DOI:
10.1039/C7RA10090F
(Paper)
RSC Adv., 2017,
7, 49299-49302
Visible-light induced tandem radical cyanomethylation and cyclization of N-aryl acrylamides: access to cyanomethylated oxindoles†
Received
11th September 2017
, Accepted 16th October 2017
First published on 23rd October 2017
Abstract
A visible-light induced cyanomethylation of N-aryl acrylamides with bromoacetonitrile followed by intramolecular cyclization has been explored. This transformation exhibits a wide substrate scope and significant functional group tolerance, providing a facile synthetic approach and highly efficient access to cyanomethylated oxindoles.
Oxindoles are an ubiquitous heterocycles motif in many natural products, pharmaceuticals and agrochemicals.1 Moreover, oxindoles have significant biological activities and wide-ranging applications in organic synthesis.2 Therefore, the search for sustainable and more efficient methods for the preparation of oxindoles is of constant interest. Recently, catalytic difunctionalization of alkenes emerged as an attractive strategy for accessing structurally diverse heterocyclic compounds,3 for example, a tandem radical addition/cyclization of N-aryl acrylamides that provides an elegant method for the construction of the 3,3-disubstituted oxindole skeleton has been reported;4 and the radical process involving alkylarylation,5 diarylation,6 arylnitration,7 arylphosphorylation,8 aryltrifluoromethylation,9 and azidoarylation10 of N-aryl acrylamides have since been disclosed by several groups, allowing the effective formation of the oxindole framework.
Cyanomethylation oxindoles are of significant interest because cyanomethylation reaction is considered to be a privileged reaction which resulted products can be utilized as key intermediates in drug synthesis.11 Some successful examples synthesizing cyanomethylated oxindoles have been reported in recent years.12 For example, in 2011, Liu discovered a novel Pd-catalyzed oxidative method to afford nitrile-bearing indolinones, which involves α-C–H activation of both aniline and acetonitrile by the aid of stoichiometric PhI(OCOtBu)2 and AgF.12a Subsequently, You and Zhu demonstrated Cu and Fe-catalysed 1,2-cyanoalkylarylation of N-aryl acrylamides for the construction of cyanomethylation of oxindoles using acetonitrile as cyanomethyl source, respectively.12b,12e Sheng also developed cyanomethylation of activated alkenes through a radical pathway using AIBN as the radical initiator.12g Nevertheless, stoichiometric amount of transition metals or promoter mediates are required in aforementioned examples. A milder and more efficient method for the synthesis of functionalized oxindoles is still highly desirable.
Nowadays, the visible-light photoredox catalysis strategy has been identified as a uniquely powerful and straightforward tool for synthetic transformations in organic chemistry, owing to its high efficiency and environmentally friendly mild reaction conditions.13 Several groups have been synthesized the oxindole derivatives by the means of UV light or visible-light photoredox catalysis.14 However, the visible-light photoredox catalysis approaches for their preparation of cyanomethylated oxindoles are extremely limited. As far as we know, only one example involving a visible-light catalyzed cyanomethylated of oxindoles has been reported by the Li group,12c in which moderate yields and equivalent of 4-MeOC6H4N2BF4 reagent was used as promoter. As part of our ongoing interest in visible light photoredox catalytic reactions,15 we present a novel visible-light induced radical addition/cyclization cascade cyanomethylation of N-aryl acrylamides for the synthesis of valuable cyanomethyl-containing oxindoles using bromoacetonitrile as cyanomethyl radical source (Scheme 1).
