEM spectra were from 200 to 700 nm with the increment of 10 and 2 nm, respectively. The excitation and emission slit were 5 nm and the scanning speed was 12
000 nm min−1. The data of EEM spectra was analyzed by Origin 8.5.
2.5. High-throughput sequencing
For a complete understanding of the microbial community structure of sludge with different FA concentrations, high-throughput sequencing technique was explored when the SBRs were stabilized. The samples were prepared according to the OMEGA Reagent Kit Preparation Guide (E.Z.N.ATM Mag-Bind Soil DNA Kit) and tested by Omega Bio-Tek Company (Agent: Guangzhou flying Biological Engineering Co, Ltd, Nanjing, China). The primers used in high-throughput sequencing analysis were 341F (5′-CCTAYGGGRBGCASCAG-3′) and 806R (5′-GGACTACNNGGGTATCTAAT-3′).16
2.6. Statistical analysis
All tests in the present study were carried out in triplicate. An analysis of variance (ANOVA, p < 0.05) was used to test the significance of results.
3. Results and discussion
3.1. Effects of FA on the SBR operation
The short-term effects of FA on the performance of SBRs were first investigated. The obtained results showed that no evident differences on typical pollutants (NH4+–N, NO3−–N, PO43− and organics) removal were observed among reactors with different FA concentration (data not shown), which excluded the acute effects of FA on the activated sludge system. However, the SBRs are commonly operated continuously in real application and thus the study on the long-term effects of FA was more concerning and attractive. As shown in Fig. 1(a), the removal of NH4+–N appeared to be clearly decreased with the increase in FA concentration as the removal efficiencies were 99.5%, 99.0%, 68.2% and 38.8% in R1–R4, respectively. However, by calculating the total removal amounts, it can be observed that the reduction of NH4+–N was 49.8, 99.0, 136.4 and 135.8 mg L−1. Unexpectedly, the total reduction of NH4+–N was even more in R3 and R4. Previous studies indicated that high concentration of FA would inhibit the activities of ammonia-oxidizing bacteria (AOB) and thus decrease the NH4+–N removal.8 However, it was also reported that the initial high concentration of NH4+–N was closely related with its removal rate.17 In the present study, the contribution of initial high concentration of NH4+–N to its removal rate can outcompete the inhibitory effects of FA that resulted in the high removal amounts of NH4+–N. Moreover, it should be noted that the inhibitory effects of FA could be partly relieved after cultivation and adaptation as the removal efficiency of NH4+–N in the R3 were improved evidently after 9 cycles, which was consistent with previous literature.18
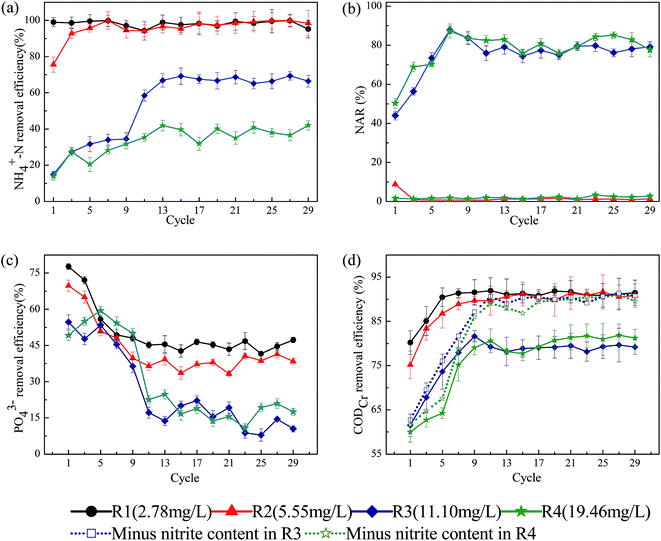 |
| Fig. 1 Long-term effects of FA on the typical pollutants removal: (a) NH4+–N; (b) NAR; (c) PO43−; (d) COD. | |
As to the nitrification process, high concentrations of NO2−–N were accumulated in R3 and R4 reactors. The NAR was about 76% and 83%, for R3 and R4 respectively (as shown in Fig. 1(b)) and the NO3−–N concentrations were relatively low. This demonstrated that the nitrite conversion process was severely inhibited in the R3 and R4 reactor. In case of the effects of FA on phosphorus removal, it can be observed from Fig. 1(c) that the phosphorus removal rate was also negatively affected by the FA. The removal efficiency was about 45% in R1 and 15% in R3 after operation of 10 cycles. The reason could be attributed to the toxicities of FA to the activities of polyphosphate accumulating organisms (PAO). It has been postulated that FA could inhibit the metabolic process of PAO and thus led to the decrease of phosphorus uptake.19
