DOI:
10.1039/C7RA10318B
(Paper)
RSC Adv., 2017,
7, 56204-56210
Preparation of a new 2D MXene/PES composite membrane with excellent hydrophilicity and high flux
Received
17th September 2017
, Accepted 21st November 2017
First published on 13th December 2017
Abstract
MXene, a new 2D transition metal carbide-based material, possesses excellent electrical conductivity and hydrophilicity. In this study, Ti3C2Tx (where T represents a functional group (O, OH, and/or F)) was produced by etching and ultrasonicating Ti3AlC2. Then, it was used to prepare the MXene composite membrane via a simple filtration method performed at 0.2 MPa on a polyethersulfone (PES) ultrafiltration membrane. The MXene composite membrane shows excellent flux (115 L m−2 h−1) and favorable rejection to Congo red dye (92.3% at 0.1 MPa). The membrane demonstrated rejection to inorganic salts below 23% with flux above 432 L m−2 h−1 at 0.1 MPa. Due to its loose lamellar structure, the composite membrane is able to demonstrate efficient permselectivity in the separation of dyes from salts. Furthermore, the composite membrane shows excellent hydrophilicity and flux because of the lamellar hydrophilic MXene.
1. Introduction
Polymer membranes have been widely used in the process of wastewater treatment and desalination due to their excellent permselectivity, mechanical properties, and relative low cost.1 In order to further improve the thermal resistance, fouling resistance and other performance parameters of such polymer membranes, substantial effort has been made including crosslinking,2,3 hydrophilic modification of membrane material4 and fabrication of mixed-matrix membranes (MMMs).5–7 A heterogeneous MMM consists of an inorganic filler embedded in a polymer matrix and combines the superior permeability and selectivity of inorganic membranes with the processability of polymeric membranes; thus, it shows great advantages in membrane-based gas separations and pervaporation.8 Graphene oxide (GO) membranes have been demonstrated to function as effective barriers for gas and liquid separations.9–12 Several breakthroughs relating to GO-based membranes have been achieved, demonstrating that GO is a promising membrane material for gas and liquid separation because of its distinctive 2D structure. However, the flux of GO membranes is relatively low because of the small interlayer spacing that results under pressure; for example, the flux of the GO membrane in ref. 10 is about 1702 g m−2 h−1 under vacuum conditions. A hydration effect would destroy the hydrogen bonds within the membrane and its layer spacing would become larger. Therefore, the separation performance of GO membrane would be unstable.
Zhang et al. developed a crosslinking method to enhance the flux of GO membranes without sacrificing the rejection rate. The GO-IPDI membrane exhibited a high flux of 80–100 L (m2 h bar)−1 under a low external pressure (1.0 bar). It exhibited a high rejection rate (above 96%) for Congo red dye.13 Recently, a large family of 2D materials named ‘MXene’ has attracted significant research interest because they exhibit hydrophilic surfaces, good structural and chemical stability and excellent electrical conductivity.14–16 MXenes are a type of layered 2D materials, which are often produced with MAX phase (where M represents an early transition metal, A corresponds to III A or IV A group elements, and X is C or N) powders in HF solutions at ambient temperatures, followed by Al etching. Nowadays, Ti3C2Tx (where T represents O, OH, and/or F groups while x is the number of the terminating groups) is more commonly studied because it is easier to prepare; the synthesis involves etching Ti3AlC2 with HF at room temperature. Compared with GOs, MXene materials have superior chemical stability and thermal resistance. They have been widely utilized in the fabrication of super capacitors,17 lithium-ion batteries,18 and for heavy metal adsorption because of the layered structure of the MXene have large surface areas.19 Barsoum et al. prepared the polymer-Ti3C2Tx composite nanofibers via electrospinning.20 Nanometer-thin sheets of 2D MXene were assembled into freestanding or supported membranes for the selective rejection of ions and molecules. Micrometer-thick MXene membranes demonstrated ultra-fast