DOI:
10.1039/C7RA08916C
(Paper)
RSC Adv., 2017,
7, 43648-43654
Extremely low coercivity in Fe3O4 thin film grown on Mg2TiO4 (001)
Received
12th August 2017
, Accepted 14th August 2017
First published on 11th September 2017
Abstract
We report very different magnetic properties of 40 nm-thick Fe3O4 thin films grown on tailored spinel substrate Mg2TiO4 (001) and on general substrate MgO (001). The sample on Mg2TiO4 (001) shows a very sharp Verwey transition with narrow hysteresis of only 0.5 K and a high transition temperature up to 126 K and, in particular, an extremely small coercivity as low as around 7 Oe from the Verwey transition to room temperature. This low coercivity is close to that of the single crystal bulk but several times smaller than that of the sample on MgO (001). Our work gives a first example of the magnetic properties in Fe3O4 thin film having higher Verwey transition than that of the single crystal bulk, which not only greatly expands our understanding about Fe3O4 but also provides a very good candidate for spintronic applications with quite low energy consumption.
I. Introduction
Magnetite (Fe3O4) is one of the most studied transition-metal oxides over the past several decades because of its rather unique and interesting set of magnetic and electrical properties, such as high Curie temperature (TC = 858 K), relatively high saturation magnetization, small coercivity field1,2 and theoretically predicted half-metallic character.3,4 These properties make Fe3O4 very attractive for room temperature spintronic applications.5–9 Especially, Fe3O4 is a highly correlated material that undergoes a first-order metal–insulator transition (known as the Verwey transition10) at TV = 124 K, but the mechanism of this transition is still unclear though tremendous amount of work has been done. However, the unique properties, which are relevant for various device applications, have been very difficult to realize in thin film form due to the existence of growth defects (such as the anti-phase boundaries (APBs)) and chemical-off stoichiometry. The inevitable presence of APBs in Fe3O4 thin films generally results in some unusual magnetic and transport properties, such as unsaturated magnetization in high magnetic fields,11,12 superparamagnetic behavior for epitaxial ultrathin films,13,14 unsaturated negative magnetoresistance,15–24 and very low Verwey temperature and quite broadened transition.15–19,21,22,24–26 Therefore, previous work of Fe3O4 thin films grown on MgO, MgAl2O4, SrTiO3 or Al2O3 substrates in fact only reported the extrinsic magnetic and transport properties.11–26
To overcome these negative aspects, very recently, Liu et al.27 obtained exceptionally high quality epitaxial Fe3O4 thin films grown on tailor-made spinel Co2−x−yMnxFeyTiO4 (001) substrates, which not only show the Verwey transition as sharp as the single crystal bulk but also present very high TV up to 136.5 K. This work provides a completely new platform to further investigate the intrinsic physical properties of the magnetite.27 The Co2−x−yMnxFeyTiO4 substrates, however, are magnetic, which significantly restricts the study of the magnetic properties in these high-quality Fe3O4 thin films. Furthermore, up to now, all the investigations on the magnetic properties of Fe3O4 thin films are done in the films grown on the general substrates such as MgO, MgAl2O4, SrTiO3 or Al2O3,9,11,12,15–19,21,22,24 which only show lower TV than that of the bulk due to the existence of microstructure defects. Therefore, to study the magnetic properties in Fe3O4 thin film with higher TV than that of the bulk will greatly extend our understanding about the magnetite. To experimentally achieve this goal, based on the work in ref. 27, we carefully chose and made a new non-magnetic spinel substrate Mg2TiO4 (001) with small lattice mismatch +0.51%. We expect that the Fe3O4 thin film grown this substrate will present higher TV than that of the bulk and exhibit quite different magnetic properties from that of the films grown on the general substrates.
In this work, we report very different magnetic properties of 40 nm-thick Fe3O4 thin films grown on Mg2TiO4 (001) and MgO (001) substrates. It is found that the sample on Mg2TiO4 (001) displays a very sharp Verwey transition with narrow hysteresis of 0.5 K and a high TV of 126 K, and remarkably an extremely small coercivity as low as around 7 Oe from Verwey transition to room temperature. This so low coercivity is close to that of the single crystal bulk but several times smaller than that of the sample on MgO (001), which makes Fe3O4/Mg2TiO4 (001) a very good candidate for spintronic applications in quite low energy consumption.