The metabolic activity of heterotrophic bacteria has also been reported to be affected by the high FA since the biodegradation rate of organic matters was inhibited.20 In the present study, the removal of organics in SBRs also appeared to be greatly affected by FA as the COD removal efficiency varied in different reactors and it was relatively low in R3 and R4 (as shown in Fig. 1(d)). However, further exploration has found that the lower COD removal efficiency was primarily attributed to the accumulation of NO2−–N. As a reducing substance, 1 mg NO2−–N is calculated to be equivalent to 1.14 mg COD. After excluding the contribution of NO2−–N to COD, the effects of FA on the COD removal in SBRs were actually insignificant (Fig. 1(d)). The result obtained is quite different from previous studies, which have neglected the contribution of NO2−–N to COD and assumed that the degradation of organic matter by heterotrophic bacteria was inhibited by FA.20
3.2. Mechanisms of the negative effects on pollutants removal by FA
It has been discussed above that FA affected the removal of N and P in SBR systems in the long-term run. However, the operation of SBRs included anoxic and aerobic processes. In order to deeply disclose the detailed effects of FA on the long-term performance of SBRs, FA impacts on the particular process in each cycle during SBR operation were further explored.
As shown in Fig. 2, the denitrification rate was very rapid during anoxic period as the concentration of NO2−–N and NO3−–N in the four reactors all dropped to about 0 within 1 h. These results indicated that the FA had minor inhibitory effects on the denitrifying bacteria under anoxic condition.
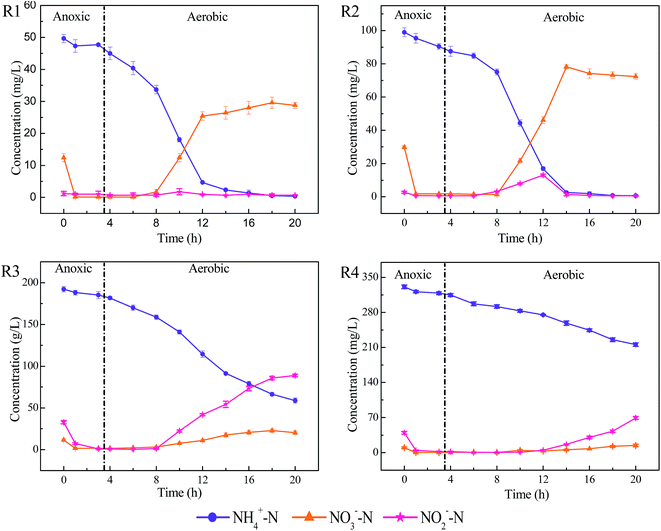 |
| Fig. 2 Dynamics of NH4+–N, NO2−–N and NO3−–N concentration in a cycle. | |
In case of the aerobic stage, the average NH4+–N removal rates in R1–R4 were 3.1, 6.2, 8.8 and 8.4 mg (L−1 h−1), respectively, indicating that initial high NH4+–N concentration offsets the inhibition on AOB by high FA. The generated NO2−–N was further converted to NO3−–N quickly in R1 and R2 as only minor quantity of NO2−–N was observed, while high concentration of NO3−–N was accumulated. However, contrary to R1 and R2, the concentration of NO3−–N was relatively low in R3, while NO2−–N gradually accumulated in the entire stage. Moreover, a significant lag occurred in R4 as no evident accumulation of NO2−–N was observed until 12 h; moreover, the concentration of NO3−–N was also very low. The results demonstrated that high FA affected the operation of SBRs by exhibiting negative effects on the oxidation process of NO2–N, which resulted in the accumulation of NO2−–N in aerobic stage. The reason could be attributed to the increasing biotoxicity of high FA to nitrite-oxidizing bacteria (NOB) and thus exhibited inhibitory effects on the nitrification process, which has also been reported by previous studies.21
In case of the organics removal, it can be observed from Fig. 3(a) that the removal rates of COD during anoxic stage in R3 and R4 were higher than that of R1 and R2, which could be attributed to the utilization of carbon substrates during denitrification process. In aerobic stage, the concentrations of COD kept decreasing initially because of the consumption of organics by heterotrophic bacteria in all reactors. However, the apparent COD started to increase later in R3 (10 h) and R4 (14 h). This exactly coincided with the time of significant NO2−–N accumulation. After subtracting the contribution of NO2−–N, the concentrations of COD showed little difference in all reactors. Thus, it was further confirmed that the increase of apparent COD concentration was primarily caused by the accumulation of NO2−–N and the high concentration FA had a minor effect on the organics removal.
