DOI:
10.1039/C7RA10650E
(Paper)
RSC Adv., 2017,
7, 53415-53421
Yixinshu ameliorates hippocampus abnormality induced by heart failure via the PPARγ signaling pathway
Received
26th September 2017
, Accepted 6th November 2017
First published on 21st November 2017
Abstract
Heart failure (HF) patients exhibit a wide range of cognitive and mood abnormalities, especially hippocampus abnormality. Unfortunately, there are few treatments for hippocampus abnormality with HF. Yixinshu (YXS), a traditional Chinese herbal medication, has been found to improve cardiac function in an animal model. However, the effect of treatment with YXS on the hippocampus abnormality induced by heart failure and the related mechanisms remain unclear. In this study, a coronary artery ligation model of HF was treated by the daily intragastric administration of YXS or saline for six weeks. Echocardiography and ELISA for BNP and NT-proBNP were used to determine cardiac function. Western blot assays were used to determine the protein levels of ZO-1, AQP4 and PPARγ. We found that YXS significantly improved cardiac function and reduced BNP and NT-proBNP. Meanwhile, YXS also protected the integrity of the blood brain barrier (BBB) and attenuated the inflammation of the hippocampus in rats with HF. Moreover, the reduction of the expression level of PPARγ was significant in rats with HF via treatment by YXS. In conclusion, long-term treatment with YXS may protect against heart failure and hippocampus abnormality induced by heart failure via the PPARγ pathway.
Introduction
Heart failure (HF) patients exhibit a wide range of cognitive and mood abnormalities, such as memory deficits, depression, and anxiety.1 A systematic review shows that the ratio of the odds for cognitive impairment was 1.62 among subjects with HF, which confirms the relationship between HF and cognitive impairment.2 The estimated prevalence of cognitive impairment is between 25% and 80%.3 The syndromes of cognitive impairment indicate the dysfunction of the central nervous system related to neural injury, specifically damage to the hippocampus. Since the hippocampus is a very vascular structure, it is vulnerable to changes in the morbidities of blood flow and hypoxemia during HF exacerbations.4,5 Injury to the hippocampus may contribute to the cognitive impairment accompanying HF, and it can impair the ability to carry out self-care. Patients with cognitive impairment may have poor learning ability, which may make them less likely to adhere to their medical regimens.6,7 Thus, cognitive impairment may reduce the quality of life in patients with HF. The hippocampal damage may be responsible for the cognitive and mood abnormalities commonly reported in HF patients. However, the mechanisms of damage to the hippocampus are unknown, but they may include several processes, such as inflammation. Unfortunately, there are few treatments for brain damage with HF, especially hippocampal damage. Therefore, treatments must be explored to protect, prevent, and intervene for brain damage with HF, especially hippocampal damage with HF.
Yixinshu (YXS) is derived from Sheng-Mai-San, which is a classical TCM prescription, and it is widely used in protecting tissues from oxidative damage due to heart disease and cerebral injury in China.8–10 YXS consists of seven common Chinese herbal medicines, Panax ginseng, Fructus Schisandra chinensis, Astragalus membranaceus, Radix Salviae miltiorrhizae, Radix Ophiopogonis, Rhizoma Chuanxiong, and Fructus Crataegi. YXS has been approved by the State Food and Drug Administration of China for treating coronary heart disease, angina pectoris, and HF. Increasing research shows that YXS is a multi-component oral drug with therapeutic effects on HF.11 Clinically, YXS was also used in treating the cognitive and mood abnormalities commonly reported with HF according to the traditional Chinese medicine theory of “treating heart and brain diseases with the same methods (Nao Xin Tong Zhi: NXTZ)”.12 However, based on animal models, the potential mechanism of YXS against brain abnormalities induced by heart failure remains unclear.
Firstly, to confirm the effects of YXS in brain abnormalities induced by heart failure, coronary artery ligation rat model was used to be performed. Systemic inflammation-derived pro-inflammatory chemokines, such as interleukin-1 beta (IL1-beta), TNF-alpha and interleukin-6 (IL-6), can cause a breach in the blood–brain barrier (BBB), which plays a key role in maintaining the specialized microenvironment of the central nervous system (CNS) and enabling communication with the systemic compartment.13–15 Zonula occludens-1 (ZO-1) plays an important role in mediating the link between the transmembrane tight junctions and the actin cytoskeleton, which is the most important structures of the BBB. Aquaporin-4 (AQP4) is a water channel protein that can reconcile the homeostasis of water in the BBB.16,17 Neuro-inflammation is a hallmark that induces alterations in brain structure and function in major depressive disorders.18 It has been reported that a high level of IL1-beta, a key mediator of inflammation, is expressed in the brain, particularly in the hippocampus, which plays a pivotal role in memory and mood regulation.19 TNF-alpha, another pro-inflammatory cytokine during HF-mediated neuro-inflammation, was associated impaired hippocampus-independent nonspatial episodic memory function.20 Thus, we detected the inflammatory cytokines IL1-beta, TNF-alpha and IL-6 to study.