water flux of 37.4 L (bar·h m2)−1 and differential sieving of salts based on the hydration radius and charge of the ions.21 Wu et al. used Ti3C2Tx nanosheets as nanofillers to prepare a SRNF composite membrane. When this membrane was incorporated into two typical polymer matrixes, hydrophilic polyethyleneimine (PEI) and hydrophobic polydimethylsiloxane (PDMS), the uniformly dispersed Ti3C2Tx nanosheets enhanced the thermal/mechanical stabilities and solvent resistance of the polymer-based membranes.22 Wang et al. proposed a type of 2D lamellar membrane consisting of Ti3C2Tx MXene nanosheets and nanosized Fe(OH)3 supported on an anode, aluminum oxide (AAO) substrate. The MXene membrane with an extremely short transport pathway and large amounts of nanochannels showed excellent water permeance (more than 1000 L m−2 h−1) and a favorable rejection rate (over 90%) for molecules with sizes around 2.5 nm using a filter flask under a vacuum of 0.1 MPa.23 Herein, the Ti3C2Tx was produced by etching and ultrasonication of Ti3AlC2 and utilized to prepare the MXene composite membrane by a simple filtration method at 0.5 MPa on a flexible polyethersulfone (PES) ultrafiltration membrane, thus broadening the range of application. The prepared membrane has extremely high flux and high rejection to Congo red dye. Simultaneously, the membrane preparation process can be easily scaled up and the functional layer is very stable to hydraulic flush.
2. Experimental
2.1. Materials and instruments
The average particle size of the Ti3AlC2 used in the experiment is about 3 μm (98%, Beijing Fusiman). A PES ultrafiltration membrane with MWCO (10
000) was prepared using the phase inversion method. The concentration of the hydrofluoric acid (HF) solution used was 49 wt% (Aladdin). All other chemicals were of analytical grade and used without further purification.
2.2. Membrane preparation
The Ti3C2Tx was produced by etching of Ti3AlC2 with 49% HF at 50 °C for 4 h, followed by filtration. Then, the filtrate was neutralized to at least pH 6 and dried in the air at room temperature. Further, it was delaminated in DMSO via ultrasonication for 20 h. Then, the solution was diluted to 1 g L−1 and filtered through a PES UF membrane in a dead-end membrane set-up. The filtration was processed at 0.2 MPa with continuous stirring. The membrane area was determined to be 48 cm2 and the influences on the membrane performance and morphology were studied by adjusting the MXene content.
2.3. Membrane characterization
The performances of the membranes are primarily described by their product water flux, J, and rejection, R. The membranes were characterized in a dead-end membrane module after they were pretreated under a pressure of 0.3 MPa for 30 min. The membrane performances including water flux, salt rejection (1 g L−1) and dye rejection (100 ppm) were measured under a pressure of 0.2 MPa at 20 °C. The permeation flux, F (L m−2 h−1), is calculated as follows: |
 | (1) |
where V is the total volume of the water or solution permeated during the experiment; A (m2) is the membrane area; and t (h) is the operation time. Rejection, R, is calculated using the following equation: |
 | (2) |
where Cp (g L−1) and Cf (g L−1) are the concentrations of the permeate solution and the feed solution, respectively. All the experiments to determine flux and rejection were repeated three times. The relation standard deviation of the data was lower than 15%. The contact angle of the membrane surface was tested with a contact angle meter (WCA, SL200KB). Membrane rejection was tested with a conductivity meter (DDS-11A, Shanghai rex electric chemical instrument co., LTD) and UV-vis Spectrophotometer (721, Shanghai Youke). The material and membrane morphology was observed using scanning electronic microscopy (SEM, NanoSEM 450, FEI), and main elements were detected using INCA EDX.
3. Results and discussion
3.1. Characterization of the membrane material MXene
The Ti3C2Tx was produced by etching Ti3AlC2 with 40% HF at room temperature for 12 h, followed by filtration. Then, the filtrate was neutralized to at least pH 6 and dried in the air at room temperature. The Ti3C2Tx was filtered through a PES membrane supported with a non-woven fabric to obtain MXene/PES composite membrane as shown in Fig. 1.
