DOI:
10.1039/C7RA09037D
(Comment)
RSC Adv., 2017,
7, 53686-53688
Reply to the ‘Comment’ on “Lewis acidic ionic liquids of crown ether complex cations: preparation and applications in organic reactions” by M. Swadźba-Kwaśny, RSC Adv., 2017, 7, DOI: 10.1039/c7ra05921c
Received
15th August 2017
, Accepted 8th November 2017
First published on 22nd November 2017
Introduction
We published recently in RSC Advances a paper,1 reporting a new group of Lewis acidic ionic liquids that were prepared by the combination of a crown ether (18-crown-6) and alkali metal chloride (NaCl or KCl) and applied in organic reactions as catalysts. This study was developed by following our previous report of crown ether complex cations ionic liquids (CECILs).2 The typical structures of new CECILs are described in Fig. 1 (left). The anion structures were assigned using anionic mass spectra and Raman spectroscopy (Fig. 1, right), indicating the symmetry stretching vibration of M–Clx cluster. The abovementioned assignment was doubted by Małgorzata Swadźba-Kwaśny in the comment.
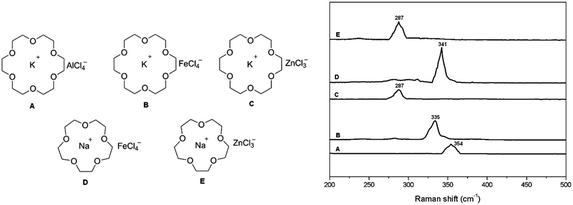 |
| Fig. 1 Left: structures of Lewis acidic ionic liquids; right: Raman spectra of M–Clx cluster for symmetry stretching vibration. | |
Discussion
People who work on the coordination chemistry know that the coordination structure of transition metal complexes varies according to their ligands, solvents, and also their counterions. The structure of anion cluster ZnClx also obeys this rule. As a matter of fact, the description shown in the Fig. 1 of our paper is a typically expressed formula for organic compounds. The real assignment for this cluster can be seen in the first paragraph of results and discussion: “On the basis of the principles of Raman spectroscopy, the Stokes line is attributed to the symmetric stretching vibration of metal chloride clusters. The Stokes line of the triangular structure of ZnCl3 that has a weak interaction with cation appeared at 287 cm−1 in both C and E ILs.”
Structures description in the literature
The tetrahedral structure of [ZnCl4]2− anion cluster generally appears in the crystal of double salts, such as K2[ZnCl4].3,4 The dimer structure of [V2Cl3(THF)6]2[Zn2Cl6] was also reported in literature.5 For the anion cluster of [ZnCl3]−, there are also some reported examples,6–9 in which, most of them require a ligand to stabilize their structure as a distorted tetrahedron. The oxygen of water and nitrogen of heterocycles can be used as stabilizer to form anion cluster of [ZnCl3·H2O]− and [ZnCl3·L]−. In these structures, the linkage between [ZnCl3]− and water or ligand is weaker than the bond of Zn–Cl. When the crown ether metal cation is used, the water can be considered as a rotator.8
Raman spectra experiments part 1
The Raman spectra of these clusters were widely investigated in numerous literature reports. Gzaiel et al. reported6 that the vibrational frequency appearing at 261 cm−1 is attributed to the anion cluster of [ZnCl4]2−, and that at 283 cm−1 can be assigned as the vibration of anion cluster of [ZnCl3·H2O]−. Their crystal structures are clearly shown in Fig. 2.