Secondly, to provide insights into the potential mechanism of YXS, this study attempts to investigate the mechanism via bioinformatics technology. Although TCM implies sophisticated multi-target effects,21,22 the key targets of TCM to treat diseases were unclear due to their complex compositions. With the development of bioinformatics technology, BATMAN-TCM (available at http://bionet.ncpsb.org/batman-tcm), is an online bioinformatics analysis tool used to profile the candidate genes of TCM.23 In this study, we performed BATMAN-TCM to discover the key pathway of the effect of YXS on the hippocampus abnormality in rats with HF.
In this study, we investigated the effect of YXS on the hippocampus abnormality induced by heart failure and demonstrated that YXS could protect against heart failure and the hippocampus abnormality induced by heart failure via the PPARγ pathway.
Materials and methods
Coronary artery ligation model of HF
All animal procedures were approved by the Academy of Chinese Medical Science's Administrative Panel on Laboratory Animal Care and performed in accordance with institutional guidelines and ethics of committee of China Academy of Chinese Medical Sciences. The male Sprague Dawley rats (240–260 g, Charles River) were randomized to two groups: SHAM (n = 6) and HF groups. The coronary artery ligation model was induced in rats by coronary artery ligation, as previously described.24 In brief, rats were anesthetized with 1% sodium pentobarbital (0.5 ml/100 g) and ventilated through endotracheal intubation on a Zoovent ventilator (ALC-V8S, Shanghai Alcott Biotech Co., Ltd.). A left sided thoracotomy was performed in the 4th intercostal space. After the pericardium was opened, a 5.0 nylon suture was deliberately tied across the left anterior descending coronary artery. The sham group of rats underwent the same procedure except ligation. Four weeks after surgery, rats went through non-invasive echocardiography examination protocols. All rats with coronary artery ligation were randomly allocated in to the treatment groups (n = 6, per group). Then, YXS was administrated to rats for six weeks by oral administration at dosages of 800 mg kg−1 (as YXS-M group) and 1600 mg kg−1 (as YXS-H group), respectively. Valsartan (8 mg kg−1, as Val group) or saline (as model group) was given to rats for six weeks in the same way.
Drugs
Yixinshu was obtained from the Xinbang Pharmaceutical Co. Ltd. (Guizhou, China), which was extracted and concentrated from seven herbal medicines including Panax ginseng, Schisandra chinensis, Ophiopogon japonicus, Astragalus membranaceus, Salvia miltiorrhiza, Ligusticum wallichii, and Fructus Crataegi.
Echocardiography
Echocardiography was performed on all animals using a Visual Sonics Vevo 770 (Toronto, Canada) with a 17.4 MHz central frequency scan at various time points after surgery. Rats were anesthetized using oxygen and isoflurane (64% N2, 32% O2 and 4% isoflurane), and freely breathing animals were maintained using oxygen and isoflurane (1.75%) during the echocardiography. Long axis cine loops and M-mode images of the LV and atrium were acquired in the parasternal long axis. The left ventricular ejection fraction (LVEF; %) and left ventricular fractional shortening (LVFS; %) were measured off-line according to the guidelines accompanying the Vevo 770 system software.
Western blot assay
Protein fractions were isolated in ice-cold RIPA lysis buffer (Beyotime, China), and protein concentrations were determined using the enhanced bicinchoninic acid protein assay kit (Thermo, USA). Protein fractions were denatured in a loading buffer, and 40 μg of each sample was loaded into alternating lanes of 10% sodium dodecyl sulfate–polyacrylamide gel (SDS–PAGE). Protein blots were transferred to polyvinylidene fluoride membranes (Millipore, USA). After blocking with 5% non-fat milk, the blots were washed with a mixture of Tris-buffered saline and Tween 20 (TBST) and incubated overnight at 4 °C with one of the following appropriate primary antibodies: anti-actin (1
:
5000, Proteintech Group), anti-ZO-1 (1
:
1000, Proteintech Group), anti-AQP4 (1
:
1000, Proteintech Group) and PPARγ (1
:
1000, Proteintech Group). Membranes were washed with TBST and then incubated at room temperature for 1 h with an appropriate secondary antibody conjugated to horseradish peroxidase, followed by washing with TBST again. Finally, the protein blots were visualized using an enhanced chemiluminescence kit (Millipore, USA). Relative intensities of protein bands were measured using ImageJ.