 |
| Scheme 1 Visible-light induced tandem cyanomethylation and cyclization of N-aryl acrylamides. | |
Initially, we investigated this reaction using N-methyl-N-phenylmethacrylamide (1a) and bromoacetonitrile (2) as the starting materials with K2CO3 as the base and fac-Ir(ppy)3 (2 mol%) as the catalyst. To our delight, the transformation proceeded smoothly after 24 h of irradiation with a 23 W household fluorescent lamp (CFL) in CH3CN at room temperature, affording the desired product 3a in 76% yield (Table 1, entry 1). When this reaction was performed in the absence of base, only 15% yield of 3a was obtained, and most of the N-methyl-N-phenylmethacrylamide 1a was recovered (Table 1, entry 2). Encouraged by this result, the reaction conditions of this cascade were further optimized. Firstly, we screened the catalysts and found that other photoredox catalysts, such as [Ir(dtbbpy)(ppy)2][PF6], Ru(bpy)3Cl2·6H2O, and Eosin Y, resulted in low reaction efficiency (Table 1, entries 3–5). Then we screened different bases (Table 1, entries 6–12). It turned out that Na2CO3 was the best base among the inorganic and organic bases tested, giving the product with 93% yield. A survey of commonly used solvents, such as DMF, DMSO, CHCl3, MeOH, and THF, were tested (Table 1, entries 13–17). However, reaction in none of the above solvents afforded higher yield than that in CH3CN. The highest yield was achieved when 2 equiv. of bromoacetonitrile was used (Table 1, entry 19). Increased to 4 equiv. or reduced to 1.5 equiv. of bromoacetonitrile led to a lower yield of 91% and 71%, respectively (Table 1, entries 18 and 20). Control experiments suggested that photocatalyst and irradiation are indispensable to this transformation (Table 1, entries 21 and 22).
Table 1 Optimization of reaction conditionsa
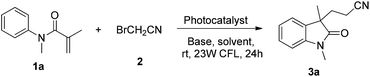
|
Entry |
Photocatalyst |
Base |
Solvent |
Yieldb (%) |
Reaction conditions: 1a (88 mg, 0.5 mmol), 2 (180 mg, 1.5 mmol, 3 eq.), catalyst (2 mol%), base (1 mmol, 2 eq.), solvent (5 mL), rt, 24 h, under N2 atmosphere. Determined by 1H NMR analysis with benzyl ether as an internal standard. The value in parentheses was isolated yield. 4 equiv. of 2 was used. 2 equiv. of 2 was used. 1.5 equiv. of 2 was used. In the dark. |
1 |
fac-Ir(ppy)3 |
K2CO3 |
CH3CN |
76 |
2 |
fac-Ir(ppy)3 |
None |
CH3CN |
15 |
3 |
[Ir(dtbbpy)(ppy)2][PF6] |
K2CO3 |
CH3CN |
12 |
4 |
Ru(bpy)3Cl2·6H2O |
K2CO3 |
CH3CN |
3 |
5 |
Eosin Y |
K2CO3 |
CH3CN |
5 |
6 |
fac-Ir(ppy)3 |
Li2CO3 |
CH3CN |
79 |
7 |
fac-Ir(ppy)3 |
Na2CO3 |
CH3CN |
93 |
8 |
fac-Ir(ppy)3 |
NaHCO3 |
CH3CN |
82 |
9 |
fac-Ir(ppy)3 |
Na2HPO4 |
CH3CN |
71 |
10 |
fac-Ir(ppy)3 |
K3PO4 |
CH3CN |
41 |
11 |
fac-Ir(ppy)3 |
KOAc |
CH3CN |
46 |
12 |
fac-Ir(ppy)3 |
NEt3 |
CH3CN |
29 |
13 |
fac-Ir(ppy)3 |
Na2CO3 |
DMF |
86 |
14 |
fac-Ir(ppy)3 |
Na2CO3 |
DMSO |
69 |
15 |
fac-Ir(ppy)3 |
Na2CO3 |
CHCl3 |
87 |
16 |
fac-Ir(ppy)3 |
Na2CO3 |
MeOH |
22 |
17 |
fac-Ir(ppy)3 |
Na2CO3 |
THF |
85 |
18d |
fac-Ir(ppy)3 |
Na2CO3 |
CH3CN |
91 |
19e |
fac-Ir(ppy)3 |
Na2CO3 |
CH3CN |
95(93)c |
20f |
fac-Ir(ppy)3 |
Na2CO3 |
CH3CN |
71 |
21 |
None |
Na2CO3 |
CH3CN |
0 |
22g |
fac-Ir(ppy)3 |
Na2CO3 |
CH3CN |
0 |