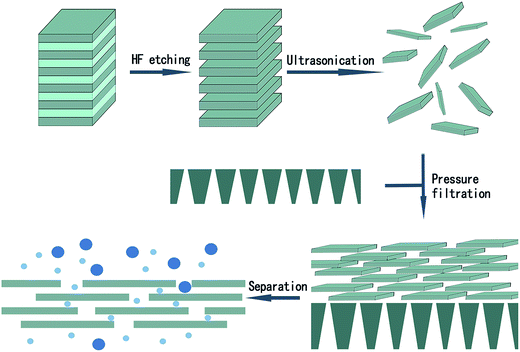 |
| Fig. 1 Schematic of the preparation and separation of the MXene/PES composite membrane. | |
The morphology of the material was observed using SEM (Fig. 2). The particles of Ti3AlC2 are very dense with layered structures and the layers are clearly connected. Following treatment, the Al layer of Ti3AlC2 was reacted with HF. It can be observed that the lamellar Ti3C2Tx was successfully produced with enlarged interplanar spacing.
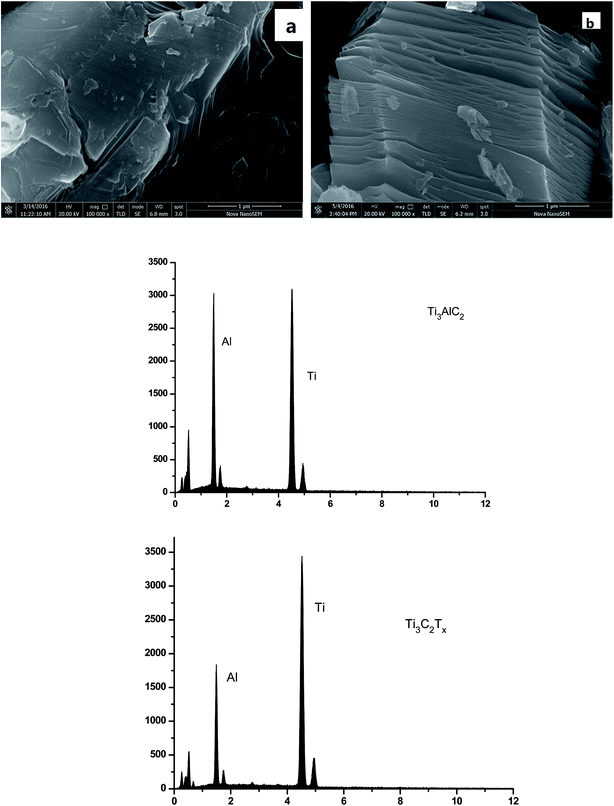 |
| Fig. 2 The morphology and EDS image of the materials: (a) Ti3AlC2; (b) Ti3C2Tx. | |
EDS results, indicating the variation in Al/Ti atomic ratio, is another powerful evidence reflecting etching effect. As shown in Fig. 2, the amount of elemental Al decreased clearly after the treatment with HF. The Al/Ti atomic ratio declined from 0.7 to about 0.41 after treatment, indicating the etching of the Al from Ti3AlC2.
3.2. Characterization of the as-prepared MXene composite membrane
In order to prepare the MXene/PES composite membrane, MXene was delaminated in DMSO via ultrasonication for 20 h. Then, the solution was diluted to 1 g L−1 and filtered through a PES UF membrane at 0.2 MPa with continuous stirring. The membranes were prepared with different content of MXene. The constitution and contact angle of the membrane are listed in Table 1. It is found that the water drop would immediately spread over the membrane surface. This could occur because the MXene has excellent hydrophilicity for the –OH group and the nano-sized channels in the MXene sheets would absorb significant amounts of water.
Table 1 Substance constitution and contact angle of the composite membrane
Membrane |
M0 |
M1 |
M2 |
M3 |
M4 |
MXene content/g |
0 |
0.1 |
0.15 |
0.2 |
0.25 |
Contact angle/° |
89 |
0 |
0 |
0 |
0 |
The morphologies of the composite membranes are shown in Fig. 3. The composite membranes display good flexibility and the functional layer would not peel off the support membrane under ambient temperature. The top surface of the composite membrane, under 10
000× magnification, reveals the rough structure. Most of the particles are closely stacked, inferring the good permselectivity of the composite membrane. With an increase in MXene content, the morphology changes slightly since the same membrane material is used. As shown in Fig. 3, the inorganic particles are stacked within the surface of PES membrane with the non-woven fabric. The thickness of the functional layer is increased, which, in turn, increases the mass transfer resistance and decreases the defects of the composite membrane.