Prediction of candidate targets for YXS
The herbs of YXS were submitted to BATMAN-TCM (http://bionet.ncpsb.org/batman-tcm), which is an online bioinformatics analysis tool for the discovery of TCM therapeutic mechanisms.23 Constituent chemical ingredients of the 7 herbs were uniformly retrieved according to the backstage database of BATMAN-TCM, and the candidate targets of these ingredients were predicted by the similarity-based target prediction method. To obtain excellent prediction performance, predicted candidate targets with a cutoff score larger than 20 were used for further functional enrichment analysis of KEGG pathways.25
Enzyme-linked immunosorbent assay (ELISA)
Commercial ELISA test kits were used in the assessment of the hippocampal samples and serum: rat IL1-beta ELISA kit (eBioscience, USA), rat TNF-alpha ELISA Kit (eBioscience, USA), rat IL-6 ELISA kit (eBioscience, USA), rat BNP ELISA kit (Novus, Hong Kong) and NT-proBNP ELISA kit (Novus, Hong Kong). Proteins extracts of the hippocampus were assayed in 10 μg using a rat IL1-beta ELISA kit, rat TNF-alpha ELISA kit and IL-6 ELISA kit according to the manufacturer's instructions. Serum was detected using the BNP ELISA kit and NT-proBNP ELISA kit according to the manufacturer's instructions. The ELISA kit consisted of a 96-well plate. The plates were read on a microplate reader (SpectraMax M5, USA) at 450 nm. The reactions were carried out in triplicate for each sample.
Data analysis
Statistical analysis was performed using Prism software version 6.0 (Graphpad Software, San Diego, California, USA). All values were presented as a mean ± SD. The student's t-test or 2-way repeated ANOVA measures were used for comparison. P < 0.05 was considered statistically significant.
Results
YXS improves the cardiac function of rats with heart failure
To investigate the effect of YXS on heart failure in rats, the LVEF and LVFS were detected by cardiac echography. As shown in Fig. 1, the results revealed that rats with HF in the model group had significantly lower LVEF (36.90 ± 7.22%) and LVFS (19.01 ± 4.17%) values at 4 weeks compared to the sham group (LVEF: 69.68 ± 1.33%; LVFS: 40.97 ± 1.06%), while YXS can significantly increase the LVEF (middle dose: 52.89 ± 8.42%; high dose: 59.90 ± 5.44%) and LVFS (middle dose: 28.98 ± 5.72%; high dose: 33.45 ± 3.95%) after administration compared to the model group (Fig. 1A and B), respectively.
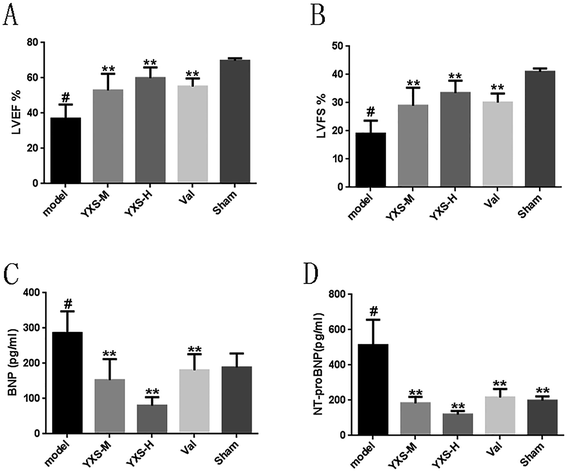 |
| Fig. 1 YXS improves the cardiac function of rats with heart failure. (A, B) Averaged data left ventricular ejection fraction (LVEF, A) and left ventricular fractional shortening (LVFS, B) assessed by echocardiography in rats. (C, D) Effects of YXS on BNP and NT-proBNP determined via ELISA assay. The plasma level of heart failure-related bio-marker including BNP (C), and NT-proBNP (D) were determined by ELISA assay in each group of rats vs. the model group, **P < 0.01; vs. the sham group, #P < 0.05, n = 4–6 rats per group. | |
It is known that BNP and NT-proBNP are two important hypertrophy markers in HF. Therefore, to examine whether BNP and NT-proBNP proteins were beneficially affected by YXS, secreted proteins of BNP and NT-proBNP in the serum were detected by ELISA. The levels of BNP and NT-proBNP in the serum were significantly increased in the model group (BNP: 286.25 ± 53.23 pg ml−1; NT-proBNP: 513.32 ± 125.12 pg ml−1), and they were also decreased by YXS treatment compared with the model group (BNP: middle dose: 152.37 ± 53.57 pg ml−1; high dose: 80.30 ± 21.43 pg ml−1; NT-proBNP: middle dose: 183.85 ± 32.21 pg ml−1; high dose: 119.88 ± 17.14 pg ml−1) (Fig. 1C and D).
