DOI:
10.1039/C7RA10529K
(Paper)
RSC Adv., 2017,
7, 50822-50828
Asymmetric synthesis of δ-amino acid derivatives via diastereoselective vinylogous Mannich reactions between N-tert-butanesulfinyl imines and dioxinone-derived lithium dienolate†
Received
22nd September 2017
, Accepted 26th October 2017
First published on 1st November 2017
Abstract
A diastereoselective vinylogous Mannich reaction between the N-tert-butanesulfinyl imines and dioxinone-derived lithium dienolate was developed. A variety of aldimines, ketimines and isatin-derived ketimines are suitable for this process. On the basis of X-ray crystallography of products, a predictive model for this transformation was provided.
Introduction
Chiral δ-amino acids and their derivatives are commonly found in many bioactive natural alkaloids, drugs, and drug candidates,1–4 e.g. the vasodilator vincamine,1 the α2-adrenoceptor antagonist yohimbine,2 clinically important anticancer drugs vinblastine and vincristine,3 and the antibiotic cephalosporin C4 (Fig. 1). In addition, chiral δ-amino acid derivatives 1 are useful building blocks for asymmetric organic synthesis (Scheme 1),5–8 in particular for the synthesis of chiral δ-amino β-ketoesters 2 which are versatile intermediates for various piperidine and pyrrolidine alkaloids.5 As a consequence, some effective methods have been developed towards the synthesis of chiral δ-amino acid derivatives 1.6–8
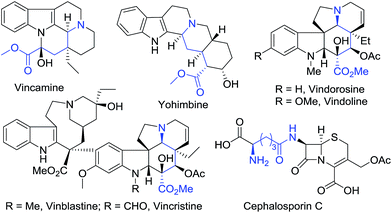 |
| Fig. 1 Selected compounds containing chiral δ-amino acid units. | |
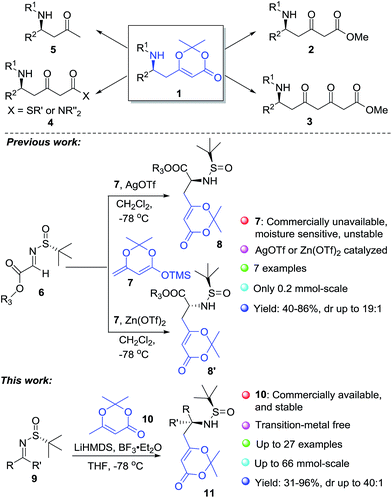 |
| Scheme 1 Application and synthesis of δ-amino acid derivatives. | |
In our recent efforts towards the synthesis of vindoline and vindorosine (Fig. 1),9 we needed a scalable process (>10 g) for the preparation of chiral δ-amino acid derivatives 11 as starting materials. Because the chiral tert-butanesulfinamide has been widely used as a versatile reagent for the asymmetric introduction of amine units,10,11 we planned to synthesize the chiral δ-amino acid derivatives 1 based on vinylogous Mannich reactions between N-tert-butanesulfinyl imines and dioxinone-derived dienolate (Scheme 1). Although an elegant vinylogous Mannich reaction between N-tert-butanesulfinyl imines 6 and dioxinone-derived silyl dienolate 7 mediated by Lewis acid has been reported by Chen's group (Scheme 1),7b the moisture-sensitive and unstable silyl dienolates6–8,12,13 were not deemed suitable for large-scale preparation in our laboratory. After a few experiments, we could not get the desired products in large quantities. Therefore, we decided to develop a practical process using dioxinone-derived lithium dienolates directly as nucleophiles.
In this paper, we report full details of the general and practical diastereoselective approach towards the synthesis of δ-amino acid derivatives in gram-scale by vinylogous Mannich reactions between N-tert-butanesulfinyl imines and dioxinone-derived lithium dienolate.