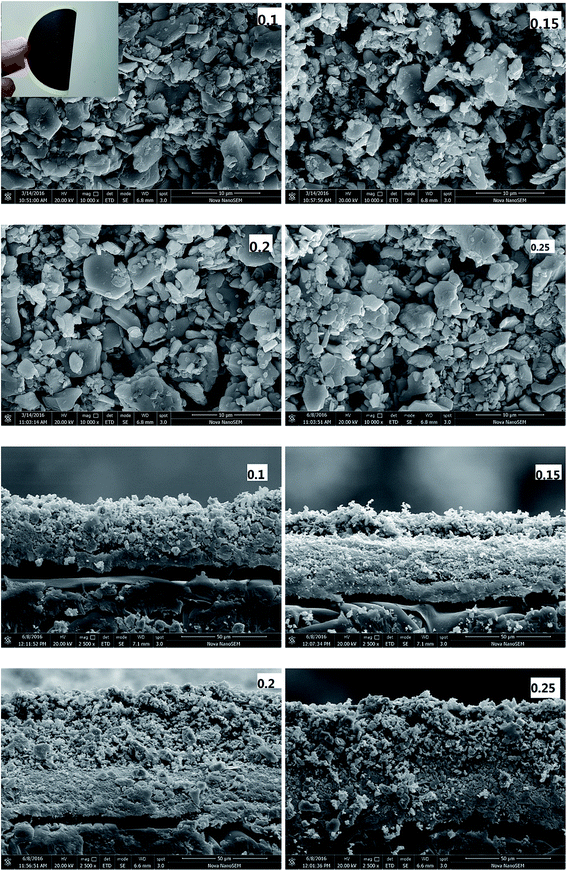 |
| Fig. 3 The top surface and cross-section morphology of the prepared MXene/PES composite membrane: 0.1, the MXene membrane prepared with 0.1 g Ti3C2Tx; the inset is the top surface morphology of the membrane; 0.15, the MXene membrane prepared with 0.15 g Ti3C2Tx; 0.2, the MXene membrane prepared with 0.2 g Ti3C2Tx; 0.25, the MXene membrane prepared with 0.25 g Ti3C2Tx. | |
The membrane top surface of M3 was scanned by EDX and the elemental maps are shown in Fig. 4. The elemental maps show that all elements are distributed homogeneously with the content of element Ti shown to be sufficient. Al is also observed in the elemental map probably because the inner Al was not completely etched by the HF due to its low concentration.
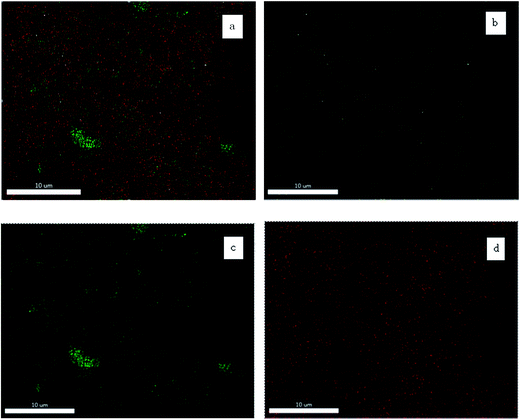 |
| Fig. 4 The elemental maps of EDS of M3 with the same scale bar: (a) C, Al and Ti; (b) C; (c) Al; (d) Ti. | |
3.3. The performance of the MXene composite membrane
As shown in Fig. 5, the performance of membranes with different MXene contents was investigated and along with that of the supporting membrane. The 100 ppm Congo red dye (697 Da) solution was used as the testing solution. The supporting membrane has a rejection of 10.7% to the dye and the flux is about 114.9 L m−2 h−1 at 0.1 MPa. With the increase in MXene content, the rejection to dye clearly elevated; the membrane flux initially increases and then declines. The supporting membrane possesses satisfactory rejection to dye and low flux. This can be interpreted by the hydrophobic nature of the membrane causing the adsorption of the dye molecules to reduce the flux of the membrane. The introduction of MXene particles into the membrane surface improves the hydrophilicity of the membrane and significantly lowers the membrane fouling. Only a few dye molecules are adsorbed in the membrane pores. Therefore, the membrane flux initially improves. With a further increase in MXene content, the resistance of mass transfer increases and the membrane flux is decreases. When the MXene content is increased to 0.2 g, the membrane shows high rejection (92.3%) and high flux (115 L m−2 h−1) due to the dense and defect-free functional layer (Fig. 6–8).