YXS protects the hippocampus in rats with heart failure
In our study, the results of the western blotting assay revealed that the expressions of ZO-1 and AQP4 were downregulated in rats with HF. The expressions of ZO-1 and AQP4 were significantly upregulated in the YXS-treated group compared to the model group (Fig. 2A and B).
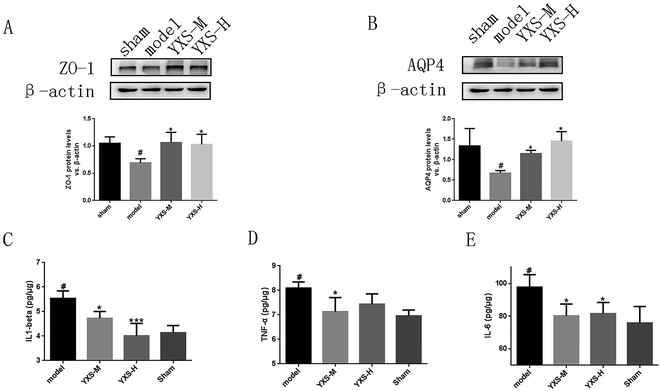 |
| Fig. 2 YXS protects the hippocampus in rats with heart failure. (A) Expression of the tight junction proteins ZO-1 in the hippocampi of rats. Original Western blots shown on the upper panels. β-Actin was used as a loading control. Bar graphs are gray values. (B) Expression of the proteins AQP4 in the hippocampus of rats. Original Western blots shown on the upper panels. β-Actin was used as a loading control. Bar graphs are gray values. (C–E) The levels of pro-inflammatory cytokines, IL1-beta, TNF-alpha and IL-6, were detected by ELISA. Data are mean ± SD. vs. model, *p < 0.05, ***<0.001; vs. sham, #P < 0.05. n = 4–6 animals per group. | |
Evolving evidence implies that the state of chronic peripheral inflammation reduces adult hippocampal neurogenesis.26 To investigate the effect of YXS on the inflammation of the hippocampus, ELSIA was performed to detect the levels of pro-inflammatory cytokines, such as IL1-beta, IL-6 and TNF-alpha. In our study, the results revealed that the levels of IL1-beta, IL-6 and TNF-alpha were upregulated in the serum and hippocampus of rats with HF. YXS treatment can significantly decrease the upregulated levels of IL1-beta, IL-6 and TNF-alpha of rats with HF compared to the model group (Fig. 2C–E).
The PPARγ pathway was enriched by BATMAN-TCM combined with KEGG analysis
For YXS's candidate compounds, only the predicted candidate target proteins with scores >= 20 are presented by BATMAN-TCM. Function enrichment results show that candidate targets of YXS tend to relate to atherosclerosis (adjusted p-value = 1.16 × 10−2), heart failure (adjusted p-value = 9.50 × 10−5), depression (adjusted p-value = 3.51 × 10−5) and anxiety disorders (adjusted p-value = 3.81 × 10−3), as shown in Fig. 3A. Excitingly, according to KEGG enrichment analysis, candidate targets were enriched in the PPAR pathway (adjusted p-value = 1.13 × 10−4) in which PPARγ plays important roles (Fig. 3B). Moreover, by Function2TCM in BATMAN-TCM (cutoff scores > 25), Panax ginseng and Schisandra chinensis were significantly associated with PPAR pathway (adjusted p-value = 1.74 × 10−4, 6.68 × 10−4 respectively). Combined the literature and BATMAN-TCM, herbs–compounds–targets network was constructed by Cytoscape (Fig. 3C). The degree of PPARγ in the network was 14 by network analysis in Cytoscape.