II. Experiments
The 40 nm-thick Fe3O4 thin films were grown on Mg2TiO4 (001) and MgO (001) substrates by using molecular beam epitaxy (MBE) in an ultrahigh vacuum system with a background pressure of 1 × 10−10 mbar range. The substrates were annealed for 2 h at 600 °C in an oxygen pressure of 3 × 10−7 mbar to obtain a clean and well-ordered surface structure before the deposition of Fe3O4. Standard samples were grown using an iron flux of 1 Å per minute, an oxygen background pressure of 1 × 10−6 mbar, and a growth temperature of 250 °C.26 To determine the structural quality and chemical states, the films were analyzed in situ by using reflection high-energy electron diffraction (RHEED), low-energy electron diffraction (LEED) and X-ray photoemission spectroscopy (XPS). The RHEED patterns were taken at 20 keV electron energy, with the beam aligned parallel to the [100] direction of the substrate. The LEED patterns were recorded at electron energy of 88 eV. The thickness of the film was determined during growth from the oscillation period of the RHEED specular spot intensity. The XPS data were collected using 1486.6 eV photons (monochromatized Al Kα light) in normal emission geometry and at room temperature using a Scienta R3000 electron energy analyzer. The overall energy resolution was set to about 0.3 eV. The transport and magnetic properties of the Fe3O4 thin films were ex situ measured with a standard four probe technique using a physical property measurement system (PPMS) and superconducting quantum interference device (SQUID), respectively. High-resolution X-ray diffraction (HR-XRD) was employed for further ex situ investigation of the structural quality and the microstructure of the thin films. The XRD measurements were performed with a high resolution PANalytical X'Pert MRD diffractometer using monochromatic Cu Kα1 radiation (λ = 1.54056 Å).
III. Results and discussions
Fig. 1 shows the RHEED electron diffraction patterns of clean substrates Mg2TiO4 (001) (a) and MgO (001) (b), the RHEED and LEED patterns of 40 nm-thick Fe3O4 thin films grown on Mg2TiO4 (001) (c and e), and on MgO (001) (d and f), respectively. The sharp RHEED streaks and the presence of Kikuchi lines (Fig. 1(c) and (d)), as well as the high contrast and sharp LEED spots (Fig. 1(e) and (f)) indicate a flat and well ordered (001) single crystalline surface structure of both samples. The characteristic (
)R45° surface reconstruction of Fe3O4 (001) can be observed, providing another indication for the high structural quality of the two Fe3O4 thin films. The (1 × 1) unit cell and the (
)R45° superlattice are indicated by the red dashed square and solid square, respectively (see Fig. 1(e) and (f)).28,29 Moreover, it is found that the LEED pattern for the film on Mg2TiO4 (001) has 45° rotation as compared to the film on MgO (001), which should be due to the direction rotation of the substrate during its production process. Furthermore, to clarify the chemical states of the iron oxide, the thin films were in situ analyzed by XPS, as shown in Fig. 2(a)–(c). It is clear that the two samples exhibit the same wide scan spectra with binding energy from 1200 to −18 eV (Fig. 2(a)), Fe 2p core-level spectra (Fig. 2(b)) and valence band spectra (Fig. 2(c)), which demonstrates quite clean surface of the thin films and represents the typical signatures of Fe3O4 thin film.26,27,30,31 The structural quality of the thin films was further ex situ investigated by the high-resolution X-ray diffraction (HR-XRD). As shown in the Fig. 2(d), the long range θ–2θ XRD patterns do not present any phase other than Fe3O4, the (002)/(004) and (004)/(008) reflections correspond to MgO/Fe3O4 because of the lattice constant of Fe3O4 as twice as that of MgO (see the green color curve), and the (004) and (008) reflections are presented for both Mg2TiO4 and Fe3O4 for the red color curve. The two samples are in fully strained due to the small lattice mismatches.27,32 As the lattice mismatch of Mg2TiO4 (+0.51%) is larger than that of MgO (+0.33%), the tensile strain and also the lattice constant a (in-plane) are bigger for the former, and thus the lattice constant c (out-of-plane) of the Fe3O4/Mg2TiO4 (001) (8.343 Å) is smaller than that of the Fe3O4/MgO (001) (8.367 Å), corresponding to the relative shift of (004) and (008) peaks to the larger angles (see the red curve).