With the optimized reaction conditions in hand, we evaluated the scope of acrylamides with 2 (Table 2). Initially, the examination of different N-protecting groups revealed that methyl-protected (1a) was still the best choice, similar ethyl-protected (1b) and benzyl-protected (1c) substrate gave slightly reduced yields, whereas the reactions of N–H derivatives failed (1d). Gratifyingly, various functional groups were well tolerated, and both electron-donating group (e.g., Me, OMe, tBu) and electron-withdrawing group (e.g., CN, COOMe, COMe) substituents at the para position of the aniline moiety proceeded efficiently to afford the cyclized products 3e–n in moderate to good yields. Notably, halogen functional groups such as F, Cl, and Br were well-tolerated leading to the corresponding halogen-substituted cyanomethylation of oxindoles in good yields (76–90%, 3i–k, Table 2), which offered the potential for further synthetic elaboration. For the N-aryl acrylamides containing ortho-position substituent groups exhibited a particularly distinct steric hindrance effect, and lower yields were observed as a result (Table 2, 3o–p). N-Aryl acrylamides bearing a meta-substituent underwent cyanomethylation smoothly to give a mixture product of isomers in 95% yield with poor regioselectivity (3q
:
3q′ = 1.6
:
1). Moreover, 3,5-dimethyl N-aryl amides 3r also underwent the tandem reaction smoothly. In addition, naphthalene and tetrahydroquinoline derivative were also viable substrates to provide the corresponding oxindoles 3s and 3t with the same yield of 84% (Table 2, 3s–3t).
Table 2 Scope of acrylamidesab

|
Reaction conditions: 1 (0.5 mmol), BrCH2CN (120 mg, 2 equiv.), base (2 equiv.), catalyst (2 mol%), CH3CN (5 mL), irradiation with a 23 W household light bulb, rt, 24 h. Yields of isolated products. |
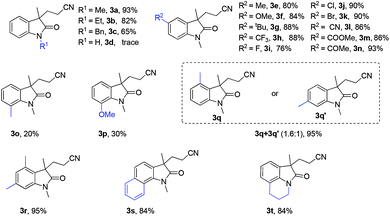 |
To gain additional mechanistic insights, 3 equiv. of TEMPO relative to 1a was added to the reaction system, no desired product 3a was observed and starting material was recovered, indicating that a radical process is probably involved in this reaction. On the basis of above results and previous literature reports,11 a plausible mechanism was proposed (Scheme 2).
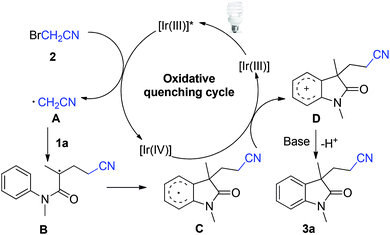 |
| Scheme 2 Proposed plausible mechanism. | |
Initially, iridium catalyst was excited to generate the excited species fac-[Ir(ppy)3]* under visible light irradiation, which then undergoes single electron transfer (SET) process with bromoacetonitrile 2 to generate cyanomethyl radical A and IrIV metal complex. Subsequently, the addition of cyanomethyl radical A to activated alkene 1a afforded alkyl radical B, followed by intramolecular cyclization with aryl ring lead to intermediate C, which was further oxidized through SET process to give key carbocation D and regenerated the photocatalyst. Finally, deprotonation of D in the presence of a base gave the desired product 3a.
Conclusions
In summary, we have disclosed an operationally convenient visible-light photocatalytic tandem cyanomethylation of N-aryl acrylamides using available bromoacetonitrile as starting material. The protocol presents a mild and efficient to furnish a variety of functionalized oxindoles. Both electron donating and electron withdrawing groups on the N-aryl acrylamides are tolerated in the reaction, and the corresponding products were obtained in moderate to good yields.