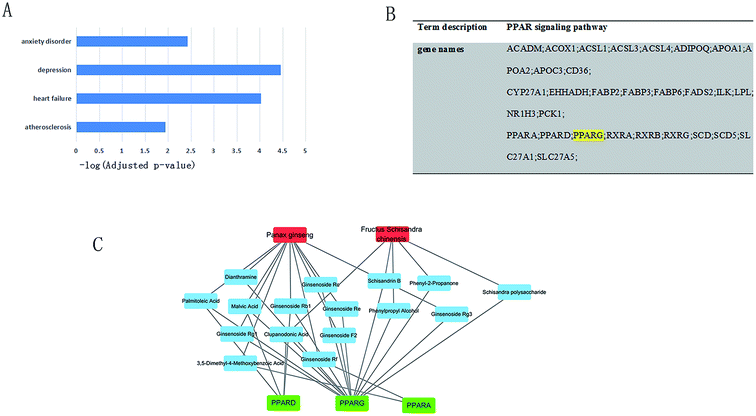 |
| Fig. 3 PPAR pathway was enriched by BATMAN-TCM combined with KEGG analysis. (A) Function enrichment diseases that candidate targets of YXS tent to relate. (B) KEGG enrichment analysis and candidate targets were enriched in PPAR pathway. (C) Herbs–compounds–targets network. Red rectangles refer to herbs in YXS, blue rectangles refer to compounds in YXS, green rectangles refer to targets in PPAR pathway. | |
YXS upregulated the expression of PPARγ in the hippocampus of rats with heart failure
For validation of the PPARγ pathway, a western blot assay was used to detect the expression of PPARγ in the hippocampus of rats with heart failure. As shown in Fig. 4, the expression of PPARγ in the model group was significantly reduced in rats in comparison with the sham group. Furthermore, the expression of PPARγ was significantly upregulated by YXS treatment (Fig. 4).
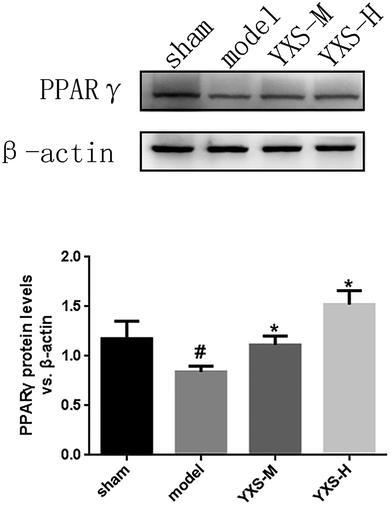 |
| Fig. 4 Expression of the proteins PPARγ in the hippocampi of rats. Original Western blots shown on the upper panels. β-Actin was used as a loading control. Bar graphs are gray values. Data are mean ± SD. *P < 0.05, vs. the model group; #P < 0.05, vs. the sham group, n = 3–4 rats per group. | |
Discussion
Up until now, the treatments for brain damage in HF, especially hippocampal damage, were limited. In the present study, the administration of YXS, a SMS-derived TCM formula, significantly improved cardiac function in rats subjected to hippocampal injury. These beneficial effects of YXS were associated with the upregulation of PPARγ in the heart compared with the model group. Furthermore, YXS activated the expression of PPARγ in the hippocampus compared with the model group. Taken together, our results imply that YXS is a potent TCM drug that reduces brain damage with HF by upregulating PPARγ.
In our previous studies, 276 compounds of YXS have been identified via molecular feature orientated precursor ion selection and tandem mass spectrometry structure elucidation, such as ginsenoside Rb1, ginsenoside Re, and ginsenoside Rg1 from Panax ginseng.27 As the chemical composition of YXS are mainly composed of the chemical substances from the involved single herbs, it is complex to predict and validate the mechanism of YXS protecting against heart failure and hippocampus abnormality by a large number of components in YXS preparations. In previous studies, YXS was focused on heart diseases, such as myocardial dysfunction.27 In this study, inflammatory cytokines, such as TNF-alpha, in the serum increased, consistent with the results previously reported.20,28 Moreover, YXS can increase the expression of ZO-1 and AQP4, which implies that YXS had a protective effect in maintaining the integrity of the BBB. On the other hand, YXS can significantly decrease the levels of IL1-beta, IL-6 and TNF-alpha in the hippocampus in rats with HF compared to the model group. Taken together, this results suggest that YXS have a protection on hippocampus induced by heart failure.
Although we investigated the effect of YXS on the hippocampus abnormality induced by heart failure, the mechanism of YXS protecting against heart failure and hippocampus abnormality was largely unknown. In this study, we used BATMAN-TCM to enrich the pathway relative to the hippocampus abnormality induced by HF. According to the herbs–compounds–targets network (Fig. 3C), we found that PPARγ was the key node in PPAR pathway regulated by Panax ginseng and Schisandra chinensis. In previous researches, PPARγ is a ligand-activated transcription factor that is emerging as a major regulator in HF.29,30 Increasing the ligands of PPARγ decreases inflammatory cytokines such as IL-6 and TNF-alpha.31 PPARγ agonists, such as pioglitazone and rosiglitazone, have protective effects against congestive heart failure in an animal model.30,32 In this study, we found that the PPAR pathway was enriched by BATMAN-TCM combined with KEGG analysis.