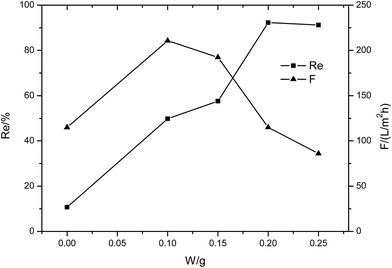 |
| Fig. 5 The performance of the MXene membrane with different MXene contents (100 ppm Congo red dye, 0.1 MPa). | |
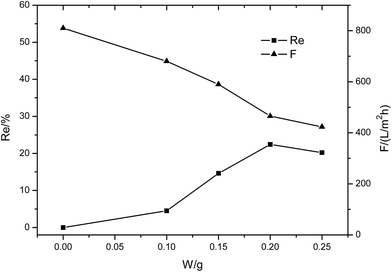 |
| Fig. 6 The performance of the MXene membrane with different MXene contents (1 g L−1 MgCl2 solution, 0.1 MPa). | |
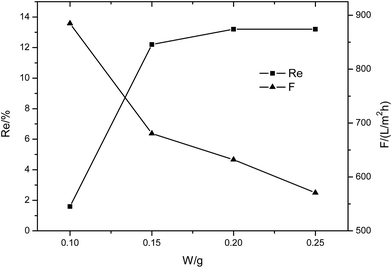 |
| Fig. 7 The performance of the MXene membrane with different MXene contents (1 g L−1 Na2SO4 solution, 0.1 MPa). | |
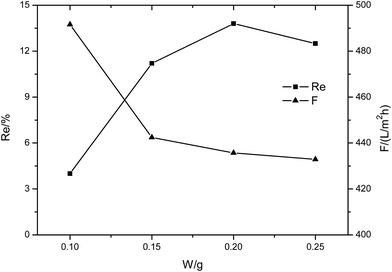 |
| Fig. 8 The performance of the MXene membrane with different MXene contents (1 g L−1 NaCl solution, 0.1 MPa). | |
The membranes were also tested with 1 g L−1 MgCl2 solution at 0.1 MPa. It was found that the supporting membrane has no rejection to the inorganic salt MgCl2 and the membrane flux is very high (about 810 L m−2 h−1). When MXene was introduced in the membrane surface, the rejection to MgCl2 increased, but remained below 23%, while the flux clearly declined with MXene content. At the same operating pressure, the membrane flux for MgCl2 is about 4 to 6 times that tested with the dye solution. This phenomenon is common because the dye molecules would accumulate in the membrane pores, thus improving the flow resistance substantially. The high flux of the composite membrane indicates that the MXene membrane exhibits a very loose lamellar structure, which provides a good flow channel for the solution.
The membranes were further tested with Na2SO4 and NaCl solutions to analyze the separation performance of the composite membrane. The membranes showed high flux (above 432 L m−2 h−1) and low rejection to inorganic salts at 0.1 MPa. The flux decreases and rejection to salts increases with an increase in MXene content.
The MXene composite membrane prepared with 0.2 g MXene has relatively high permselectivity. Therefore, it was tested with another dye, Gentian violet (408 Da). In this case, the membrane also showed high rejection (80.3%) and flux (about 117.6 L m−2 h−1). As shown in Table 2, the membrane demonstrates large difference in the rejection of the dye molecule and that of the inorganic salt. Therefore, it is very highly suitable for dye desalination and wastewater treatment.