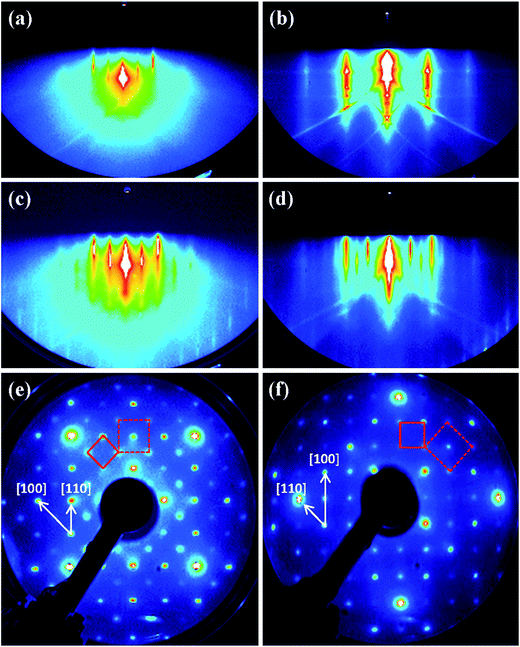 |
| Fig. 1 RHEED and LEED electron diffraction patterns of the following: the clean substrates Mg2TiO4 (001) (a) and MgO (b); 40 nm-thick Fe3O4 thin films grown on Mg2TiO4 (001) (c) and (e), and on MgO (001) (d) and (f), respectively. The (1 × 1) unit cell and the ( )R45° superlattice are indicated by the red dashed square and solid square, respectively. | |
 |
| Fig. 2 XPS spectra of 40 nm-thick Fe3O4 thin films grown on Mg2TiO4 (001) and MgO (001): wide scan spectra (a), Fe 2p core-level spectra (b) and valence band spectra, as well as Ag for reference (c); (d) X-ray diffraction patterns for the Fe3O4 thin films on Mg2TiO4 (001) and MgO (001). Only the (004) and (008) reflections of Fe3O4 can be observed for the two samples. | |
The resistivity as a function of temperature ρ(T) of 40 nm-thick Fe3O4 thin films grown on Mg2TiO4 (001) and MgO (001), and of single crystal bulk is shown in Fig. 3(a). It is found that the ρ(T) curves present a clear first-order Verwey transition. The Fe3O4/MgO (001) sample displays a low TV with a big hysteretic loop of about 4 K whereas the Fe3O4/Mg2TiO4 (001) sample exhibits a higher TV (126 K) than that of the bulk with very narrow hysteresis of only 0.5 K, which demonstrates that the Fe3O4/Mg2TiO4 (001) sample has quite few microstructural defects and the tensile strain pushes the TV over that of the bulk.27 The temperature dependence of magnetization M(T) of 40 nm-thick Fe3O4 thin films on Mg2TiO4 (001) and MgO (001) are exhibited in Fig. 3(b) and (c), respectively. It is obvious that a sharp jump of magnetization takes place at Verwey transition. The TV− and TV+ are defined respectively as the temperature of the maximum slop of log(ρ(T)) or M(T) curve for the cooling down and warming up temperature branches. Clearly, the TV+ and TV− from the zero-field-cooling (ZFC) and field-cooling (FC) M(T) curves in Fig. 3(b) and (c) are consistent with that from ρ(T) curves in Fig. 3(a) (see the insets of Fig. 3(a)–(c)). Furthermore, it is found that the FC curve of Fe3O4/Mg2TiO4 (001) film shows much larger magnetization change at TV− than that of Fe3O4/MgO (001) film (see Fig. 3(b) and (c)). Although the bulk Fe3O4 keeps ferrimagnetic below TC ∼ 860 K, yet the easy (hard) axis changes from [111] ([100]) to [100] ([111]) with changing from cubic Fd
m to monoclinic Cc.33,34 Usually, the bulk Fe3O4 exhibits a very sharp variation of magnetization at TV under small magnetic field.35 In Fe3O4 thin films, however, the presence of microstructure defects can negatively affect the rotation of the magnetic axis. Therefore, the Fe3O4/Mg2TiO4 (001) film having fewer microstructure defects is more sensitive to the applied magnetic field, and the much larger magnetization change at TV− can be observed.