Moreover, PPARs regulate both inflammation and several other pathways related to neuro-inflammation,33,34 and neuro-inflammation supposedly contributes to neuronal degeneration. The up-regulation of PPARγ can attenuate neuro-inflammation in the hippocampus, subsequently decreasing the associated cognitive impairment.34 On the other hand, the activation of brain PPARγ in heart failure rats can reduce central inflammation and brain renin-angiotensin system activity, ameliorating angiotensin II-induced hypertension in heart failure.29,35 Thus, central PPARγ may be the therapeutic target in treating hippocampus damage in myocardial infarction-induced HF. In present study, we found that PPARγ was reduced in the hippocampus in rats with heart failure. Meanwhile, YXS could upregulate the expression of PPARγ. It has been reported that PPARγ regulated tight junction development during human urothelial cytodifferentiation, which implies that PPARγ plays a role in the BBB.36 In this study, the expression of ZO-1 belonging to tight junction proteins was upregulated by YXS. These results suggest that YXS may activate the PPAR pathway, subsequently to protect the BBB, finally prevent the hippopotamus abnormality induced by heart failure.
Accumulating evidence demonstrates that PPARγ has a protective effect in cardiovascular disease, such as enhancing fatty acid oxidation, improving endothelial cell function, and attenuating myocardial fibrosis.37–40 PPARγ agonists, such as thiazolidinediones (TZDs), have the potential to reduce inflammation, which is involved in all of the cellular and molecular mechanisms of cardiac fibrosis.41 However, largely due to the concomitant adverse effects of TZDs, the utility of TZDs in T2DM has declined in the past decade.42 More and more works have attempted to discover new and potent PPARγ agonists from natural products.43 In our previous study, we found that 62 compounds consist of 19 ginsenosides from Panax ginseng, 13 lignans from Fructus Schisandrae chinensis.27 Some ingredients of Panax ginseng and Fructus Schisandrae chinensis regulated the function of PPAR pathway.44–48 For example, it was reported that ginsenoside Re, a major ginsenoside in ginseng, could activate PPARγ to prevents angiotensin II-induced gap-junction remodeling in isolated beating rat atria.47 Schisandrin B, a dibenzocyclooctadiene lignan isolated from Schisandra chinensis, inhibited LPS-induced inflammatory response by activating PPARγ.46 PPARγ may be the common pathway in treating heart and brain diseases by YXS. However, we will continue to investigate the detailed mechanisms of PPARγ in heart failure and hippocampus abnormality.
There are several limitations of the current study. First, although our results demonstrated a beneficial effect of YXS against hippocampus and heart injury, the behavioral effects of YXS on HF remain unclear. Further sensitive behavioral testing may be warranted. Second, because there are several subunits of PPAR in different cell types, the effects of YXS on individual subunits of PPAR warrant investigation.
Collectively, our findings provide the first evidence suggesting that long-term treatment with YXS protects against heart failure and the hippocampus abnormality induced by heart failure via the PPARγ pathway. Our data provide important experimental data and rationale for future clinical trials and the application of YXS.
Conflicts of interest
The authors declare no conflict of interest.
Abbreviations
YXS | Yixinshu |
HF | Heart failure |
BNP | Brain natriuretic peptide |
NT-proBNP | N-terminal pro-brain natriuretic peptide |
ZO-1 | Zonula occludens-1 |
AQP4 | Aquaporin 4 |
PPAR | peroxisome proliferator-activated receptor |
Acknowledgements
We thank the financial support from the National Science and Technology Major Project (2014ZX09201021-009), National Program on Key Basic Research Project (2015CB554406), National Natural Science Foundation of China (81673700, 81603422 and 81703951), Science and Technology Cooperation Major Project of Guizhou Province of China ([2014]6019).
References
- M. A. Woo, J. A. Ogren, C. M. Abouzeid, P. M. Macey, K. G. Sairafian, P. S. Saharan, P. M. Thompson, G. C. Fonarow, M. A. Hamilton, R. M. Harper and R. Kumar, Eur. J. Heart Failure, 2015, 17, 494–500 CrossRef PubMed.
- R. L. Vogels, P. Scheltens, J. M. Schroeder-Tanka and H. C. Weinstein, Eur. J. Heart Failure, 2007, 9, 440–449 CrossRef PubMed.