Table 2 Performance of the MXene composite membrane with 0.2 g MXene (0.1 MPa)
Feed |
Congo red |
Gentian violet |
MgCl2 |
Na2SO4 |
NaCl |
F (L m−2 h−1) |
115 |
117.6 |
460 |
632 |
435 |
R (%) |
92.3 |
80.3 |
23 |
13.2 |
13.8 |
4. Conclusions
In conclusion, a type of a 2D MXene/PES composite membrane based on stacks of MXene nanosheets was prepared successfully by filtration on a PES UF membrane at 0.2 MPa. The membrane material was characterized using SEM and EDX. The prepared Ti3C2Tx has a delaminated structure and a low Al/Ti ratio after HF etching. The MXene membranes have rough and dense surface layers and uniform element distributions. The MXene membrane exhibits an increased rejection to dyes and inorganic salts with the increase in MXene content. When the MXene is fixed at 0.2 g, the membrane shows high rejections to Congo red dye (92.3%) and Gentian violet (80.3%) and high flux (above 115 L m−2 h−1) at 0.1 MPa. The membrane's rejection to inorganic salts is below 23% with flux above 432 L m−2 h−1 at 0.1 MPa, which indicates that the membrane can be used with extremely high efficiency in dye desalination and wastewater treatment.
Conflicts of interest
There are no conflicts to declare.
Acknowledgements
The authors wish to thank the support of Natural Science Foundation of China (No: 51503089), the Fundamental Research Funds for the Central Universities (DUT16RC(3)049), the State Key Laboratory of Fine Chemicals (KF 1402).
References
- I. G. Wenten and Khoiruddin, Reverse osmosis applications: Prospect and challenges, Desalination, 2016, 391, 112–125 CrossRef CAS.
- Y. Yao, C. Ba, S. Zhao, W. Zheng and J. Economy, Development of a positively charged nanofiltration membrane for use in organic solvents, J. Membr. Sci., 2016, 520, 832–839 CrossRef CAS.
- K. Vanherck, G. Koeckelberghs and I. F. J. Vankelecom, Crosslinking polyimides for membrane applications: a review, Prog. Polym. Sci., 2013, 38, 874–896 CrossRef CAS.
- Y. Liu, H. Huang, P. Huo and J. Gu, Exploration of zwitterionic cellulose acetate antifouling ultrafiltration membrane for bovine serum albumin (BSA) separation, Carbohydr. Polym., 2017, 165, 266–275 CrossRef CAS PubMed.
- B. M. Ganesh, A. M. Isloor and A. F. Ismail, Enhanced hydrophilicity and salt rejection study of graphene oxide-polysulfone mixed matrix membrane, Desalination, 2013, 313, 199–207 CrossRef CAS.
- X.-H. Ma, Z. Yang, Z.-K. Yao, Z.-L. Xu and C. Y. Tang, A facile preparation of novel positively charged MOF/chitosan nanofiltration membranes, J. Membr. Sci., 2017, 525, 269–276 CrossRef CAS.
- R. Zhang, S. Ji, N. Wang, L. Wang, G. Zhang and J. R. Li, Coordination-driven in situ self-assembly strategy for the preparation of metal-organic framework hybrid membranes, Angew. Chem., Int. Ed., 2014, 53, 9775–9779 CrossRef CAS PubMed.
- R. Lin, L. Ge, S. Liu, V. Rudolph and Z. Zhu, Mixed-matrix membranes with metal-organic framework-decorated CNT fillers for efficient CO2 separation, ACS Appl. Mater. Interfaces, 2015, 7, 14750–14757 CAS.
- R. K. Joshi, P. Carbone, F. C. Wang, V. G. Kravets, Y. Su, I. V. Grigorieva, H. A. Wu, A. K. Geim and R. R. Nair, Precise and Ultrafast Molecular Sieving Through Graphene Oxide Membranes, Science, 2014, 343, 752–754 CrossRef CAS PubMed.
- K. Huang, G. Liu, Y. Lou, Z. Dong, J. Shen and W. Jin, A Graphene Oxide Membrane with Highly Selective Molecular Separation of Aqueous Organic Solution, Angew. Chem., Int. Ed., 2014, 53, 6929–6932 CrossRef CAS PubMed.