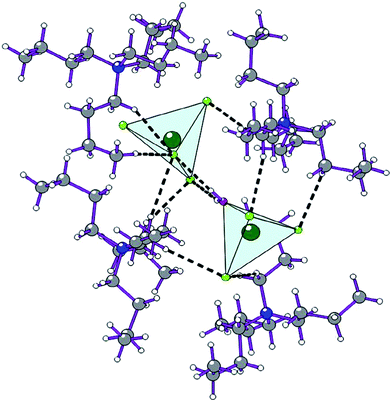 |
| Fig. 2 The anion clusters of [ZnCl4]2− and [ZnCl3·H2O]− in the single crystal.6 | |
Raman spectra experiments part 2
Symmetry and bond strength analysis
Considering the results of Raman frequencies for anion cluster of [ZnCl4]2− and [ZnCl3·H2O]−reported in literature 6 and depicted in Fig. 3, we reassigned these results using symmetry analysis in light of the fact that Raman activity vibration models are primarily designed on the basis of the symmetric vibration of the clusters. In the anion cluster of [ZnCl4]2−, which is assigned to Td point group, the bond strength of Zn–Cl is weaker than in the anion cluster of [ZnCl3·H2O]−, resulting in smaller wave numbers of symmetry vibration (261 vs. 283 cm−1). When the temperature changes, the unstable anion cluster of [ZnCl3·H2O]− could dimerize to [Zn2Cl6]2− that has a D2d point group. In addition, the dimer exhibits two symmetry vibrations at 312 and 195 cm−1 linked to A1 and B2 symmetry, which differs from the only one symmetry vibration model reflecting A1 symmetry in Td of [ZnCl4]2−, D3h of [ZnCl3]− and C3v of [ZnCl3·H2O]− (Fig. 4). Thus, based on the results obtained, the as-prepared anion cluster is most likely to be described as [ZnCl3·O]− (Fig. 4), whose vibrational frequency in the Raman spectra appeared at 287 cm−1; in this cluster the oxygen is supplied by the crown framework.
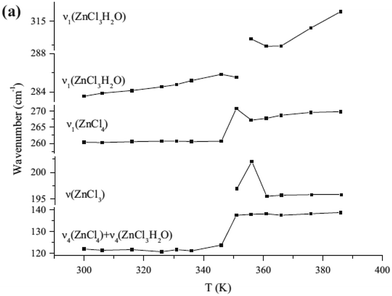 |
| Fig. 3 Temperature dependence of Raman frequencies.6 | |
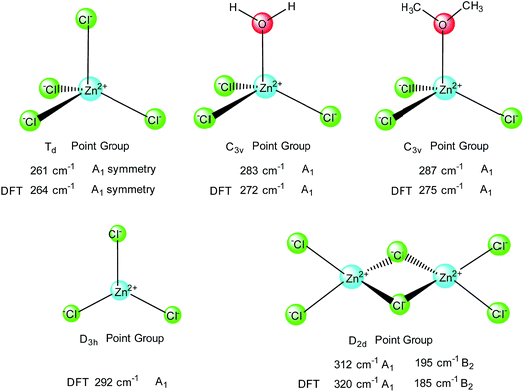 |
| Fig. 4 Proposed vibration frequencies related to their molecular point group and their vibration models. | |
DFT calculation support
The theoretical Raman spectra of these structures, as shown in Fig. 4, were obtained through quantum mechanical calculations. The calculations were carried out using the B3LYP functional.10,11 The 6-31G(d,p) basis set was used for the C, H, O and Cl atoms, while the LANL2DZ basis set was used for Zn atom. All the calculations were performed using the Gaussian 09 suite of programs and the bond lengths of the crystals were referenced without zero-point energy calibration and solvent effect consideration.12
Based on the DFT theoretical investigations we can infer that the computational Raman spectral data (Fig. 4, DFT data) of clusters ZnClx are consistent with the experimental data. The structure of [ZnCl3·O]− was replaced by [ZnCl3·OMe2]− and the DFT calculated peak from the spectral data of [ZnCl3]− is around 292 cm−1, which is higher than that in both clusters, [ZnCl3·OH2]− and [ZnCl3·OMe2]−.
Conclusion
Based on the above discussion, our assignment for ZnClx− is not “in stark contrast with the current knowledge”. The vibrational frequency at 287 cm−1 is reported for the first time and could be in agreement with a band corresponding to [ZnCl3·O]− without crystal data. We shall accept criticism with an open mind and consider that the assignment of cluster ZnClx− in our as-prepared ILs belongs to anion cluster of [ZnCl4]2− as suggested by Małgorzata Swadźba-Kwaśny would be incorrect based on the experiments and DFT calculations.
