DOI:
10.1039/C7RA09330F
(Paper)
RSC Adv., 2017,
7, 50264-50268
Simple method for simultaneously achieving red and green up-conversion luminescence†
Received
23rd August 2017
, Accepted 19th October 2017
First published on 27th October 2017
Abstract
The simultaneous emission of red and green light with high brightness and color purity was obtained from Er3+-doped NaYbF4-based up-conversion nanoparticles excited by 980 and 1550 nm excitation. The 2F5/2 level of Yb3+ showed high absorption efficiency at 980 nm. The 4I13/2 level of Er3+, an excellent UC intermediate with high energy and a long lifetime (milliseconds), absorbed more energy in the cross section (6.0 × 10−20 cm2) than did the Yb3+ 2F5/2 level (1.2 × 10−20 cm2) and was efficiently and directly pumped by light with a wavelength of ∼1500 nm. In contrast to particles resulting from other methods using complex coating for achieving multi-color emission in a single sample, the as-prepared luminescent NaYbF4:25% Er3+ up-conversion nanoparticles were designed to be single-layered and spherical and showed excellent dispersibility and uniform sizes. Nanoparticles prepared by this method exhibit a great advantage based on the simple preparation process and small particle size. On this basis, we expect to easily achieve the emission of three primary colors by these reasonably designed core–shell particles.
1. Introduction
Multi-color up-conversion luminescence (UCL) materials have major application prospects in biological imaging, multiple detection and treatment of disease, anti-counterfeiting and multi-color coding, and display areas.1–4 The tunable range of the up-conversion (UC) emission color, however, is seriously limited due to the finite excitation wavelength, type of activator, and inappropriate emission levels, which also hinder the wide application of UCL materials.5,6 Current methods for achieving multi-color emission can be divided into three types. (1) The first type of method involves achieving emissions from multiple samples by exciting them with a single wavelength.7,8 Here, the emission color can be finely adjusted by changing the host,9 doping concentrations of rare earth ions,10,11 particle size12,13 and crystal structure,14,15 or co-doping with other ions.16,17 Furthermore, the UCL color can be tuned using different activator combinations and core–shell structures excited with the single wavelength.18,19 (2) The second type of method involves achieving emissions from multiple samples by exciting them with multiple wavelengths.20 These two methods usually face chromatic aberration problems resulting from different types of particles having inconsistent sedimentation rates and being unevenly mixed. (3) The third type of method involves achieving multi-color emission from a single sample under multi-wavelength excitation, and this method presents excellent application characteristics. Wen et al. prepared NaYF4 up-conversion nanoparticles (UCNPs) with a five-layer core–shell structure to obtain blue-green two-color emission under excitation with light of wavelengths of 980 and 808 nm.21 Deng et al.22 designed an unsteady UC full-color luminescence in NaYF4 UCNPs with a five-layer core–shell structure by adjusting the pulse width. These UCNPs realize multi-color emission but require a relatively complex preparation process and large particle size. Consequently, there is an urgent need to obtain a simple method for realizing multi-color emission.
Green and red double color UCL has been realized in micron-sized single Y2O2S:Yb3+, Er3+ UC materials under 980 and 1550 nm dual wavelength excitation.23 Yb3+ and Er3+ ions form two sensitization systems excited at 980 and 1550 nm, respectively, and exhibit different UCL characteristics. In the current work, NaYbF4:25% Er3+ UCNPs were shown to simultaneously emit red and green light when excited with light of wavelengths of 980 and 1550 nm.
2. Experimental
2.1 Materials
All materials purchased were used as described below without further purification. 1-Octadecene (OM; 90%), oleic acid (OA; 90%), Y(CH3COO)3·4H2O, Yb(CH3COO)3, and Er(CH3COO)3 were purchased from Alfa Aesar. Ammonium fluoride (NH4F), sodium hydroxide (NaOH), methanol, cyclohexane, and ethanol were purchased from Tianjinzhiyuan Chemical Reagent Co and used as received. For each experiment, Re(CH3COO)3 was dissolved in deionized water, and NaOH and NH4F were dissolved in methanol solution in advance to reduce the experimental error.