Conflicts of interest
There are no conflicts to declare.
Acknowledgements
The authors acknowledge the financial support provided by the National Natural Science Foundation of China (No. 21232003). We thank the Instrumental Analysis Center of Shanghai Jiao Tong University for microanalysis.
Notes and references
- For selected reviews, see:
(a) B. S. Jensen, CNS Drug Rev., 2002, 8, 353–360 CrossRef CAS PubMed;
(b) C. Marti and E. M. Carreira, Eur. J. Org. Chem., 2003, 2003, 2209–2219 CrossRef;
(c) C. V. Galliford and K. A. Scheidt, Angew. Chem., Int. Ed., 2007, 46, 8748–8758 CrossRef CAS PubMed;
(d) B. M. Trost and M. K. Brennan, Synthesis, 2009, 2009, 3003–3025 CrossRef;
(e) G. S. Singh and Z. Y. Desta, Chem. Rev., 2012, 112, 6104–6155 CrossRef CAS PubMed.
- For selected reviews, see:
(a) F. Zhou, Y.-L. Liu and J. Zhou, Adv. Synth. Catal., 2010, 352, 1381–1407 CrossRef CAS;
(b) J. E. M. N. Klein and R. J. K. Taylor, Eur. J. Org. Chem., 2011, 2011, 6821–6841 CrossRef CAS;
(c) R. Dalpozzo, G. Bartoli and G. Bencivenni, Chem. Soc. Rev., 2012, 41, 7247–7290 RSC.
- For selected reviews, see:
(a) G. Li, S. R. S. S. Kotti and C. Timmons, Eur. J. Org. Chem., 2007, 2007, 2745–2758 CrossRef;
(b) S. R. Chemler, Org. Biomol. Chem., 2009, 7, 3009–3019 RSC;
(c) R. M. Romero, T. H. Woste and K. Muniz, Chem.–Asian J., 2014, 9, 972–983 CrossRef CAS PubMed;
(d) T. Besset, T. Poisson and X. Pannecoucke, Eur. J. Org. Chem., 2015, 2015, 2765–2789 CrossRef CAS;
(e) G. Y. Yin, X. Mu and G. S. Liu, Acc. Chem. Res., 2016, 49, 2413–2423 CrossRef CAS PubMed.
- For selected reviews, see:
(a) J.-R. Chen, X.-Y. Yu and W.-J. Xiao, Synthesis, 2015, 47, 604–629 CrossRef CAS;
(b) R.-J. Song, Y. Liu, Y.-X. Xie and J.-H. Li, Synthesis, 2015, 47, 1195–1209 CrossRef CAS.
- For examples of alkylarylation, see:
(a) T. Wu, H. Zhang and G. Liu, Tetrahedron, 2012, 68, 5229–5233 CrossRef CAS;
(b) J.-H. Li, R.-J. Song, J.-H. Fan, M.-B. Zhou, Y. Liu, W.-T. Wei and X.-H. Ouyang, Synlett, 2014, 25, 657–660 CrossRef;
(c) Z. Li, Y. Zhang, L. Zhang and Z. Q. Liu, Org. Lett., 2014, 16, 382–385 CrossRef CAS PubMed;
(d) Z. Xu, C. Yan and Z. Q. Liu, Org. Lett., 2014, 16, 5670–5673 CrossRef CAS PubMed.
- For examples of diarylation, see:
(a) L. Shi, Y. Wang, H. Yang and H. Fu, Org. Biomol. Chem., 2014, 12, 4070–4073 RSC;
(b) S. Tang, D. Zhou and Y.-C. Wang, Eur. J. Org. Chem., 2014, 2014, 3656–3661 CrossRef CAS;
(c) B. Zhou, W. Hou, Y. Yang, H. Feng and Y. Li, Org. Lett., 2014, 16, 1322–1325 CrossRef CAS PubMed;
(d) Z. Ni, S. Wang, X. Huang, J. Wang and Y. Pan, Tetrahedron Lett., 2015, 56, 2512–2516 CrossRef CAS.