- J. Cameron, R. Gallagher and S. J. Pressler, Curr. Heart Failure Rep., 2017, 14, 13–22 CrossRef PubMed.
- D. T. Balu and I. Lucki, Neurosci. Biobehav. Rev., 2009, 33, 232–252 CrossRef PubMed.
- S. Zola-Morgan, L. R. Squire and D. G. Amaral, J. Neurosci., 1986, 6, 2950–2967 CAS.
- J. Cameron, L. Worrall-Carter, K. Page, B. Riegel, S. K. Lo and S. Stewart, Eur. J. Heart Failure, 2010, 12, 508–515 CrossRef PubMed.
- S. Alkhuja and K. Duffy, Heart Lung, 2013, 42, 387 Search PubMed.
- C. Z. Chai, W. L. Mo, X. F. Zhuang, J. P. Kou, Y. Q. Yan and B. Y. Yu, J. Evidence-Based Complementary Altern. Med., 2016, 2016, 4682786 Search PubMed.
- G. S. Cao, S. X. Li, Y. Wang, Y. Q. Xu, Y. N. Lv, J. P. Kou and B. Y. Yu, Chin. J. Nat. Med., 2016, 14, 508–517 Search PubMed.
- G. Q. Zhang, H. Wang, W. T. Liu, H. Dong, W. F. Fong, L. M. Tang, Y. H. Xiong, Z. L. Yu and K. M. Ko, Rejuvenation Res., 2008, 11, 991–1000 CrossRef CAS PubMed.
- Y. Zhao, L. Xu, Z. Qiao, L. Gao, S. Ding, X. Ying, Y. Su, N. Lin, B. He and J. Pu, Sci. Rep., 2016, 6, 23025 CrossRef CAS PubMed.
- D. Chen, S. H. Tang, P. Lu and H. J. Yang, Zhongguo Zhongyao Zazhi, 2015, 40, 4288–4296 Search PubMed.
- A. Varatharaj and I. Galea, Brain, Behav., Immun., 2017, 60, 1–12 CrossRef CAS PubMed.
- G. J. Schenk and H. E. de Vries, Drug Discovery Today: Technol., 2016, 20, 5–11 CrossRef PubMed.
- D. Kempuraj, R. Thangavel, G. P. Selvakumar, S. Zaheer, M. E. Ahmed, S. P. Raikwar, H. Zahoor, D. Saeed, P. A. Natteru, S. Iyer and A. Zaheer, Front. Cell. Neurosci., 2017, 11, 216 CrossRef PubMed.
- H. Ikeshima-Kataoka, Int. J. Mol. Sci., 2016, 17, 1306 CrossRef PubMed.
- H. Wolburg, S. Noell, K. Wolburg-Buchholz, A. Mack and P. Fallier-Becker, Neuroscientist, 2009, 15, 180–193 CrossRef CAS PubMed.
- Y. K. Kim and E. Won, Behav. Brain Res., 2017, 329, 6–11 CrossRef CAS PubMed.
- S. J. Tsai, Cytokine Growth Factor Rev., 2017, 37, 89–97 CrossRef CAS PubMed.
- A. Meissner, N. P. Visanji, M. A. Momen, R. Feng, B. M. Francis, S. S. Bolz and L. N. Hazrati, J. Am. Heart Assoc., 2015, 4, e001920 CrossRef PubMed.
- N. J. Sucher, Expert Opin. Drug Discovery, 2013, 8, 21–34 CrossRef CAS PubMed.
- L. Li, F. Bonneton, X. Y. Chen and V. Laudet, Mol. Cell. Endocrinol., 2015, 401, 221–237 CrossRef CAS PubMed.
- Z. Liu, F. Guo, Y. Wang, C. Li, X. Zhang, H. Li, L. Diao, J. Gu, W. Wang, D. Li and F. He, Sci. Rep., 2016, 6, 21146 CrossRef CAS PubMed.
- M. A. Pfeffer, J. M. Pfeffer, M. C. Fishbein, P. J. Fletcher, J. Spadaro, R. A. Kloner and E. Braunwald, Circ. Res., 1979, 44, 503–512 CrossRef CAS PubMed.
- M. Kanehisa, S. Goto, M. Furumichi, M. Tanabe and M. Hirakawa, Nucleic Acids Res., 2010, 38, D355–D360 CrossRef CAS PubMed.
- V. Chesnokova, R. N. Pechnick and K. Wawrowsky, Brain, Behav., Immun., 2016, 58, 1–8 CrossRef CAS PubMed.
- M. Zhang, H. Wu, F. Guo, Y. Yu, J. Wei, Y. Geng, S. Wang, S. Li and H. Yang, Mol. BioSyst., 2017, 13, 1469–1480 RSC.