2.2 Synthesis of NaYbF4:xEr3+ (x = 10, 25, 50, 90 mol%)
A volume of 3 mL of oleic acid and a volume of 7 mL of octadecene were added, respectively, to two vials by using a two-way valve, and a volume of 2 mL (0.4 mmol) of Re(CH3COO)3 (0.2 M) was added in proportion (Yb = 0.36, 0.3, 0.2, 0.04 mmol, as Er = 0.04, 0.1, 0.2, 0.36 mmol, respectively). A thermometer able to measure temperatures between 0 and 360 °C was inserted into each solution to precisely measure the temperature. The samples became pale yellow after being heating to 150 °C and kept at this temperature for 45 min, and a rare earth oleate precursor solution was obtained as the sample was cooled to room temperature. Methanol solutions of NaOH (1 mL, 1 M) and NH4F (4 mL, 0.4 M) were added to the respective flasks, and kept at 50 °C for 40 min. The temperature was then increased to 100 °C in a vacuum environment in order to remove the methanol from the mixtures. Each solution was quickly heated (15 min) to 290 °C under an argon environment and then, when bubbles were no longer produced, kept warm at 290 °C for 1.5 h, and desired product was obtained as the solution was cooled to room temperature. A volume of 8 mL of an ethanol solution was added to each solution, and the resulting mixture was centrifuged at 9000 rad per min for 6 min. The resulting product in each case was dissolved in 4 mL of cyclohexane and 8 mL of ethanol, and the resulting solution was centrifuged at 9000 rad per min for 6 min. The previous step was then repeated with 4 mL of ethanol and 4 mL of methanol instead of 8 mL of ethanol. The resulting sample in each case was dissolved in 5 mL of cyclohexane and sealed and stored in a glass vial at 4 °C.
2.3 Characterizations
The size and morphology of each sample were determined by performing JEM-2100F high-resolution transmission electron microscopy (HRTEM). The UCL spectra were acquired by using an Edinburgh FS5 fluorescence spectrometer, and power-tunable 980 nm-wavelength (maximum power: 800 mW) and 1550 nm-wavelength (maximum power: 800 mW) laser diodes (LDs) were used as excitation sources.
3. Results and discussion
4I11/2 of Er3+ was pumped directly by light with a wavelength of ∼980 nm due to the relatively large energy absorption section (1.7 × 10−21 cm2) and millisecond-range lifetime of the 4I11/2 level.23 In addition, the 4I13/2 level of Er3+ was found in the current work to be an excellent UC intermediate energy level with a long lifetime, and with an energy absorption cross section (6.0 × 10−20 cm2) much larger than that of the Yb3+ 2F5/2 level (1.2 × 10−20 cm2) and that was efficiently pumped by light with a wavelength of ∼1500 nm. Therefore, a high UCL efficiency was achieved using Er3+ ions as the activator with 980 and 1550 nm dual-wavelength excitation.24 An efficient single red UCL was easily obtained at 1500 nm-wavelength excitation relying on the non-radiative 4I9/2 → 4I11/2 relaxation, which is based on the relatively short lifetime (10 microseconds) of Er3+ ions and small energy gap between 4I9/2 and 4I11/2 levels (ΔE ≈ 1900 cm−1).25,26 Fig. 3a and b are the main green and red double-color UCL mechanism of Y2O2S:Yb3+, Er3+ UC materials under 980 and 1550 nm dual-wavelength excitation. However, the opposite is the case for the β-NaYF4 host, as β-NaYF4:Er3+ (ref. 27 and 28) and LiYF4:Er3+ (ref. 29) show yellow-green UCL when excited at a wavelength of 1500 nm. This condition remains unchanged even upon adding Yb3+ ions (Fig. S1f†), and high-color-purity emission of red light is difficult to obtain from the β-NaYF4 host. Therefore, in this work, spherical NaYbF4:x% Er3+ UCNPs (Fig. 1), with an average particle size of 18.34 nm, were prepared through co-precipitation. The distance between adjacent lattice planes of this product was measured using the HRTEM images to be about 0.51 nm, which confirmed the product to be β-phase NaYbF4 (Fig. 1b).