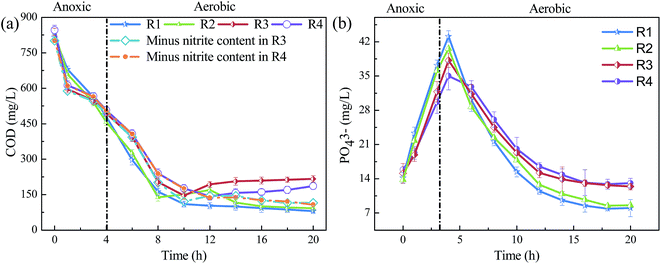 |
| Fig. 3 Dynamics of COD (a) and PO43− (b) concentration in one cycle. | |
The release of phosphate was also first affected by the FA in the anoxic stage. It can be observed from Fig. 3(b) that the concentration of PO43− in R1–R4 reached the maximum of 43.1, 40.6, 38.2 and 35.0 mg L−1 at the end of anoxic stage, respectively. These results illustrated that an evident inhibition of phosphate release occurred in the presence of high concentration FA. However, when it reached the aerobic stage, the phosphorus uptake was clearly faster in R1 and R2 compared with that in R3 and R4. From 4 to 10 h, the removal of phosphorus in the R1–R4 were 27.7, 22.8, 18.9 and 14.9 mg L−1, respectively. Some studies have reported that NO2−–N would inhibit the respiration of PAO under aerobic condition and thus reduced the removal efficiency of phosphate.9 However, in the present study, the NO2−–N accumulation did not appear during this period (Fig. 2). This indicated that the accumulation of NO2−–N was not the main reason for the decline of phosphate removal in R3 and R4, while the high concentration of FA would be the key inhibitory factor to the activity of phosphate accumulating microorganisms.
3.3. Microstructure properties of sludge characteristics
In order to further explore the mechanisms for the inhibitory effects of FA on typical nutrients removal in SBRs, the changes in sludge morphology and structure were first observed using a scanning electron microscope after stable operation. The results showed that the amount of mucus in the sludge flocs increased along with the increase in FA concentration. It can be observed from Fig. 4 that as compared with the loose structure in R1 and R2, the sludge in R3 and R4 was more compact and the floc was thicker. This indicated that large amounts of mucoid substances formed in R3 and R4, where most of the micro-organisms were embedded.
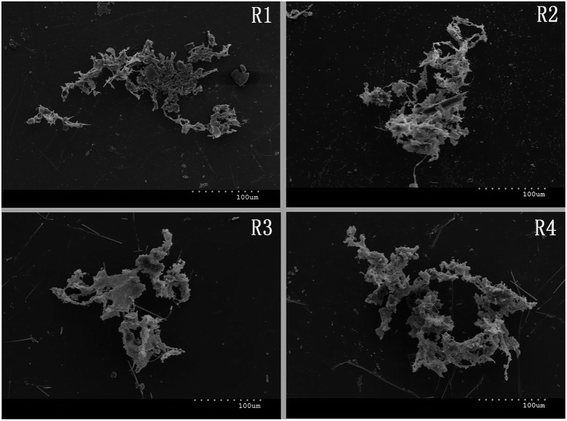 |
| Fig. 4 Scanning electron micrographs of sludge in different reactors in the stable stage. | |
The changes in sludge surface structure at high FA concentration resulted in massive losses of the original gully and channel as well as the reduction of mass exchange surface, which would block the exchange and transport of external nutrients and internal metabolites in cells. Therefore, the activities of functional microbes were negatively affected and the removal of typical pollutants was inhibited.