- For examples of arylnitration, see:
(a) Y. M. Li, X. H. Wei, X. A. Li and S. D. Yang, Chem. Commun., 2013, 49, 11701–11703 RSC;
(b) Y.-M. Li, Y. Shen, K.-J. Chang and S.-D. Yang, Tetrahedron Lett., 2014, 55, 2119–2122 CrossRef CAS;
(c) T. Shen, Y. Yuan and N. Jiao, Chem. Commun., 2014, 50, 554–556 RSC;
(d) S.-D. Yang, X.-H. Wei and Q.-X. Wu, Synlett, 2015, 26, 1417–1421 CrossRef.
- For examples of arylphosphorylation, see:
(a) Y. M. Li, M. Sun, H. L. Wang, Q. P. Tian and S. D. Yang, Angew. Chem., Int. Ed., 2013, 52, 3972–3976 CrossRef CAS PubMed;
(b) Y.-M. Li, Y. Shen, K.-J. Chang and S.-D. Yang, Tetrahedron, 2014, 70, 1991–1996 CrossRef CAS.
- For examples of aryltrifluoromethylation, see:
(a) H. Egami, R. Shimizu and M. Sodeoka, J. Fluorine Chem., 2013, 152, 51–55 CrossRef CAS;
(b) F.-X. Chen, Y.-F. Wang, J. Qiu and D. Kong, Synlett, 2014, 25, 1731–1734 CrossRef;
(c) W. Fu, F. Xu, Y. Fu, C. Xu, S. Li and D. Zou, Eur. J. Org. Chem., 2014, 2014, 709–712 CrossRef CAS;
(d) J. Liu, S. Zhuang, Q. Gui, X. Chen, Z. Yang and Z. Tan, Eur. J. Org. Chem., 2014, 2014, 3196–3202 CrossRef CAS;
(e) L. Shi, X. Yang, Y. Wang, H. Yang and H. Fu, Adv. Synth. Catal., 2014, 356, 1021–1028 CrossRef CAS;
(f) W. Wei, J. Wen, D. Yang, X. Liu, M. Guo, R. Dong and H. Wang, J. Org. Chem., 2014, 79, 4225–4230 CrossRef CAS PubMed;
(g) L. Zhang, Z. Li and Z. Q. Liu, Org. Lett., 2014, 16, 3688–3691 CrossRef CAS PubMed.
- For examples of azidoarylation, see:
(a) K. Matcha, R. Narayan and A. P. Antonchick, Angew. Chem., Int. Ed., 2013, 52, 7985–7989 CrossRef CAS PubMed;
(b) X.-H. Wei, Y.-M. Li, A.-X. Zhou, T.-T. Yang and S.-D. Yang, Org. Lett., 2013, 15, 4158–4161 CrossRef CAS PubMed;
(c) Y. Yuan, T. Shen, K. Wang and N. Jiao, Chem.–Asian J., 2013, 8, 2932–2935 CrossRef CAS PubMed;
(d) J. Qiu and R. Zhang, Org. Biomol. Chem., 2014, 12, 4329–4334 RSC.
-
(a) H. Yi, X. Zhang, C. Qin, Z. Liao, J. Liu and A. Lei, Adv. Synth. Catal., 2014, 356, 2873–2877 CrossRef CAS;
(b) E. R. Welin, A. A. Warkentin, J. C. Conrad and D. W. C. MacMillan, Angew. Chem., Int. Ed., 2015, 54, 9668–9672 CrossRef CAS PubMed;
(c) Q. Chang, Z. Liu, P. Liu, L. Yu and P. Sun, J. Org. Chem., 2017, 82, 5391–5397 CrossRef CAS PubMed;
(d) Y. Yu, Z. Cai, W. Yuan, P. Liu and P. Sun, J. Org. Chem., 2017, 82, 8148–8156 CrossRef CAS PubMed;
(e) Y. Kumagai, T. Naoe, K. Nishikawa, K. Osaka, T. Morita and Y. Yoshimi, Aust. J. Chem., 2015, 68, 1668–1671 CrossRef CAS.