Results and discussion
We began our research using indolyl N-tert-butanesulfinyl aldimine 9a and commercially available dioxinone 10 as substrates. A systematic screening of reaction conditions was conducted to optimize the yield and diastereoselectivity of the vinylogous Mannich reaction (Table 1). Initially, the reactions were carried out in the presence of bases without adding any Lewis acid (entry 1 and 2). Treatment of 10 with lithium diisopropylamide (LDA) in tetrahydrofuran (THF) at −78 °C followed by sulfinyl aldimine 9a resulted in a complex mixture, with the desired product being isolated in only 8% yield.
Table 1 Optimization of the vinylogous Mannich reactiona
Changing the base to lithium bis(trimethylsilyl)amide improved the yield to 27%, unfortunately, both the yield and stereoselectivity were unacceptable (entry 2). Introductions of transition metal Lewis acids to activate the imines were attempted but failed to provide the desired products. To our delight, the same reaction conducted in the presence of BF3·Et2O provided the desired products in 65% yield (entry 7, 11a + 11a′). Finally the optimized reaction conditions were found (entries 8). Mannich addition of dioxinone-derived lithium dienolate (2.0 eq.) towards sulfinyl aldimine in the presence of BF3·Et2O (1.0 eq.) provided desired product (11a) and its diastereomer (11a′) in 87% and 2% isolated yields (1 mmol scale) respectively (entry 8). The reactions could be scaled up to 5 mmol, 14.9 mmol and 66.0 mmol without significant loss in yields and diastereoselectivity of the desired products (entries 9–11).
With the optimized reaction conditions in hand, the scope of the diastereoselective vinylogous Mannich reactions between N-tert-butanesulfinyl imines 9 and dioxinone-derived lithium dienolate (generated in situ from 10) activated by BF3·Et2O was investigated (Table 2). For substrate 6-methoxyindolyl N-tert-butanesulfinyl aldimine 9b, major product 11b and its diastereomer were obtained in 87% yield and with high d.r. (>40
:
1). For phenyl N-tert-butanesulfinyl aldimine 9c, excellent yield (96%) and diastereoselectivity (>40
:
1 d.r.) were obtained (Table 2, entry 2). Next, the influence of the substitution at the aromatic rings (9d–9h) was examined (entries 3–7). All these aldimines afforded good to excellent yields and diastereoselectivity. The electron rich 3,4-dimethoxybenzaldimine 9d gave the best result, which provided product 11d in 95% yield and >40
:
1 d.r. (Table 2, entry 3). The substrate 9h bearing an electron deficient nitro group also afforded the corresponding product (11h) in 89% yield and high diastereoselectivity (entry 7, >40
:
1). Benzofuran-derived N-tert-butanesulfinyl aldimine 9i and quinoline-derived N-tert-butanesulfinyl aldimine 9j were also investigated (entries 8 and 9). Benzofuran-derived N-tert-butanesulfinyl aldimine 9i provided the expected product in 80% yield and 10.4
:
1 d.r. (Table 2, entry 8).
Table 2 Aldimines in the vinylogous Mannich reactiona
It was of interests to note that the quinoline-derived N-tert-butanesulfinyl aldimine required two equivalents of BF3·Et2O and the corresponding product 11j could be isolated in 86% yield and >40
:
1 d.r. (Table 2, entry 9). This interesting result indicated that the first equivalent of BF3 might coordinate to the more basic quinoline nitrogen and the second equivalent of BF3 activated the sulfinyl aldimine in this vinylogous Mannich reaction. Changing the substrate to aliphatic butanesulfinyl aldimine 9k resulted in 37% yield and moderate diastereoselectivity (Table 2, entry 10, 6.4
:
1). The low isolated yield was mainly caused by decomposition of aliphatic N-tert-butanesulfinyl aldimine in the presence of Lewis acid.
Next, the phenyl N-tert-butanesulfinyl ketimines 9l–9q were examined (Table 3). Due to the thermal sensibility and hydrolytical instability,11e substrates 9l–9q provided the corresponding products 11l–11q in low yields and with moderate to excellent diastereoselectivities. For the phenylethanimine 9l, only 1.2
:
1 d.r. was observed (Table 3, entry 1). However, treatment of the phenyl cyclic ketimines 9m–9q under the same optimized reaction conditions resulted in mild to excellent diastereoselectivities (d.r. 2
:
1 to >40
:
1, entries 2–6). Although the yields were moderate, to the best of our knowledge, these were the first reported examples on diastereoselective vinylogous Mannich reaction of N-tert-butanesulfinyl ketimines.