3.4. Effects of FA on extracellular polymeric substances (EPS) variations
Furthermore, the mucoid substances were expected to primarily consist of EPS as the microbial cells were reported to be able to produce extra EPS to protect them from adverse conditions,22,23 such as the toxicity caused by FA in this study. In order to testify this assumption, the overall amounts and components of EPS in SBRs with different FA concentrations were measured. As shown in Fig. 5 and Fig. 6, the main components of EPS in all reactors were PN and PS, while humic acid-like substance was quite low. Moreover, the amount of EPS was clearly more at high FA concentration, particularly in the R3 reactors, in which the EPS increased from 7.2 mg g−1 VSS in cycle 1 to 24.32 mg g−1 VSS in cycle 15.
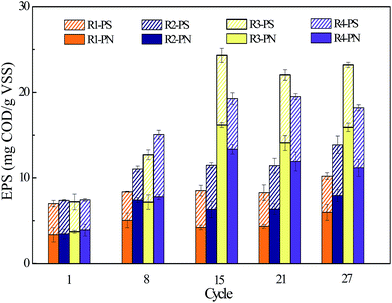 |
| Fig. 5 Changes in EPS contents during the operation. | |
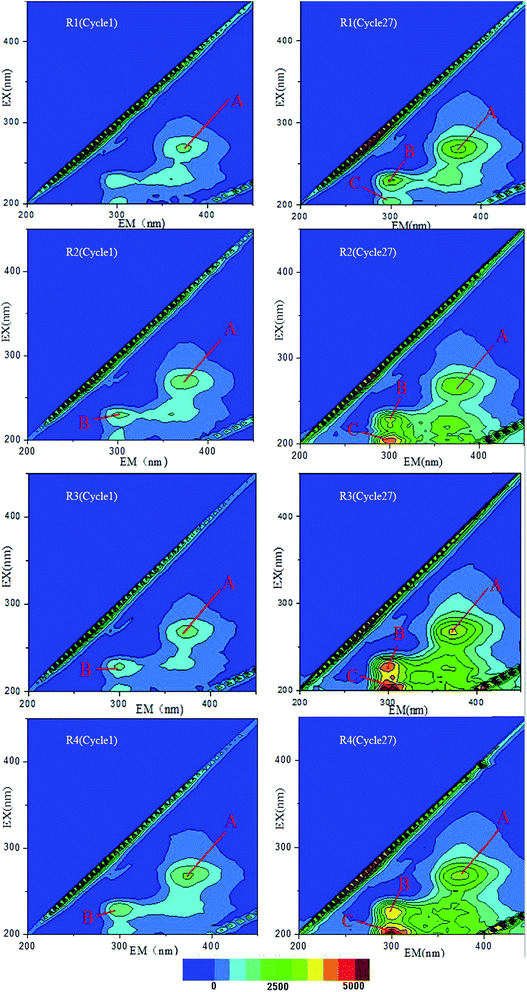 |
| Fig. 6 Fluorescent component of EPS samples in cycle 1 and 27 (peak A: tryptophan PN-like; peak B: tyrosine PN-like; peak C: aromatic PN-like). | |
In addition, it has been found that the increase in EPS was primarily derived from PN (as shown in Fig. 5) as the ratio of PN/PS in R1–R4 were 1.16, 1.27, 1.98 and 1.76, respectively after 15 d. It appeared that the microorganisms resisted the adverse environment by excreting more protein, which was consistent with previous studies.24 More precisely, it also can be observed from Fig. 6 and Table S2† that aromatic PN-like substances (peak C in Fig. 6) newly emerged after long-term operation in different reactors. Simultaneously, the high concentrations of FA would influence microorganisms secreting more tryptophan PN-like (peak A in Fig. 6) and tyrosine PN-like (peak B in Fig. 6) substances, which are consistent with the observations shown in Fig. 5. However, the polysaccharides have been reported to play more important roles in cohesion and adhesion of cells and the granules with lower PN/PS have been considered to have better function.25 For example, Liu et al. found that the decrease of polysaccharides could negatively influence the attachment and self-immobilization processes of bacteria in activated sludge.26 From this perspective, the function of activated sludge systems appeared to be altered due to high FA exposure.