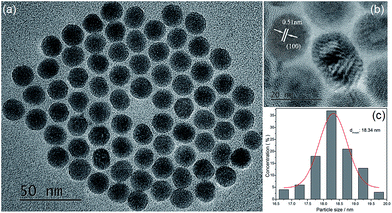 |
| Fig. 1 (a), (b) HRTEM images and (c) corresponding particle size histograms of NaYbF4:25% Er3+. | |
NaYbF4:x% Er3+ showed different luminescence properties with different Er3+ doping concentrations and presented distinct emission colors under excitation with wavelengths of 980 nm and 1550 nm (Fig. S1†). Under excitation at 980 nm, NaYbF4:10% Er3+ showed green UCL with a red-to-green emission ratio (Ir/Ig) of 0.59 (Fig. S1a†) and NaYbF4:25% Er3+ showed red UCL, specifically at 654 nm (Ir/Ig = 3.29, Fig. 2), whereas NaYbF4:50% Er3+ exhibited decreased red UCL color purity (Ir/Ig = 2.19, Fig. S1†) and exhibited luminescence quenching. However, Wei et al.30 reported that NaYbF4:50 mol% Er3+ exhibits the optimal red UCL performance when excited with light at a wavelength of 980 nm, this condition is mainly resulted from the large particle size (∼100 nm) and crystallinity (Fig. S2†). NaYbF4:xEr3+ excited at 1550 nm showed a constant green UCL (Fig. S1b†) and exhibited the optimal emission characteristics when the doping concentration of Er3+ was 25 mol% (Ir/Ig = 0.45, Fig. 2). Therefore, emission of both red and green light with high brightness and color purity was achieved using NaYbF4:25% Er3+ subjected to dual-wavelength excitation, at 980 and 1500 nm; the corresponding CIE chromaticity coordinates were determined to be (0.62, 0.31) and (0.29, 0.64), respectively. The double-color UCL samples prepared by this method were each shown to have a simple structure and small particle size, and the preparation process was simple and easily controlled. Multi-color emission was achieved by adjusting the relative intensities of the two lasers (Fig. 2b).
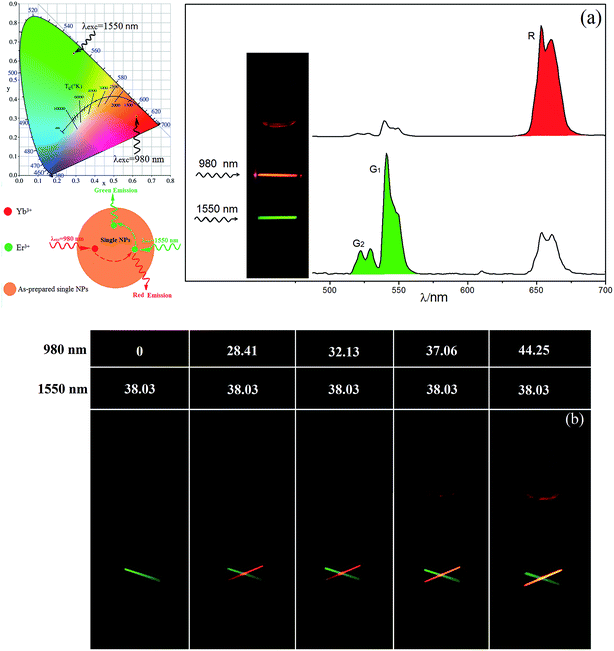 |
| Fig. 2 (a) CIE chromaticity coordinates, UCL spectra, and digital camera images of NaYbF4:25% Er3+ under 980 and 1500 nm wavelength excitation. (b) Multi-color emission images of NaYbF4:25% Er3+ under excitation with wavelengths of 980 nm (excitation power density = 0, 28.41, 32.13, 37.06, 44.52 mW mm−2) and 1550 nm (excitation power density = 38.03 mW mm−2) (Canon EOS 5D Mark III, Tv = 1 : 1250, Len: EF24-70 mm f/2.8 L II USM, Av = 2.8, ISO = 12800). | |