Table 3 Ketimines in the vinylogous Mannich reactiona
Isatin, a valuable building block for the synthesis of diverse biologically active compounds, has attracted considerable attention in chemical society.14 It was our interests to use it as starting material in the diastereoselective vinylogous Mannich reactions. The isatin-derived N-tert-butanesulfinyl ketimines (9r–9aa) were then prepared and reacted with dioxinone-derived lithium dienolate (Scheme 2).
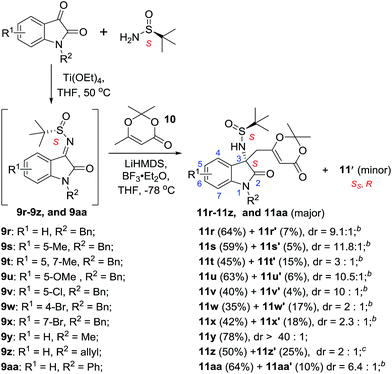 |
| Scheme 2 Isatin-derived ketimines in the vinylogous Mannich reaction.a Yields represent isolated yields for two steps. Reactions were conducted at 1.0 mmol scale in THF at −78 °C (acetone-dry ice bath). (Ss)-tert-Butanesulfinamide was served in all reactions.b Major products and their diastereomers could be isolated by flash column chromatography.c Major products and their diastereomers could be isolated by preparative chromatography. Bn = benzyl, Ph = phenyl. | |
For isatin-derived ketimine 9r, good yield (71%) and high level of diastereoselectivity (9.1
:
1 d.r.) were observed (Scheme 2). The isatin-derived ketimines (9s, 9u and 9v) bearing one substituted group (methyl, methoxyl, or chloro) at the 5-position usually gave the products (11s, 11u and 11v) with better diastereoselectivities. While the isatin-derived ketimines (9t, 9w and 9x) bearing substituted group at the 4-position or 7-position resulted in moderate isolated yields with lower diastereoselectivities (11t, 11w and 11x).
Finally, the influence of the substitution at 1-position (N-substituent) was investigated. As a result, the methyl-substituted substrate (9y) provided the best result in terms of yields and diastereoselectivities (11y, 78% yield, >40
:
1 d.r.).
For this BF3·Et2O-mediated vinylogous Mannich reaction, we provided a transition state model for predicting the absolute configuration of the products (Fig. 2). The imine was activated by coordination with BF3·Et2O and Si-face addition of the dioxinone-derived lithium dienolate leads to the major products with S-configuration for the newly formed stereo center. X-ray crystallographic experiments established the absolute configurations of 11c, 11h and 11w (Fig. 3), and the absolute configurations of 11c, 11h and 11w were determined to be (Rs, R), (Ss, S) and (Ss, S), respectively.15 The X-ray crystallographic experimental results are in fully accordance with our model prediction.
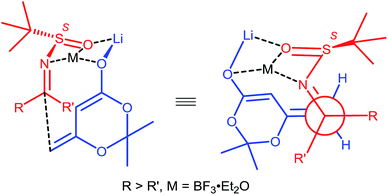 |
| Fig. 2 Proposed transition state model for the vinylogous Mannich reaction between (S)–N-tert-butanesulfinyl imines and dioxinone-derived lithium dienolate. | |
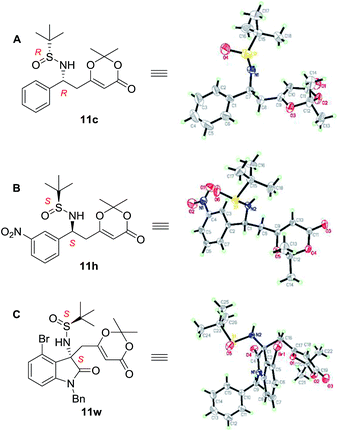 |
| Fig. 3 X-ray crystal structures of compound 11c, 11h and 11w. Thermal ellipsoids are shown at 30% probability. | |
Conclusions
In conclusion, we have established a general and diastereoselective method for the synthesis of δ-amino acid derivatives. The BF3·Et2O mediated vinylogous Mannich reactions between N-tert-butanesulfinyl imines and dioxinone-derived lithium dienolate are practical and could be conducted in gram-scale. To validate our method as a means to access complex natural products, the asymmetric total synthesis of (−)-vindorosine has been accomplished based on this procedure recently.9 This new approach should find more applications in the synthesis of complex natural products and drug candidates.