-
(a) T. Wu, X. Mu and G. Liu, Angew. Chem., Int. Ed., 2011, 50, 12578–12581 CrossRef CAS PubMed;
(b) J. Li, Z. Wang, N. Wu, G. Gao and J. You, Chem. Commun., 2014, 50, 15049–15051 RSC;
(c) J.-L. Zhang, Y. Liu, R.-J. Song, G.-F. Jiang and J.-H. Li, Synlett, 2014, 25, 1031–1035 CrossRef CAS;
(d) X. Li, J. Xu, Y. Gao, H. Fang, G. Tang and Y. Zhao, J. Org. Chem., 2015, 80, 2621–2626 CrossRef CAS PubMed;
(e) C. Pan, H. Zhang and C. Zhu, Org. Biomol. Chem., 2015, 13, 361–364 RSC;
(f) S. Tang, S.-H. Li, Z.-H. Li, D. Zhou and R.-L. Sheng, Tetrahedron Lett., 2015, 56, 1423–1426 CrossRef CAS;
(g) S. Tang, D. Zhou, Z.-H. Li, M.-J. Fu, L. Jie, R.-L. Sheng and S.-H. Li, Synthesis, 2015, 47, 1567–1580 CrossRef CAS;
(h) D. Zhou, Z.-H. Li, J. Li, S.-H. Li, M.-W. Wang, X.-L. Luo, G.-L. Ding, R.-L. Sheng, M.-J. Fu and S. Tang, Eur. J. Org. Chem., 2015, 2015, 1606–1612 CrossRef CAS;
(i) Z. Ni, X. Huang, J. Wang and Y. Pan, RSC Adv., 2016, 6, 522–526 RSC.
- For selected reviews, see:
(a) J. M. R. Narayanam and C. R. J. Stephenson, Chem. Soc. Rev., 2011, 40, 102–113 RSC;
(b) J. Xuan and W.-J. Xiao, Angew. Chem., Int. Ed., 2012, 51, 6828–6838 CrossRef CAS PubMed;
(c) C. K. Prier, D. A. Rankic and D. W. C. MacMillan, Chem. Rev., 2013, 113, 5322–5363 CrossRef CAS PubMed;
(d) N. A. Romero and D. A. Nicewicz, Chem. Rev., 2016, 116, 10075–10166 CrossRef CAS PubMed;
(e) K. L. Skubi, T. R. Blum and T. P. Yoon, Chem. Rev., 2016, 116, 10035–10074 CrossRef CAS PubMed;
(f) J. Xuan, Z.-G. Zhang and W.-J. Xiao, Angew. Chem., Int. Ed., 2015, 54, 15632–15641 CrossRef CAS PubMed;
(g) J.-R. Chen, D.-M. Yan, Q. Wei and W.-J. Xiao, ChemPhotoChem, 2017, 1, 148–158 CrossRef CAS.