The red and green UCL displayed by NaYbF4:25% Er3+ under 980 and 1550 nm-wavelength excitation was attributed to different interaction mechanisms between Yb3+ and Er3+ excited at these two excitation wavelengths. Wei et al.30 attributed the enhancement of red UCL in highly doped Er3+ samples to the cross relaxation (CR, 4F7/2 + 4I11/2 → 4F9/2, +4F9/2) process between Er3+ ions. The CR process was enhanced as the Er3+ concentration was increased, and with increasing Ir/Ig (Fig. S3†). However, the Ir/Ig of Er3+-doped NaYbF4:xEr3+ samples failed to increase as the Er3+ concentration was increased, indicating that the enhancement of red UCL was also related to Yb3+ co-doping.31 Therefore, the enhancement of red UCL in NaYbF4:25% Er3+ excited at 980 nm was due to the combined effect of the CR process and reverse energy transfer from the Er3+ 4G11/2 level to Yb3+ (Fig. 3c). The green emission displayed by NaYbF4:25% Er3+ excited with light of a wavelength of 1550 nm was mainly due to the radiation relaxation 4I9/2 → 4I11/2 occurring in other host being less likely to take place in NaYbF4 because of the low phonon energy, thus making the energy transfer process Er3+ → Yb3+ → Er3+ difficult to transpire.32 Moreover, the lack of occurrence of the above CR process and the occurrence of weak red emission (Fig. 3d) were due to the difficulty in the 4F7/2 level being pumped by light with a wavelength of 1550 nm (Fig. 4).
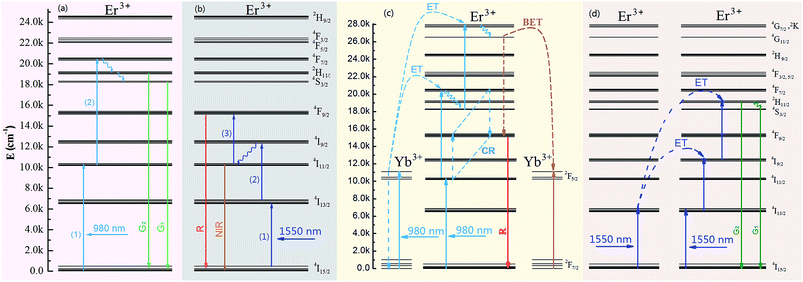 |
| Fig. 3 (a, b) Double-color UCL mechanism of Er3+ under (a) 980 nm-wavelength and (b) 1500 nm-wavelength excitation. (c, d) UCL mechanism of NaYbF4:25% Er3+ under (c) 980 nm-wavelength and (d) 1500 nm-wavelength excitation. | |
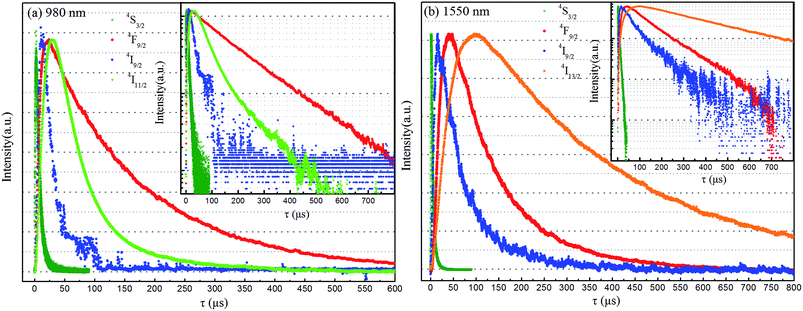 |
| Fig. 4 Decay curves of the Er3+ 4S3/2 (550 nm), 4F9/2 (654 nm), 4I9/2 (800 nm), 4I11/2 (1000 nm), 4I13/2 (1535 nm) levels in an NaYbF4:Er3+ sample under (a) 980 nm-wavelength and (b) 1550 nm-wavelength pulse LD excitation. | |
4. Conclusions
Single-layer spherical NaYbF4:25% Er3+ UCNPs, with an average particle size of 18 nm, showed double-color emission, of red and green light, under dual-wavelength excitation, of 980 nm and 1550 nm wavelengths. In contrast to other methods for achieving multi-color emission from a single sample with complex coating processes, the as-prepared Er3+-doped NaYbF4-based system is a single-layer structure. And compared with the existing research on multi-color luminescence, the method we have now described involves a simple preparation process, suggesting the potential of achieving mass production. And on this basis, the three primary colors may be expected to be easily achieved by a reasonable designed core–shell.