Experimental
General
Melting points were obtained on a XT-4 melting-point apparatus and were uncorrected. The infrared (IR) spectra were measured on a Nicolet iS10 FTIR spectrometer with 4 cm−1 resolution and 32 scans between wavenumber of 4000 cm−1 and 400 cm−1. Samples were prepared as KBr disks with 1 mg of samples in 100 mg of KBr. Proton nuclear magnetic resonance (1H-NMR) spectra were obtained on a Bruker Avance 400 spectrometers at 400 MHz. Carbon-13 nuclear magnetic resonance (13C-NMR) was obtained on Bruker Avance 400 spectrometers at 100 MHz. Chemical shifts are reported as δ values in parts per million (ppm) relative to tetramethylsilane (TMS) for all recorded NMR spectra. High Resolution Mass spectra were taken on AB QSTAR Pulsar mass spectrometer or Aglient LC/MSD TOF mass spectrometer. Optical rotations were recorded on a JASCO P-2000 polarimeter. All new compounds were characterized by IR, 1H NMR, 13C NMR and HRMS. The known compounds were characterized by 1H NMR and 13C NMR. Silica gel (200–300 mesh) for column chromatography and silica GF254 for TLC were produced by Merck Chemicals Co. Ltd. (Shanghai). THF used in the reactions were dried by distillation over metallic sodium and benzophenone. Starting materials and reagents used in reactions were obtained commercially from Acros, Aldrich, Adamas-beta®, and were used without purification, unless otherwise indicated. All moisture-sensitive reactions were conducted in dried glassware under a positive pressure of dry nitrogen or argon. Reagents and starting materials were accordingly transferred via syringe or cannula. Unless otherwise stated, all other reactions were also performed under a dry nitrogen atmosphere.
General procedure for aldimines and phenyl ketimines (11a–11q)
To a solution of sulfinimines 9 (1.0 mmol) in THF (10 mL) was added BF3·Et2O (0.13 mL, 1.0 mmol) at −78 °C. In the mean time, to a stirred solution of dioxinone 10 (284 mg, 2.0 mmol) in THF (10 mL) was added lithium bis(trimethylsilyl)amide (1.0 M in THF, 2.4 mL, 2.4 mmol) at −78 °C. The resulting mixtures were stirred at −78 °C for 1 h. Then, the solution of dioxinone-derived lithium dienolate was cannulated to the solution of sulfinimine at −78 °C. Stirring was continued at −78 °C for 3 h. The reaction was then quenched with saturated aq. NH4Cl (3 mL) at −78 °C. The cooling bath was removed and the mixture was warmed to rt, followed by extraction with EtOAc (3 × 15 mL). The combined organic layers were washed with brine (15 mL), dried over Na2SO4, filtered, and concentrated under reduced pressure. The residue was purified by flash chromatography eluting with petroleum ether/EtOAc (5
:
1 to 2
:
1) or purified by preparative chromatography to afford sulfinamides 11 and their diastereomer 11′. Caution: 2 mmol BF3·Et2O was added for quinoline-derived N-tert-butanesulfinyl aldimine 9j. See ESI† file for characterization data.