- Q. Zhao, J. Hou, J. Shen, J. Liu and Y. Zhang, Long-lasting antibacterial behavior of a novel mixed matrix water purification membrane, J. Mater. Chem. A, 2015, 3, 18696–18705 CAS.
- J. Zhu, M. Tian, J. Hou, J. Wang, J. Lin, Y. Zhang, J. Liu and B. Van der Bruggen, Surface zwitterionic functionalized graphene oxide for a novel loose nanofiltration membrane, J. Mater. Chem. A, 2016, 4, 1980–1990 CAS.
- P. Zhang, J. L. Gong, G. M. Zeng, C. H. Deng, H. C. Yang, H. Y. Liu and S. Y. Huan, Cross-linking to prepare composite graphene oxide-framework membranes with high-flux for dyes and heavy metal ions removal, Chem. Eng. J., 2017, 322, 657–666 CrossRef CAS.
- M. Naguib, M. Kurtoglu, V. Presser, J. Lu, J. Niu, M. Heon, L. Hultman, Y. Gogotsi and M. W. Barsoum, Two-dimensional nanocrystals produced by exfoliation of Ti3AlC2, Adv. Mater., 2011, 23, 4248–4253 CrossRef CAS PubMed.
- M. Naguib, J. Come, B. Dyatkin, V. Presser, P.-L. Taberna, P. Simon, M. W. Barsoum and Y. Gogotsi, MXene: a promising transition metal carbide anode for lithium-ion batteries, Electrochem. Commun., 2012, 16, 61–64 CrossRef CAS.
- A. Feng, Y. Yua, F. Jiang, Y. Wang, L. Mi, Y. Yu and L. Song, Fabrication and thermal stability of NH4HF2-etched Ti3C2 MXene, Ceram. Int., 2017, 43, 6322–6328 CrossRef CAS.
- Z. Ling, C. E. Ren, M.-Q. Zhao, J. Yang, J. M. Giammarco, J. Qiu, M. W. Barsoum and Y. Gogotsi, Flexible and conductive MXene films and nanocomposites with high capacitance, Proc. Natl. Acad. Sci. U. S. A., 2014, 111, 16676–16681 CrossRef CAS PubMed.
- M. Naguib, J. Come, B. Dyatkin, V. Presser, P.-L. Taberna, P. Simon, M. W. Barsoum and Y. Gogots, MXene: a promising transition metal carbide anode for lithium-ion batteries, Electrochem. Commun., 2012, 16, 61–64 CrossRef CAS.
- O. Mashtalir, K. M. Cook, V. N. Mochalin, M. Crowe, M. W. Barsoum and Y. Gogotsi, Dye adsorption and decomposition on two-dimensional titanium carbide in aqueous media, J. Mater. Chem. A, 2014, 2, 14334–14338 CAS.
- E. A. Mayerberger, O. Urbanek, R. M. McDaniel, R. M. Street, M. W. Barsoum and C. L. Schauer, Preparation and characterization of polymer-Ti3C2Tx (MXene) composite nanofibers produced via electrospinning, J. Appl. Polym. Sci., 2017, 134, 1–7 CrossRef.
- C. E. Ren, K. B. Hatzell, M. Alhabeb, Z. Ling, K. A. Mahmoud and Y. Gogotsi, Charge- and Size-Selective Ion Sieving Through Ti3C2Tx MXene Membranes, J. Phys. Chem. Lett., 2015, 6, 4026–4031 CrossRef CAS PubMed.
- X. Wu, L. Hao, J. Zhang, X. Zhang, J. Wang and J. Liu, Polymer-Ti3C2Tx composite membranes to overcome the trade-off in solvent resistant nanofiltration for alcohol-based system, J. Membr. Sci., 2016, 515, 175–188 CrossRef CAS.
- L. Ding, Y. Wei, Y. Wang, H. Chen, J. Caro and H. Wang, A Two-Dimensional Lamellar Membrane: MXene Nanosheet Stacks, Angew. Chem., Int. Ed., 2017, 56, 1825–1829 CrossRef CAS PubMed.
|
This journal is © The Royal Society of Chemistry 2017 |