Conflicts of interest
There are no conflicts to declare.
Acknowledgements
The authors thank the Research Program of the National Natural Science Foundation of China (Grant No. 11504039 and 51502031), and the Application Foundation (Main subject) of the Ministry of Transport of PR China (No. 2015329225090). The Fundamental Research Funds for the Central Universities (Grant No. 3132016349 and 3132017061), Program for Liaoning Excellent Talents in University (LR2013020), and Foundation of Liaoning Educational Committee (L2013201, L2014212, L2014208) are also acknowledged for their financial support.
References
- F. Wang and X. G. Liu, Multicolor tuning of lanthanide-doped nanoparticles by single wavelength excitation, Acc. Chem. Res., 2014, 47, 1378–1385 CrossRef CAS PubMed.
- Y. H. Zhang, L. X. Zhang, R. R. Deng, J. Tian, Y. Zong, D. Y. Jin and X. G. Liu, Multicolor barcoding in a single upconversion crystal, J. Am. Chem. Soc., 2014, 136, 4893–4896 CrossRef CAS PubMed.
- B. Zhou, B. Y. Shi, D. Y. Jin and X. G. Liu, Controlling upconversion nanocrystals for emerging applications, Nat. Nanotechnol., 2015, 10, 924–936 CrossRef CAS PubMed.
- X. Li, R. Wang, F. Zhang and D. Zhao, Engineering homogeneous doping in single nanoparticle to enhance upconversion efficiency, Nano Lett., 2014, 14, 3634–3639 CrossRef CAS PubMed.
- D. J. Gargas, E. M. Chan, A. D. Ostrowski, S. Aloni, M. V. P. Altoe, E. S. Barnard, B. Sanii, J. J. Urban, D. J. Milliron, B. E. Cohen and P. J. Schuck, Engineering bright sub-10-nm upconverting nanocrystals for single-molecule imaging, Nat. Nanotechnol., 2014, 9, 300–305 CrossRef CAS PubMed.
- J. J. Zhou, G. X. Chen, E. Wu, G. Bi, B. T. Wu, Y. Teng, S. F. Zhou and J. R. Qiu, Ultrasensitive polarized up-conversion of Tm3+-Yb3+ doped β-NaYF4 single nanorod, Nano Lett., 2013, 13, 2241–2246 CrossRef CAS PubMed.
- O. Ehlert, R. Thomann, M. Darbandi and T. Nann, A four-color colloidal multiplexing nanoparticle system, ACS Nano, 2008, 2, 120–124 CrossRef CAS PubMed.