General procedure for isatin-derived ketimines (11r–11aa)
A mixture of isatin-derived substrate (1.0 mmol), (S)-tert-butanesulfonamine (146 mg, 1.2 mmol) and titanium ethoxide (1.6 mL, 2.5 mmol) in dichloromethane (10 mL) was stirred under reflux for 12 h. The reaction was quenched with water and the resulting suspension was filtered through a short pad of anhydrous sodium sulfate. The solid cake was washed with dichloromethane, and the separated organic layer was dried over anhydrous sodium sulfate, filtered, and concentrated under reduced pressure. The resulting residue was dissolved in THF (10 mL). BF3·Et2O (0.13 mL, 1 mmol) was added at −78 °C. In the mean time, to a stirred solution of dioxinone 10 (284 mg, 2.0 mmol) in THF (10 mL) was added lithium bis(trimethylsilyl)amide (1.0 M in THF, 2.4 mL, 2.4 mmol) at −78 °C. The resulting mixtures were stirred at −78 °C for 1 h. Then, the solution of dioxinone-derived lithium dienolate was cannulated to the solution of sulfinimine at −78 °C. Stirring was continued at −78 °C for 3 h. The reaction was then quenched with saturated aq. NH4Cl (3 mL) at −78 °C. The cooling bath was removed and the mixture was warmed to rt, followed by extraction with EtOAc (3 × 15 mL). The combined organic layers were washed with brine (15 mL), dried over Na2SO4, filtered, and concentrated under reduced pressure. The residue was purified by flash chromatography eluting with petroleum ether/EtOAc (5
:
1 to 2
:
1) or purified by preparative chromatography to afford isatin-derived sulfinamides and their diastereomer. Yields represent isolated yields for two steps. See ESI† file for characterization data.
Conflicts of interest
There are no conflicts of interest to declare.
Acknowledgements
This work was supported by grants from the Program for Changjiang Scholars and Innovative Research Team in University (IRT17R94), Natural Science Foundation of China (21332007, 20925205, 21762047 and 20832005), YunLing scholar of Yunnan Province, Yunnan Provincial Science & Technology Department (2016FD007 and 2017FB010), the Excellent Young talents Program of Yunnan University, and the Program for Innovative Research Team (in Science and Technology) in University of Yunnan Province.
Notes and references
-
(a) A. G. Schultz, W. P. Malachowski and Y. Pan, J. Org. Chem., 1997, 62, 1223–1229 CrossRef CAS;
(b) S. Hagstadius, Psychopharmacology, 1984, 83, 321 CrossRef CAS PubMed.
- P. Mayer, P. Brunel, C. Chaplain, C. Piedecoq, F. Calmel, P. Schambel, P. Chopin, T. Wurch, P. J. Pauwels, M. Marien, J.-L. Vidaluc and T. Imbert, J. Med. Chem., 2000, 43, 3653–3664 CrossRef CAS PubMed.
-
(a) J. W. Moncrief and W. N. Lipscomb, Acta Crystallogr., 1966, 21, 322–331 CrossRef CAS PubMed;
(b) H. Ishikawa, D. A. Colby, S. Seto, P. Va, A. Tam, H. Kakei, T. J. Rayl, I. Hwang and D. L. Boger, J. Am. Chem. Soc., 2009, 131, 4904–4916 CrossRef CAS PubMed.
-
(a) M. Toth, N. T. Antunes, N. K. Stewart, H. Frase, M. Bhattacharya, C. A. Smith and S. B. Vakulenko, Nat. Chem. Biol., 2016, 12, 9–14 CrossRef CAS PubMed;
(b) A. Sarkar, K. C. Anderson and G. E. Kellogg, Eur. J. Med. Chem., 2012, 52, 98–110 CrossRef CAS PubMed;
(c) A. Felici, M. Perilli, N. Franceschini, G. M. Rossolini, M. Galleni, J.-M. Frere, A. Oratore and G. Amicosante, Antimicrob. Agents Chemother., 1997, 41, 866–868 CAS.