 |
| Fig. 3 Resistivity as a function of temperature for 40 nm-thick Fe3O4 thin films on Mg2TiO4 (001) and MgO (001), and of single crystal bulk Fe3O4 (a). Inset: temperature dependence of dlog(ρ)/dT near the TV for the samples on Mg2TiO4 (001) (up) and MgO (001) (down), respectively; zero-field-cooling (ZFC) and field-cooling (FC) magnetization of the samples on Mg2TiO4 (001) (b) and MgO (001) (c), respectively. The dM/dT versus temperature around the TV for the thin films on Mg2TiO4 (001) and MgO (001) are displayed as insets of (b) and (c), respectively. | |
It has been reported that the coercivity field (HC) significantly enhances with the Fe3O4 transforming from high-temperature cubic spinel structure to low-temperature monoclinic structure due to the abrupt increase in magnetocrystalline and magnetostriction constants.35–38 The in-plane magnetic hysteresis loops at 126 K (at TV) and 127 K (just above TV) in applied field of 50 kOe of the 40 nm Fe3O4/Mg2TiO4 (001) film is shown in Fig. 4(a). It is observed that the HC sharply decreases from 213 Oe at 126 K to only 6 Oe at 127 K (see the inset (left) of Fig. 4(a)). Remarkably, this extremely small HC at 127 K, to our knowledge, is the smallest value in Fe3O4 thin films so far, which is close to that of the single crystal bulk36 but several times smaller than that reported in thin films grown on MgO, MgAl2O4, SrTiO3 or Al2O3.9,15,16,19,21,22,24,37,38 Moreover, near the HC, a clear incoherent reversal of magnetization with magnetic field for 126 K while a rapid jump of magnetization with magnetic field for 127 K are observed, see the sharp peak of d(M/MS)/dH at 127 K in the inset (right) of Fig. 4(a).
 |
| Fig. 4 (a) In-plane magnetic hysteresis loops in applied field of 50 kOe for 40 nm-thick Fe3O4 thin film grown on Mg2TiO4 (001) at 126 (at TV) and 127 K (just above TV). Inset: (left) magnetic hysteresis loop under small magnetic field 0.1 kOe at 127 K; (right) d(M/MS)/dH as a function of magnetic field around the HC; (b) in-plane and out-of-plane magnetic hysteresis loops of the sample on Mg2TiO4 (001) at 300 K. Inset: d(M/MS)/dH vs. H around the HC; (c) temperature dependence of HC (in-plane) for 40 nm-thick Fe3O4 thin films on Mg2TiO4 (001) and MgO (001). A sharp change of HC can be seen at their Verwey transitions and the values of HC for the sample on Mg2TiO4 (001) are much smaller than that of the sample on MgO (001). | |
The in-plane HC as a function of temperature for 40 nm-thick Fe3O4 thin films on Mg2TiO4 (001) and MgO (001) are plotted in Fig. 4(c). A sharp change of HC occurs at their Verwey transitions for the two samples, respectively. Especially, the Fe3O4/Mg2TiO4 (001) sample keeps nearly constant HC of only about 7 Oe above its TV. As a contrast, the values of HC are much larger for the Fe3O4/MgO (001) sample, ranging from 140 Oe at 130 K to 90 Oe at 300 K and still about two times bigger than that of the Fe3O4/Mg2TiO4 (001) sample at low temperatures (T < TV). Furthermore, the two samples present the perpendicular anisotropic behavior, that the in-plane and out-of-plane correspond to the easy and hard axis, respectively (see Fig. 4(b)), and the anisotropic field is about 5 kOe, similar to that reported in previous work.20,39 It has been known that the microstructural defects (such as the APBs) greatly affect the transport and magnetic properties of Fe3O4 thin films, the APBs were claimed to act as pining centers for the magnetic domain walls,37 thus the substantial enhancement of HC for the Fe3O4/MgO (001) film should be induced from this effect. As a result, by using a tailored spinel substrate we can obtain exceptionally high quality Fe3O4 thin film, with getting rid of the microstructure defects, it is the first time for us to observe the magnetic properties in Fe3O4 thin film having higher TV than that of the single crystal bulk, which enlarges our understanding about the Fe3O4. Furthermore, this Fe3O4/Mg2TiO4 (001) thin film with extremely low coercivity will bring in quite low energy consumption in spin valves or spin tunnel junctions.