- M. L. You, M. Lin, S. R. Wang, X. M. Wang, G. Zhang, Y. Hong, Y. Q. Dong, G. R. Jin and F. Xu, Three-dimensional quick response code based on inkjet printing of upconversion fluorescent nanoparticles for drug anti-counterfeiting, Nanoscale, 2016, 8, 10096 RSC.
- J. Wang, F. Wang, C. Wang, Z. Liu and X. G. Liu, Single-band upconversion emission in lanthanide-doped KMnF3 nanocrystals, Angew. Chem., Int. Ed., 2011, 50(44), 10369–10372 CrossRef CAS PubMed.
- F. Wang and X. G. Liu, Upconversion multicolor fine-tuning: visible to near-infrared emission from lanthanide-doped NaYF4 nanoparticles, J. Am. Chem. Soc., 2008, 130, 5642–5643 CrossRef CAS PubMed.
- V. Mahalingam, F. Vetrone, R. Naccache, A. Speghini and J. A. Capobianco, Colloidal Tm3+/Yb3+-Doped LiYF4 nanocrystals: multiple luminescence spanning the UV to NIR regions via low-energy excitation, Adv. Mater., 2009, 21, 4025–4028 CrossRef CAS.
- H. X. Mai, Y. W. Zhang, L. D. Sun and C. H. Yan, Highly efficient multicolor up-conversion emissions and their mechanisms of monodisperse NaYF4:Yb,Er core and core/shell- structured nanocrystals, J. Phys. Chem. C, 2007, 111, 13721–13729 CAS.
- J. Zhao, Z. Lu, Y. Yin, C. McRae, J. A. Piper, J. M. Dawes, D. Jin and E. M. Goldys, Upconversion luminescence with tunable lifetime in NaYF4:Yb, Er nanocrystals: role of nanocrystal size, Nanoscale, 2013, 5, 944–952 RSC.
- W. B. Niu, S. L. Wu, S. F. Zhang, J. Li and L. A. Li, Multicolor output and shape controlled synthesis of lanthanide-ion doped fluorides upconversion nanoparticles, Dalton Trans., 2011, 40, 3305–3314 RSC.
- H. Dong, L. D. Sun, Y. F. Wang, J. Ke, R. Si, J. W. Xiao, G. M. Lyu, S. Shi and C. H. Yan, Efficient tailoring of upconversion selectivity by engineering local structure of lanthanides in NaxREF3+x nanocrystals, J. Am. Chem. Soc., 2015, 137, 6569–6576 CrossRef CAS PubMed.
- G. Chen, H. Liu, G. Somesfalean, H. Liang and Z. Zhang, Upconversion emission tuning from green to red in Yb3+/Ho3+- codoped NaYF4 nanocrystals by tridoping with Ce3+ ions, Nanotechnology, 2009, 20, 385704 CrossRef PubMed.
- F. Zhang, Q. Shi, Y. Zhang, Y. Shi, K. Ding, D. Zhao and G. D. Stucky, Fluorescence upconversion microbarcodes for multiplexed biological detection: nucleic acid encoding, Adv. Mater., 2011, 23, 3775–3779 CAS.
- E. M. Chan, G. Han, J. D. Goldberg, D. J. Gargas, A. D. Ostrowski, P. J. Schuck, B. E. Cohen and D. J. Milliron, Combinatorial discovery of lanthanide-doped nanocrystals with spectrally pure upconverted emission, Nano Lett., 2012, 12, 3839–3845 CrossRef CAS PubMed.
- F. Wang, R. Deng, J. Wang, Q. Wang, Y. Han, H. Zhu, X. Chen and X. Liu, Tuning upconversion through energy migration in core-shell nanoparticles, Nat. Mater., 2011, 10, 968–973 CrossRef CAS PubMed.
- E. Downing, L. Hesselink, J. Ralston and R. Macfarlane, A three-color, solid-sate, three- dimensional display, Science, 1996, 273, 1185–1189 CAS.