-
(a) F. A. Davis, B. Chao, T. Fang and J. M. Szewczyk, Org. Lett., 2000, 2, 1041–1043 CrossRef CAS PubMed;
(b) F. A. Davis and B. Chao, Org. Lett., 2000, 2, 2623–2625 CrossRef CAS PubMed;
(c) F. A. Davis, T. Fang, B. Chao and D. M. Burns, Synthesis, 2000, 2016–2112 Search PubMed;
(d) F. A. Davis, B. Chao and A. Rao, Org. Lett., 2001, 3, 3169–3171 CrossRef CAS PubMed;
(e) F. A. Davis, T. Fang and R. Goswami, Org. Lett., 2002, 4, 1599–1602 CrossRef CAS PubMed;
(f) F. A. Davis, A. Rao and P. J. Carroll, Org. Lett., 2003, 5, 3855–3857 CrossRef CAS PubMed;
(g) F. A. Davis, Y. Zhang and G. Anilkumar, J. Org. Chem., 2003, 68, 8061–8064 CrossRef CAS PubMed;
(h) F. A. Davis, J. Zhang, Y. Li, H. Xu and C. DeBrosse, J. Org. Chem., 2005, 70, 5413–5419 CrossRef CAS PubMed;
(i) F. A. Davis, N. Theddu and P. M. Gaspari, Org. Lett., 2009, 11, 1647–1650 CrossRef CAS PubMed.
- Q. Wang, M. van Gemmeren and B. List, Angew. Chem., Int. Ed., 2014, 53, 13592–13595 CrossRef CAS PubMed.
-
(a) C.-L. Gu, L. Liu, D. Wang and Y.-J. Chen, Synth. Commun., 2009, 39, 2989–3002 CrossRef CAS;
(b) C.-L. Gu, L. Liu, D. Wang and Y.-J. Chen, J. Org. Chem., 2009, 74, 5754–5757 CrossRef CAS PubMed.
- R. Kawęcki, Tetrahedron, 2001, 57, 8385–8390 CrossRef.
- W. Chen, X.-D. Yang, W.-Y. Tan, X.-Y. Zhang, X.-L. Liao and H. Zhang, Angew. Chem., Int. Ed., 2017, 56, 12327–12331 (Angew. Chem., 2017, 129, 12495–12499) CrossRef CAS PubMed.
- For recent reviews, see:
(a) G. Liu, D. A. Cogan and J. A. Ellman, J. Am. Chem. Soc., 1997, 119, 9913–9914 CrossRef CAS;
(b) D. A. Cogan, G. Liu and J. A. Ellman, Tetrahedron, 1999, 55, 8883–8904 CrossRef CAS;
(c) J. A. Ellman, T. D. Owens and T. P. Tang, Acc. Chem. Res., 2002, 35, 984–995 CrossRef CAS PubMed;
(d) J. A. Ellman, Pure Appl. Chem., 2003, 75, 39–46 CrossRef CAS;
(e) P. Zhou, B.-C. Chen and F. A. Davis, Tetrahedron, 2004, 60, 8003–8030 CrossRef CAS;
(f) C. H. Senanayake, D. Krishnamurthy, Z.-H. Lu, Z. Han and I. Gallou, Aldrichimica Acta, 2005, 38, 93–104 CAS;
(g) D. Morton and R. A. Stockman, Tetrahedron, 2006, 62, 8869–8905 CrossRef CAS;
(h) F. Ferreira, C. Botuha, F. Chemla and A. Perez-Luna, Chem. Soc. Rev., 2009, 38, 1162–1186 RSC;
(i) M. R. Robak, M. A. Herbage and J. A. Ellman, Chem. Rev., 2010, 110, 3600–3740 CrossRef CAS PubMed and references therein.