IV. Conclusion
In summary, we have studied the magnetic properties of 40 nm-thick Fe3O4 thin films grown on Mg2TiO4 (001) and on MgO (001). We found that the Fe3O4/Mg2TiO4 (001) film shows a very sharp Verwey transition with narrow hysteresis and high TV up to 126 K, and especially an extremely small HC as low as about 7 Oe from the Verwey transition to room temperature. This small HC is close to that of the single crystal bulk but several times smaller than that of the films grown on general substrates. Our work not only gives a first example of the magnetic properties in Fe3O4 thin film having higher Verwey transition than that of the single crystal bulk but also provides a very good candidate for spintronic applications in quite low energy consumption.
Conflicts of interest
There are no conflicts to declare.
Acknowledgements
The authors would like to thank Prof. Liu Hao Tjeng, Dr Chun-Fu Chang and Dr Alexander Komarek for useful discussion. This work has been supported by the Max Planck-POSTECH Center for Complex Phase Materials, and the National Basic Research Program of China (No. 2017YFA0206302), and the National Nature Science Foundation of China under projects 51590883 and 51331006, and as a project of the Chinese Academy of Sciences with grant number KJZD-EW-M05-3.
References
- F. Walz, The Verwey transition – a topical review, J. Phys.: Condens. Matter, 2002, 14, R285–R340 CrossRef CAS.
- J. Garcia and G. Subias, The Verwey transition – a new perspective, J. Phys.: Condens. Matter, 2004, 16, R145–R178 CrossRef CAS.
- R. A. de Groot, F. M. Mueller, P. G. van Engen and K. H. J. Buschow, New Class of Materials: Half-Metallic Ferromagnets, Phys. Rev. Lett., 1983, 50, 2024–2027 CrossRef CAS.
- A. Yanase and K. Siratori, Band structure in the high temperature phase of Fe3O4, J. Phys. Soc. Jpn., 1984, 53, 312–317 CrossRef CAS.
- X. W. Li, A. Gupta, G. Xiao, W. Qian and V. P. Dravid, Fabrication and properties of heteroepitaxial magnetite (Fe3O4) tunnel junctions, Appl. Phys. Lett., 1998, 73, 3282–3284 CrossRef CAS.
- G. Hu and Y. Suzuki, Negative Spin Polarization of Fe3O4 in Magnetite/Manganite-Based Junctions, Phys. Rev. Lett., 2002, 89, 276601 CrossRef CAS PubMed.
- L. M. B. Alldredge, R. V. Chopdekar, B. B. Nelson-Cheeseman and Y. Suzuki, Spin-polarized conduction in oxide magnetic tunnel junctions with spinel/perovskite interfaces, Appl. Phys. Lett., 2006, 89, 182504 CrossRef.
- T. Kado, Large room-temperature inverse magnetoresistance in tunnel junctions with a Fe3O4 electrode, Appl. Phys. Lett., 2008, 92, 092502 CrossRef.
- J. B. Moussy, From epitaxial growth of ferrite thin films to spin-polarized tunneling, J. Phys. D: Appl. Phys., 2013, 46, 143001 CrossRef.
- E. J. W. Verwey, Electronic conduction of magnetite (Fe3O4) and its transition point at low temperatures, Nature, 1939, 144, 327–328 CrossRef CAS.
- D. T. Margulies, F. T. Parker, F. E. Spada, R. S. Goldman, J. Li, R. Sinclair and A. E. Berkowitz, Anomalous moment and anisotropy behavior in Fe3O4 films, Phys. Rev. B, 1996, 53, 9175–9187 CrossRef CAS.