Moreover, it is worthy to point out that the largest amount of produced EPS was observed in R3 during stable stage though the concentration of FA was lower than that in R4. This result is consistent with that shown in Fig. 4, in which the sludge was more compact in R3 compared with R4. Thus, it can be concluded that the tolerance of microorganisms under unfavorable conditions by excreting EPS was limited and they would gradually lose such protective mechanism when the concentration of toxic chemicals is beyond the tolerant value.27
3.5. Shifts of microbial community structure in the SBRs with FA
Microorganisms play key roles in the removal and biodegradation of typical nutrients in SBRs. Thus, in the current study, high-throughput sequencing was explored to analyze the structure of microbial community and the sample library coverage was more than 90% in all reactors, suggesting that it could well reflect the microbial community. The results showed that a total of 39 phyla were detected in SBRs reactors with 16 phyla jointly owned, but the microbial diversity and abundance clearly decreased owing to the existence of FA (as shown in Fig. 7(a) and Table S3†). For example, the abundances of Bacteroidetes, Verrucomicrobia and Acidobacteria, which played key roles during the nitrification process,28–30 all decreased in the presence of high concentration FA. Their abundance was determined to be 31.5%, 6.8%, and 1.87% in R1, while only 26.7%, 1.83%, and 0.4% was observed in R3, indicating that the high concentration of FA reduced the nitrification process by inhibiting the growth of related bacteria. However, the abundance of Proteobacteria, which has been reported to include large amounts of denitrifying bacteria with the ability of utilizing nitrate,31,32 increased significantly from 52.0% in R1 to 63.9% in R3. One of the possible explanations could be that a small amount of nitrate was accumulated in R3 as its denitrification process is not possibly affected by FA from the perspective of microbial analysis.
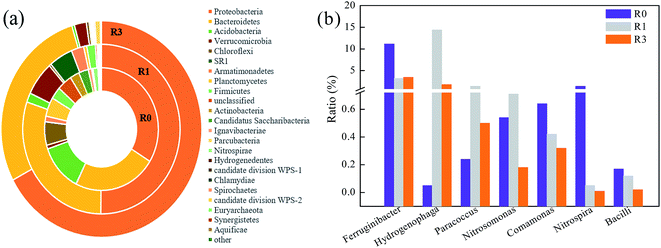 |
| Fig. 7 Abundances of the main microorganisms in SBRs under different conditions (R0 is the raw sludge): (a) phylum level; (b) genus level. | |
The function evolution of microbial community can be demonstrated better based on the genus level. Thus, in the current study, the relative abundance of key functional phylogenetic groups in SBRs at the genus level was also investigated (Fig. 7(b)). Among these genera, Ferruginibacter, which is known for its ability of degrading organic substance,33 showed little difference between R1 and R3 as the abundances were respectively 3.2% and 3.5%. This was consistent with the result of COD removal, which was not affected under different concentrations of FA in SBRs. However, the proportions of Hydrogenophaga, Paracoccus, Nitrosomonas, Comamonas, and Nitrospira, which were all regarded as heterotrophic nitrifiers responsible for the nitrification,34–39 decreased in R3 when compared with R1. In particular, the abundance of Nitrospira, as genera of NOB, was reduced by 80% in R3. Thus, the decrease of nitrifiers in the presence of high concentration FA was the main reason accounting for the reduction of nitrification removal rate and ultimately resulted in the accumulation of NO2−–N. Moreover, the abundance of Bacilli, which was proved to be a PAO,40 shrank by about 83% from R1 to R3. Thus, the phosphorus removal was then negatively affected in the high FA systems.