- M. Kobusiak-Prokopowicz, B. Jolda-Mydlowska, T. Grzebieniak, K. Poczatek and A. Mysiak, Adv. Clin. Exp. Med., 2015, 24, 987–994 CrossRef PubMed.
- M. Chandra, S. Miriyala and M. Panchatcharam, PPAR Res., 2017, 2017, 6404638 CrossRef PubMed.
- H. H. Liao, X. H. Jia, H. J. Liu, Z. Yang and Q. Z. Tang, Curr. Pharm. Des., 2017, 23, 1677–1686 CrossRef CAS PubMed.
- M. Ricote, A. C. Li, T. M. Willson, C. J. Kelly and C. K. Glass, Nature, 1998, 391, 79–82 CrossRef CAS PubMed.
- S. R. Giri, B. Bhoi, M. R. Jain and M. M. Gatne, Life Sci., 2016, 162, 1–13 CrossRef CAS PubMed.
- J. Iglesias, L. Morales and G. E. Barreto, Mol. Neurobiol., 2017, 54, 2518–2538 CrossRef CAS PubMed.
- Z. Zhang, H. Yuan, H. Zhao, B. Qi, F. Li and L. An, Int. Immunopharmacol., 2017, 43, 53–61 CrossRef CAS PubMed.
- Y. Yu, B. J. Xue, S. G. Wei, Z. H. Zhang, T. G. Beltz, F. Guo, A. K. Johnson and R. B. Felder, Hypertension, 2015, 66, 403–411 CrossRef CAS PubMed.
- C. L. Varley, M. A. Garthwaite, W. Cross, J. Hinley, L. K. Trejdosiewicz and J. Southgate, J. Cell. Physiol., 2006, 208, 407–417 CrossRef CAS PubMed.
- T. A. Ajith and T. G. Jayakumar, Clin. Exp. Pharmacol. Physiol., 2016, 43, 649–658 CrossRef CAS PubMed.
- S. Vattulainen-Collanus, O. Akinrinade, M. Li, M. Koskenvuo, C. G. Li, S. P. Rao, V. de Jesus Perez, K. Yuan, H. Sawada, J. W. Koskenvuo, C. Alvira, M. Rabinovitch and T. P. Alastalo, J. Cell Sci., 2016, 129, 693–705 CrossRef CAS PubMed.
- A. AlSaleh, T. A. Sanders and S. D. O'Dell, Proc. Nutr. Soc., 2012, 71, 141–153 CrossRef CAS PubMed.
- Y. Peng, Y. Zeng, J. Xu, X. L. Huang, W. Zhang and X. L. Xu, Eur. J. Pharmacol., 2016, 791, 105–114 CrossRef CAS PubMed.
- H. J. Liu, H. H. Liao, Z. Yang and Q. Z. Tang, PPAR Res., 2016, 2016, 2198645 Search PubMed.
- I. Goltsman, E. E. Khoury, J. Winaver and Z. Abassi, Pharmacol. Ther., 2016, 168, 75–97 CrossRef CAS PubMed.
- R. Chen, J. Wan, J. Song, Y. Qian, Y. Liu and S. Gu, Pharm. Biol., 2017, 55, 503–509 CrossRef CAS PubMed.
- D. Jin, T. Zhao, W. W. Feng, G. H. Mao, Y. Zou, W. Wang, Q. Li, Y. Chen, X. T. Wang, L. Q. Yang and X. Y. Wu, Int. J. Biol. Macromol., 2016, 87, 555–562 CrossRef CAS PubMed.
- H. H. Kwok, P. Y. Yue, N. K. Mak and R. N. Wong, Biochem. Pharmacol., 2012, 84, 532–539 CrossRef CAS PubMed.
- N. Liu, J. X. Zheng, Y. S. Zhuang, Z. K. Zhou, J. H. Zhao and L. Yang, Inflammation, 2017, 40, 1006–1011 CrossRef CAS PubMed.
- B. Zhang, X. Cui, H. H. Jin, L. Hong, X. Liu, X. Li, Q. G. Zhang and L. P. Liu, Life Sci., 2017, 190, 36–45 CrossRef CAS PubMed.
- L. Zhang, M. Zhu, M. Li, Y. Du, S. Duan, Y. Huang, Y. Lu, J. Zhang, T. Wang and F. Fu, Oncotarget, 2017, 8, 55384–55393 Search PubMed.
Footnote |
† These authors contributed equally to this work. |
|
This journal is © The Royal Society of Chemistry 2017 |