- H. L. Wen, H. Zhu, X. Chen, T. F. Hung, B. L. Wang, G. Y. Zhu, S. F. Yu and F. Wang, Upconverting near-infrared light through energy management in core-shell-shell nanoparticles, Angew. Chem., Int. Ed., 2013, 52, 13419–13423 CrossRef CAS PubMed.
- R. R. Deng, F. Qin, R. F. Chen, W. Huang, M. H. Hong and X. G. Liu, Temporal full-colour tuning through non-steady-state upconversion, Nat. Nanotechnol., 2015, 10, 237–242 CrossRef CAS PubMed.
- H. Wang, M. M. Xing, X. X. Luo, X. L. Zhou, Y. Fu, T. Jiang, Y. Peng, Y. B. Ma and X. L. Duan, Upconversion emission colour modulation of Y2O2S: Yb, Er under 1.55 μm and 980 nm excitation, J. Alloys Compd., 2014, 587, 344–348 CrossRef CAS.
- S. Fischer, B. Frohlich, K. W. Kramer and J. C. Goldschmidt, Relation between excitation power density and Er3+ doping yielding the highest absolute upconversion quantum yield, J. Phys. Chem. C, 2014, 118, 30106–30114 CAS.
- X. M. Yin, H. Wang, M. M. Xing, Y. Fu, Y. Tian, T. Jiang and X. X. Luo, High color purity red emission of Y2Ti2O7:Yb3+,Er3+ under 1550 and 980 nm excitation, J. Lumin., 2017, 182, 183–188 CrossRef CAS.
- H. Wang, T. Jiang, M. M. Xing, Y. Fu, Y. Peng and X. X. Luo, Up - conversion luminescence of Y2O3: Yb, Er under 1.55 μm excitation, Ceram. Int., 2015, 41, 259–263 CrossRef CAS.
- W. Shao, G. Y. Chen, J. Damasco, X. L. Wang, A. Kachynski, T. Y. Ohulchanskyy, C. H. Yang, H. Agren and P. N. Prasad, Enhanced upconversion emission in colloidal (NaYF4:Er3+)/NaYF4 core/shell nanoparticles excited at 1523 nm, Opt. Lett., 2014, 39, 1386–1389 CrossRef CAS PubMed.
- H. Wang, X. M. Yin, M. M. Xing, Y. Fu, Y. Tian, X. Feng, T. Jiang and X. X. Luo, Investigation on the thermal effects of NaYF4:Er under 1550 nm irradiation, Phys. Chem. Chem. Phys., 2017, 19, 8465–8470 RSC.
- G. Y. Chen, T. Y. Ohulchanskyy, A. Kachynski, H. Agren and P. N. Prasad, Intense visible and near-infrared upconversion photoluminescence in colloidal LiYF4:Er3+ nanocrystals under excitation at 1490 nm, ACS Nano, 2011, 5, 4981–4986 CrossRef CAS PubMed.
- W. Wei, Y. Zhang, R. Chen, J. L. Goggi, N. Ren, L. Huang, K. K. Bhakoo, H. Sun and T. T. Y. Tan, Cross relaxation induced pure red upconversion in activator- and sensitizer-rich lanthanide nanoparticles, Chem. Mater., 2014, 26, 5183–5186 CrossRef CAS.
- M. T. Berry and P. S. May, Disputed mechanism for NIR-to-Red upconversion luminescence in NaYF4:Yb3+,Er3+, J. Phys. Chem. A, 2015, 119, 9805–9811 CrossRef CAS PubMed.
- X. L. Shen, M. M. Xing, Y. Tian, Y. Fu, Y. Peng and X. X. Luo, Upconversion photoluminescence properties of SrY2O4:Er3+,Yb3+ under 1550 and 980 nm excitation, J. Rare Earths, 2016, 34, 458–463 CrossRef CAS.
Footnote |
† Electronic supplementary information (ESI) available. See DOI: 10.1039/c7ra09330f |
|
This journal is © The Royal Society of Chemistry 2017 |