Finally, we agree that a trigonal planar structure of cluster [ZnCl3]− is not proven in liquid or solid phase and that the anion geometry is more likely to be tetrahedral based on a number of crystallographic evidences of [ZnCl3·X]− species.6,8 However the [ZnCl3]− formulation has been used by others for many years simply to denote composition of anions. This is an old issue and does not in any way affect the issue of the catalytic activity of the as-prepared ILs reported in our study.
Conflicts of interest
There are no conflicts to declare.
Acknowledgements
Natural Science Foundation of Gansu Province (17JR5RA212).
References
- Y. Liang, J. Wang, C. Cheng and H. Jing, RSC Adv., 2016, 6, 93546 RSC.
- Y. Song, H. Jing, B. Li and D. Bai, Chem.–Eur. J., 2011, 17, 8731 CrossRef CAS PubMed.
- E. S. Ferrari, K. J. Roberts, G. B. Thomson, J. D. Gale and C. R. A. Catlow, Acta Crystallogr., Sect. A: Found. Crystallogr., 2011, 57, 264 CrossRef.
- M. Quilichini, P. Bernede, J. Lefebvre and P. Schweiss, J. Phys.: Condens. Matter, 1990, 2, 4543 CrossRef CAS.
- R. J. Bouma, J. H. Teuben, W. R. Beukema, R. L. Bansemer, J. C. Huffman and K. G. Caulton, Inorg. Chem., 1984, 23, 2715 CrossRef CAS.
- M. B. Gzaiel, A. Oueslati, J. Lhoste, M. Gargouri and A. Bulou, J. Mol. Struct., 2015, 1089, 153 CrossRef.
- M. B. Nasr, E. Jeanneau and C. B. Nasr, Am. J. Anal. Chem., 2015, 6, 189 CrossRef.
- W. Li, C.-T. He, Y. Zeng, C.-M. Ji, Z.-Y. Du, W.-X. Zhang and X.-M. Chen, J. Am. Chem. Soc., 2017, 139, 8086 CrossRef CAS PubMed.
- I. V. Pekov, N. V. Zubkova, S. N. Britvin, V. O. Yapaskurt, N. V. Chukanov, I. S. Lykova, E. G. Sidorov and D. Yu. Pushcharovsky, Eur. J. Mineral., 2015, 27, 805 CrossRef CAS.
- C. Lee, W. Yang and R. G. Parr, Phys. Rev. B, 1988, 37, 785 CrossRef CAS.
- A. D. Becke, J. Chem. Phys., 1993, 98, 5648 CrossRef CAS.
- M. J. Frisch, G. W. Trucks, H. B. Schlegel, G. E. Scuseria, M. A. Robb, J. R. Cheeseman, G. Scalmani, V. Barone, B. Mennucci, G. A. Petersson, H. Nakatsuji, M. Caricato, X. Li, H. P. Hratchian, A. F. Izmaylov, J. Bloino, G. Zheng, J. L. Sonnenberg, M. Hada, M. Ehara, K. Toyota, R. Fukuda, J. Hasegawa, M. Ishida, T. Nakajima, Y. Honda, O. Kitao, H. Nakai, T. Vreven, J. A. Montgomery Jr, J. E. Peralta, F. Ogliaro, M. Bearpark, J. J. Heyd, E. Brothers, K. N. Kudin, V. N. Staroverov, R. Kobayashi, J. Normand, K. Raghavachari, A. Rendell, J. C. Burant, S. S. Iyengar, J. Tomasi, M. Cossi, N. Rega, N. J. Millam, M. Klene, J. E. Knox, J. B. Cross, V. Bakken, C. Adamo, J. Jaramillo, R. Gomperts, R. E. Stratmann, O. Yazyev, A. J. Austin, R. Cammi, C. Pomelli, J. W. Ochterski, R. L. Martin, K. Morokuma, V. G. Zakrzewski, G. A. Voth, P. Salvador, J. J. Dannenberg, S. Dapprich, A. D. Daniels, O. Farkas, J. B. Foresman, J. V. Ortiz, J. Cioslowski and D. J. Fox, Gaussian 09, Revision, D. 01, Gaussian, Inc., Wallingford CT, 2013 Search PubMed.
|
This journal is © The Royal Society of Chemistry 2017 |