- D. T. Margulies, F. T. Parker, M. L. Rudee, F. E. Spada, J. N. Chapman, P. R. Aitchison and A. E. Berkowitz, Origin of the Anomalous Magnetic Behavior in Single Crystal Fe3O4 Films, Phys. Rev. Lett., 1997, 79, 5162–5165 CrossRef CAS.
- F. C. Voogt, T. T. M. Palstra, L. Niesen, O. C. Rogojanu, M. A. James and T. Hibma, Superparamagnetic behavior of structural domains in epitaxial ultrathin magnetite films, Phys. Rev. B: Condens. Matter Mater. Phys., 1998, 57, R8107–R8110 CrossRef CAS.
- W. Eerenstein, T. Hibma and S. Celotto, Mechanism for superparamagnetic behavior in epitaxial Fe3O4 films, Phys. Rev. B: Condens. Matter Mater. Phys., 2004, 70, 184404 CrossRef.
- G. Q. Gong, A. Gupta, G. Xiao, W. Qian and V. P. Dravid, Magnetoresistance and magnetic properties of epitaxial magnetite thin films, Phys. Rev. B: Condens. Matter Mater. Phys., 1997, 56, 5096–5099 CrossRef CAS.
- X. W. Li, A. Gupta, G. Xiao and G. Q. Gong, Transport and magnetic properties of epitaxial and polycrystalline magnetite thin films, J. Appl. Phys., 1998, 83, 7049–7051 CrossRef CAS.
- M. Ziese and H. J. Blythe, Magnetoresistance of magnetite, J. Phys.: Condens. Matter, 2000, 12, 13–28 CrossRef CAS.
- M. Ziese, Extrinsic magnetotransport phenomena in ferromagnetic oxides, Rep. Prog. Phys., 2002, 65, 143–249 CrossRef CAS.
- M. Ziese, R. Hohne, H. C. Semmelhack, H. Reckentin, N. H. Hong and P. Esquinazi, Mechanism of grain-boundary magnetoresistance in Fe3O4 films, Eur. Phys. J. B, 2002, 28, 415–422 CrossRef CAS.
- W. Eerenstein, T. T. M. Palstra, S. S. Saxena and T. Hibma, Spin-Polarized Transport across Sharp Antiferromagnetic Boundaries, Phys. Rev. Lett., 2002, 88, 247204 CrossRef CAS PubMed.
- S. K. Arora, R. G. S. Sofin and I. V. Shvets, Magnetoresistance enhancement in epitaxial magnetite films grown on vicinal substrates, Phys. Rev. B: Condens. Matter Mater. Phys., 2005, 72, 134404 CrossRef.
- A. V. Ramos, J. B. Moussy, M. J. Guittet, A. M. Bataille, M. G. Soyer, M. Viret, C. Gatel, P. B. Guillemaud and E. Snoeck, Magnetotransport properties of Fe3O4 epitaxial thin films: Thickness effects driven by antiphase boundaries, J. Appl. Phys., 2006, 100, 103902 CrossRef.
- A. F. Pacheco, J. Orna, J. M. De Teresa, P. A. Algarabel, L. Morellon, J. A. Pardo, M. R. Ibarra, E. Kampert and U. Zeitler, High-field Hall effect and magnetoresistance in Fe3O4 epitaxial thin films up to 30 Tesla, Appl. Phys. Lett., 2009, 95, 262108 CrossRef.
- R. G. S. Sofin, S. K. Arora and I. V. Shvets, Positive antiphase boundary domain wall magnetoresistance in Fe(110) heteroepitaxial films, Phys. Rev. B: Condens. Matter Mater. Phys., 2011, 83, 134436 CrossRef.
- W. Eerenstein, T. T. M. Palstra, T. Hibma and S. Celotto, Origin of the increased resistivity in epitaxial Fe3O4 films, Phys. Rev. B: Condens. Matter Mater. Phys., 2002, 66, 201101 CrossRef.