Overall, the shifts of microbial community suggested that the amounts and diversities of microorganisms were obviously affected by different FA concentration, which resulted in the variations of removal efficiency of different pollutants in SBRs.
4. Conclusions
In conclusion, the influences of FA on the removal of typical nutrients in the wastewater varied. High concentration of FA would exhibit negative effects on the removal efficiency of NH4+–N, but it was also correlated with the initial concentration. The oxidation of NO2−–N during nitrification process and the removal of phosphate was clearly inhibited due to the high concentration of FA. However, the degradation of organics was not affected in the presence of high concentration of FA though the apparent concentration of COD varied. This was primarily attributed to the contribution of NO2−–N accumulation. Moreover, it was found that the microorganisms resisted the side-effects of FA by excreting extra protein-dominated EPS, but it can simultaneously inhibit the exchange and transport of substances onto/into the microbes. The diversity and abundance of key microorganisms responsible for the removal of nutrients (e.g. NOB and PAO) were observed to decrease in the high concentration FA reactors and therefore the removal efficiencies of pollutants were negatively affected.
Conflicts of interest
There are no conflicts to declare.
Acknowledgements
The work is financially supported by the “Fundamental Research Funds for the Central Universities, No. 2017B01514”, “Open Funds of Key Laboratory of Integrated Regulation and Resource Development on Shallow Lakes, Ministry of Education, No. 2013/B1602020402”, “National Science and Technology Major Project, No. 2014ZX07305-002”; “National Natural Science Foundation of China (No. 51708171)” and the “Priority Academic Program Development of Jiangsu Higher Education Institutions (PAPD), China”.
References
- National liquor production in 2016-China industry information research network(online), 2017, http://www.askci.com/news/dxf/20170208/14345889977.shtml.
- Z. Chao, Z. Cui and S. Yan, Research Progress in the Treatment Technology of Brewing Wastewater, Contemporary Chemical Industry, 2014, 43, 447–449 Search PubMed.
- M. Zhou, T. Wang and X. Zhao, Treatment of waste water from Chinese liquor production by the anaerobic bio-contact and MBR process, China Brewing, 2011, 4, 93–95 Search PubMed.
- Y. Jo, J. Kim, S. Hwang and C. Lee, Anaerobic treatment of rice winery wastewater in an upflow filter packed with steel slag under different hydraulic loading conditions, Bioresour. Technol., 2015, 193, 53–61 CrossRef CAS PubMed.
- A. C. Anthonisen, R. C. Loehr, T. B. S. Prakasam and E. G. Srinath, Inhibition of Nitrification by Ammonia and Nitrous Acid, J.–Water Pollut. Control Fed., 1976, 48, 835–852 CAS.
- W. Qian, Y. Peng, X. Li, Q. Zhang and B. Ma, The inhibitory effects of free ammonia on ammonia oxidizing bacteria and nitrite oxidizing bacteria under anaerobic condition, Bioresour. Technol., 2017, 243, 1247–1250 CrossRef CAS PubMed.
- W. Chen, Analysis of the influence of free ammonia on the accumulation of nitrite in the nitrite nitrification system, Acta Geochim., 2009, 28, 440–444 Search PubMed.
- V. M. Vadivelu, J. Keller and Z. Yuan, Effect of free ammonia on the respiration and growth processes of an enriched Nitrobacter culture, Water Res., 2007, 41, 826–834 CrossRef CAS PubMed.
- Y. Yoshida, Y. Kim, T. Saito and K. Tanaka, Development of the modified activated sludge model describing nitrite inhibition of aerobic phosphate uptake, Water Sci. Technol., 2009, 59, 621–630 CrossRef CAS PubMed.
- Q. W. Cheng-hui and D. Yuan-hong, Influence of high concentration ammonia nitrogen on property of activated sludge, Industrial water & Wastewater, 2012, 43, 13–16 Search PubMed.
- F. Wang, Y. Ding, L. Ge, H. Ren and L. Ding, Effect of high-strength ammonia nitrogen acclimation on sludge activity in sequencing batch reactor, J. Environ. Sci., 2010, 22, 1683–1688 CrossRef CAS.