-
(a) X. Ju, Y. Liang, P. Jia, W. Li and W. Yu, Org. Biomol. Chem., 2012, 10, 498–501 RSC;
(b) W. Fu, F. Xu, Y. Fu, M. Zhu, J. Yu, C. Xu and D. Zou, J. Org. Chem., 2013, 78, 12202–12206 CrossRef CAS PubMed;
(c) J. Xie, P. Xu, H. Li, Q. Xue, H. Jin, Y. Cheng and C. Zhu, Chem. Commun., 2013, 49, 5672–5674 RSC;
(d) P. Xu, J. Xie, Q. Xue, C. Pan, Y. Cheng and C. Zhu, Chemistry, 2013, 19, 14039–14042 CrossRef CAS PubMed;
(e) W. Fu, M. Zhu, G. Zou, C. Xu and Z. Wang, Synlett, 2014, 25, 2513–2517 CrossRef CAS;
(f) W. Fu, M. Zhu, G. Zou, C. Xu and Z. Wang, Asian J. Org. Chem., 2014, 3, 1273–1276 CrossRef CAS;
(g) Y. Liu, J.-L. Zhang, R.-J. Song and J.-H. Li, Org. Chem. Front., 2014, 1, 1289–1294 RSC;
(h) X. J. Tang, C. S. Thomoson and W. R. Dolbier Jr, Org. Lett., 2014, 16, 4594–4597 CrossRef CAS PubMed;
(i) G. Bergonzini, C. Cassani and C. J. Wallentin, Angew. Chem., Int. Ed., 2015, 54, 14066–14069 CrossRef CAS PubMed;
(j) C. Liu, W. Zhao, Y. Huang, H. Wang and B. Zhang, Tetrahedron, 2015, 71, 4344–4351 CrossRef CAS;
(k) D. Xia, T. Miao, P. Li and L. Wang, Chem.–Asian J., 2015, 10, 1919–1925 CrossRef CAS PubMed;
(l) Y. An, Y. Li and J. Wu, Org. Chem. Front., 2016, 3, 570–573 RSC;
(m) W. Ji, H. Tan, M. Wang, P. Li and L. Wang, Chem. Commun., 2016, 52, 1462–1465 RSC;
(n) C. X. Li, D. S. Tu, R. Yao, H. Yan and C. S. Lu, Org. Lett., 2016, 18, 4928–4931 CrossRef CAS PubMed;
(o) G. Yin, M. Zhu, G. Yang, X. Wang and W. Fu, J. Fluorine Chem., 2016, 191, 63–69 CrossRef CAS;
(p) K. Zhou, H. Xia and J. Wu, Org. Chem. Front., 2016, 3, 865–869 RSC;
(q) Z. Gonda, F. Béke, O. Tischler, M. Petró, Z. Novák and B. L. Tóth, Eur. J. Org. Chem., 2017, 2017, 2112–2117 CrossRef CAS;
(r) T. Liu, D. Zheng and J. Wu, Org. Chem. Front., 2017, 4, 1079–1083 RSC;
(s) D. Xia, Y. Li, T. Miao, P. Li and L. Wang, Green Chem., 2017, 19, 1732–1739 RSC;
(t) Z. Xie, P. Li, Y. Hu, N. Xu and L. Wang, Org. Biomol. Chem., 2017, 15, 4205–4211 RSC;
(u) S.-M. Xu, J.-Q. Chen, D. Liu, Y. Bao, Y.-M. Liang and P.-F. Xu, Org. Chem. Front., 2017, 4, 1331–1335 RSC.
-
(a) B. Hu, H. X. Chen, Y. Liu, W. H. Dong, K. Ren, X. M. Xie, H. Xu and Z. G. Zhang, Chem. Commun., 2014, 50, 13547–13550 RSC;
(b) W. H. Dong, Y. Liu, B. Hu, K. Ren, Y. Y. Li, X. M. Xie, Y. X. Jiang and Z. G. Zhang, Chem. Commun., 2015, 51, 4587–4590 RSC;
(c) B. Hu, Y. Y. Li, W. H. Dong, K. Ren, X. M. Xie, J. Wan and Z. G. Zhang, Chem. Commun., 2016, 52, 3709–3712 RSC;
(d) Y. Y. Li, B. Hui, W. H. Dong, X. M. Xie, J. Wan and Z. G. Zhang, J. Org. Chem., 2016, 81, 7036–7041 CrossRef CAS PubMed.
Footnote |
† Electronic supplementary information (ESI) available. See DOI: 10.1039/c7ra10090f |
|
This journal is © The Royal Society of Chemistry 2017 |