-
(a) Y. Zhou, Y. Xi, J. Zhao, X. Sheng, S. Zhang and H. Zhang, Org. Lett., 2012, 14, 3116–3119 CrossRef CAS PubMed;
(b) W. Chen, J. Ren, M. Wang, L. Dang, X. Shen, X. Yang and H. Zhang, Chem. Commun., 2014, 50, 6259–6262 RSC;
(c) X. Shen, Y. Zhou, Y. Xi, J. Zhao and H. Zhang, Nat. Prod. Bioprospect., 2016, 6, 117–139 CrossRef CAS PubMed;
(d) L. Cui, C. Li and L. Zhang, Angew. Chem., Int. Ed., 2010, 49, 9178–9181 CrossRef CAS PubMed;
(e) W. Zhang and S. R. Gilbertson, Tetrahedron Lett., 2017, 58, 2175–2177 CrossRef CAS;
(f) J. A. Sirvent, F. Foubelo and M. Yus, Eur. J. Org. Chem., 2013, 2461–2471 CrossRef CAS;
(g) M. J. García-Muñoz, H. K. Dema, F. Foubelo and M. Yus, Tetrahedron: Asymmetry, 2014, 5, 362–372 CrossRef;
(h) J. A. Sirvent, F. Foubelo and M. Yus, Chem. Commun., 2012, 48, 2543–2545 RSC;
(i) A. A. Reddy, P. O. Reddy and K. R. Prasad, J. Org. Chem., 2016, 81, 11363–11371 CrossRef CAS PubMed;
(j) V. M. Foley, C. M. McSweeney, K. S. Eccles, S. E. Lawrence and G. P. McGlacken, Org. Lett., 2015, 17, 5642–5645 CrossRef CAS PubMed;
(k) Q. Chen, C. Chen, F. Guo and W. Xia, Chem. Commun., 2013, 49, 6433–6435 RSC;
(l) A. Kościołowicz and M. D. Rozwadowska, Tetrahedron: Asymmetry, 2006, 17, 1444–1448 CrossRef;
(m) S.-L. Cai, B.-H. Yuan, Y.-X. Jiang, G.-Q. Lin and X.-W. Sun, Chem. Commun., 2017, 53, 3520–3523 RSC.
- R. Villano, M. R. Acocella, A. Massa, L. Palombi and A. Scettri, Tetrahedron, 2007, 63, 12317–12323 CrossRef CAS.
- For selected recent reviews of vinylogous Mannich reactions, see:
(a) B. Karimi, D. Enders and E. Jafari, Synthesis, 2013, 45, 2769–2812 CrossRef CAS;
(b) M. S. Roselló, C. del Pozo and S. Fustero, Synthesis, 2016, 48, 2553–2571 CrossRef and references therein.
- For selected recent reviews on isatin, see:
(a) R. Moradi, G. M. Ziarani and N. Lashgari, ARKIVOC, 2017, 148–201 CAS;
(b) G. S. Singh and Z. Y. Desta, Chem. Rev., 2012, 112, 6104–6155 CrossRef CAS PubMed;
(c) M. A. Borad, M. N. Bhoi, N. P. Prajapati and H. D. Patel, Synth. Commun., 2014, 44, 897–922 CrossRef CAS;
(d) M. A. Borad, M. N. Bhoi, N. P. Prajapati and H. D. Patel, Synth. Commun., 2014, 44, 1043–1057 CrossRef CAS;
(e) N. R. Ball-Jones, J. J. Badillo and A. K. Franz, Org. Biomol. Chem., 2012, 10, 5165–5181 RSC;
(f) M. Flores, J. Pena, P. G. García, N. M. Garrido and D. Díez, Curr. Org. Chem., 2013, 17, 1957–1985 CrossRef CAS;
(g) Y. Liu, H. Wang and J. Wan, Asian J. Org. Chem., 2013, 2, 374–386 CrossRef CAS and references therein.
- Dr Jie Zhou in Yunnan University is gratefully acknowledged for X-ray crystallography analyses of compounds 11c, 11h, and 11w. CCDC 1573541 (11c), 1573540 (11h), and 1573542 (11w) contain the supplementary crystallographic data for this paper.†.
Footnote |
† Electronic supplementary information (ESI) available. CCDC 1573540–1573542. For ESI and crystallographic data in CIF or other electronic format see DOI: 10.1039/c7ra10529k |
|
This journal is © The Royal Society of Chemistry 2017 |
Click here to see how this site uses Cookies. View our privacy policy here.