- X. Zheng, P. Sun, J. Lou, J. Cai, Y. Song, S. Yu and X. Lu, Inhibition of free ammonia to the granule-based enhanced biological phosphorus removal system and the recoverability, Bioresour. Technol., 2013, 148, 343–351 CrossRef CAS PubMed.
- M. Dubois, K. A. Gilles, J. K. Hamilton, P. A. Rebers and F. Smith, Colorimetric Method for Determination of Sugars and Related Substances, Anal. Chem., 1955, 28, 350–356 CrossRef.
- F. Bo, R. Palmgren, K. Keiding and P. H. Nielsen, Extraction of extracellular polymers from activated sludge using a cation exchange resin, Water Res., 1996, 30, 1749–1758 CrossRef.
- O. H. Lowry, N. J. Rosebrough, A. L. Farr and R. J. Randall, Protein Measurement with the Folin Phenol Reagent, J. Biol. Chem., 1951, 193, 265–275 CAS.
- M. H. Hjelmsø, L. H. Hansen, J. Bælum, L. Feld, W. E. Holben and C. S. Jacobsen, High-Resolution Melt Analysis for Rapid Comparison of Bacterial Community Compositions, Appl. Environ. Microbiol., 2014, 80, 3568–3575 CrossRef PubMed.
- M. Sayed, Efficient removal of phenol from aqueous solution by the pulsed high-voltage discharge process in the presence of H2O2, Chem. Int., 2016, 1, 81–86 Search PubMed.
- D. J. Kim, D. I. Lee and J. Keller, Effect of temperature and free ammonia on nitrification and nitrite accumulation in landfill leachate and analysis of its nitrifying bacterial community by FISH, Bioresour. Technol., 2006, 97, 459–468 CrossRef CAS PubMed.
- S. J. You, Y. P. Tsai, B. C. Cho and Y. H. Chou, Metabolic influence of lead on polyhydroxyalkanoates (PHA) production and phosphate uptake in activated sludge fed with glucose or acetic acid as carbon source, Bioresour. Technol., 2011, 102, 8165–8170 CrossRef CAS PubMed.
- I. Ozturk, M. Altinbas, I. Koyuncu, O. Arikan and C. Gomecyangin, Advanced physico-chemical treatment experiences on young municipal landfill leachates, Waste Management, 2003, 23, 441–446 CrossRef CAS PubMed.
- H. W. Sun, X. T. Lü, X. F. Wei, H. N. Zhao, J. Ma and X. H. Fang, Synergetic Inhibitory Effect of Free Ammonia and Aeration Phase Length Control on the Activity of Nitrifying Bacteria, J. Environ. Sci., 2016, 37, 1075–1081 CAS.
- G. P. Sheng, H. Q. Yu and X. Y. Li, Extracellular polymeric substances (EPS) of microbial aggregates in biological wastewater treatment systems: a review, Biotechnol. Adv., 2010, 28, 882–894 CrossRef CAS PubMed.
- P. F. Xia, Q. Li, L. R. Tan, X. F. Sun, C. Song and S. G. Wang, Extracellular polymeric substances protect Escherichia coli from organic solvents, RSC Adv., 2016, 6, 59438–59444 RSC.
- X. Zheng, P. Sun, J. Lou, J. Cai, Y. Song, S. Yu and X. Lu, Inhibition of free ammonia to the granule-based enhanced biological phosphorus removal system and the recoverability, Bioresour. Technol., 2013, 148, 343–351 CrossRef CAS PubMed.
- X. Zheng, P. Sun, J. Lou, Z. Fang, M. Guo, Y. Song, X. Tang and T. Jiang, The long-term effect of nitrite on the granule-based enhanced biological phosphorus removal system and the reversibility, Bioresour. Technol., 2013, 132, 333–341 CrossRef CAS PubMed.
- Y. Liu and J. H. Tay, The Essential Role of Hydrodynamic Shear Force in the Formation of Biofilm and Granular Sludge, Water Res., 2002, 36, 1653–1665 CrossRef CAS PubMed.