- X. H. Liu, A. D. Rata, C. F. Chang, A. C. Komarek and L. H. Tjeng, Verwey transition in Fe3O4 thin films: Influence of oxygen stoichiometry and substrate-induced Microstructure, Phys. Rev. B: Condens. Matter Mater. Phys., 2014, 90, 125142 CrossRef.
- X. H. Liu, C. F. Chang, A. D. Rata, A. C. Komarek and L. H. Tjeng, Fe3O4 thin films: controlling and manipulating an elusive quantum material, npj Quantum Materials, 2016, 1, 16027 CrossRef.
- F. Y. Ran, Y. Tsunemaru, T. Hasegawa, Y. Takeichi, A. Harasawa, K. Yaji, S. Kim and A. Kakizaki, Angle-resolved photoemission study of Fe3O4(001) films across Verwey transition, J. Phys. D: Appl. Phys., 2012, 45, 275002 CrossRef.
- W. Wang, J. M. Mariot, M. C. Richter, O. Heckmann, W. Ndiaye, P. De Padova, A. Taleb-Ibrahimi, P. Le Fèvre, F. Bertran, F. Bondino, E. Magnano, J. Krempasky, P. Blaha, C. Cacho, F. Parmigiani and K. Hricovini, Fe t2g band dispersion and spin polarization in thin films of Fe3O4(001)/MgO(001): Half-metallicity of magnetite revisited, Phys. Rev. B: Condens. Matter Mater. Phys., 2013, 87, 085118 CrossRef.
- S. A. Chambers and S. A. Joyce, Surface termination, composition and reconstruction of Fe3O4(001) and γ-Fe2O3(001), Surf. Sci., 1999, 420, 111–122 CrossRef CAS.
- S. A. Chambers, R. F. C. Farrow, S. Maat, M. F. Toney, L. Folks, J. G. Catalano, T. P. Trainor and G. E. Brown Jr, Molecular beam epitaxial growth and properties of CoFe2O4 on MgO(001), J. Magn. Magn. Mater., 2002, 246, 124–139 CrossRef CAS.
- M. Luysberg, R. G. S. Sofin, S. K. Arora and I. V. Shvets, Strain relaxation in Fe3O4/MgAl2O4 heterostructures: Mechanism for formation of antiphase boundaries in an epitaxial system with identical symmetries of film and substrate, Phys. Rev. B: Condens. Matter Mater. Phys., 2009, 80, 024111 CrossRef.
- B. A. Calhoun, Magnetic and Electric Properties of Magnetite at Low Temperatures, Phys. Rev., 1954, 94, 1577–1585 CrossRef CAS.
- Z. Kakol, Magnetic and transport properties of magnetite in the vicinity of the Verwey transition, J. Solid State Chem., 1990, 88, 104–114 CrossRef CAS.
- A. R. Muxworthy and E. McClelland, Review of the low-temperature magnetic properties of magnetite from a rock magnetic perspective, Geophys. J. Int., 2000, 140, 101–114 CrossRef.
- A. R. Muxworthy, Low-temperature susceptibility and hysteresis of magnetite, Earth Planet. Sci. Lett., 1999, 169, 51–58 CrossRef CAS.
- A. Bollero, M. Ziese, R. Hohne, H. C. Semmelhack, U. Kohler, A. Setzer and P. Esquinazi, Influence of thickness on microstructural and magnetic properties in Fe3O4 thin films produced by PLD, J. Magn. Magn. Mater., 2005, 285, 279–289 CrossRef CAS.
- F. Delille, B. Dieny, J. B. Moussy, M. J. Guittet, S. Gota, M. G. Soyer and C. Marin, Study of the electronic paraprocess and antiphase boundaries as sources of the demagnetisation phenomenon in magnetite, J. Magn. Magn. Mater., 2005, 294, 27–39 CrossRef CAS.
- P. A. A. van der Heijden, M. G. van Opstal, C. H. W. Swuste, P. H. J. Bloemen, J. M. Gaines and W. J. M. de Jonge, A ferromagnetic resonance study on ultra-thin Fe3O4 layers grown on (0 0 1) MgO, J. Magn. Magn. Mater., 1998, 182, 71–80 CrossRef CAS.
|
This journal is © The Royal Society of Chemistry 2017 |
Click here to see how this site uses Cookies. View our privacy policy here.