- J. Luo, Y. Chen and L. Feng, Polycyclic Aromatic Hydrocarbon Affects Acetic Acid Production during Anaerobic Fermentation of Waste Activated Sludge by Altering Activity and Viability of Acetogen, Environ. Sci. Technol., 2016, 50, 6921–6930 CrossRef CAS PubMed.
- C. Kragelund, C. Levantesi, A. Borger, K. Thelen, D. Eikelboom, V. Tandoi, Y. Kong, J. Krooneman, P. Larsen and T. R. Thomsen, Identity, abundance and ecophysiology of filamentous bacteria belonging to the Bacteroidetes present in activated sludge plants, Microbiology, 2008, 154, 886–894 CrossRef CAS PubMed.
- Q. Kong, J. Zhang, M. Miao, L. Tian, N. Guo and S. Liang, Partial nitrification and nitrous oxide emission in an intermittently aerated sequencing batch biofilm reactor, Chem. Eng. J., 2013, 217, 435–441 CrossRef CAS.
- S. Juretschko, A. Loy, A. Lehner and M. Wagner, The microbial community composition of a nitrifying-denitrifying activated sludge from an industrial sewage treatment plant analyzed by the full-cycle rRNA approach, Syst. Appl. Microbiol., 2002, 25, 84–99 CrossRef CAS PubMed.
- S. Kondaveeti, S. H. Lee, H. D. Park and B. Min, Bacterial communities in a bioelectrochemical denitrification system: the effects of supplemental electron acceptors, Water Res., 2014, 51, 25–36 CrossRef CAS PubMed.
- H. Lu, K. Chandran and D. Stensel, Microbial ecology of denitrification in biological wastewater treatment, Water Res., 2014, 64, 237–254 CrossRef CAS PubMed.
- X. Han, Z. Wang, J. Ma, C. Zhu, Y. Li and Z. Wu, Membrane bioreactors fed with different COD/N ratio wastewater: impacts on microbial community, microbial products, and membrane fouling, Environ. Sci. Pollut. Res., 2015, 22, 11436–11445 CrossRef CAS PubMed.
- H. Papen and R. V. Berg, A Most Probable Number method (MPN) for the estimation of cell numbers of heterotrophic nitrifying bacteria in soil, Plant Soil, 1998, 199, 123–130 CrossRef CAS.
- C. Feng, L. Huang, Y. Hui, X. Yi and C. Wei, Simultaneous phenol removal, nitrification and denitrification using microbial fuel cell technology, Water Res., 2015, 76, 160–170 CrossRef CAS PubMed.
- J. W. B. Moir, L. C. Crossman, S. Spiro and D. J. Richardson, The purification of ammonia monooxygenase from Paracoccus denitrficans, FEBS Lett., 1996, 387, 71–74 CrossRef CAS PubMed.
- H. J. Schreier, N. Mirzoyan and K. Saito, Microbial diversity of biological filters in recirculating aquaculture systems, Curr. Opin. Biotechnol., 2010, 21, 318–325 CrossRef CAS PubMed.
- T. Liu, Y. J. Mao, Y. P. Shi and X. Quan, Start-up and bacterial community compositions of partial nitrification in moving bed biofilm reactor, Appl. Microbiol. Biotechnol., 2017, 101, 1–12 CrossRef PubMed.
- E. Bock, I. Schmidt, R. Stüven and D. Zart, Nitrogen loss caused by denitrifying Nitrosomonas cells using ammonium or hydrogen as electron donors and nitrite as electron acceptor, Arch. Microbiol., 1995, 163, 16–20 CrossRef CAS.
- V. V. Dawkar, U. U. Jadhav, G. S. Ghodake and S. P. Govindwar, Effect of inducers on the decolorization and biodegradation of textile azo dye Navy blue 2GL by Bacillus sp. VUS, Biodegradation, 2009, 20, 777–787 CrossRef CAS PubMed.
Footnote |
† Electronic supplementary information (ESI) available. See DOI: 10.1039/c7ra10751j |
|
This journal is © The Royal Society of Chemistry 2017 |