DOI:
10.1039/C7RA08250A
(Paper)
RSC Adv., 2017,
7, 43266-43272
Selenium–phycocyanin from selenium-enriched cultures of Nostoc sp. isolated from rice field prevents human kidney cells from paraquat-induced damage
Received
26th July 2017
, Accepted 28th August 2017
First published on 6th September 2017
Abstract
Selenium-containing phycocyanin (Se–PC) was purified from Se-enriched cultures of a nitrogen-fixing cyanobacterium (Nostoc sp.) using fast protein liquid chromatography. Ammonium sulfate precipitation, DEAE-Sepharose ion-exchange chromatography, and Sephacry S-300 size exclusion chromatography were employed in the process of protein purification. The purity ratio and the separation factor of the purified Se–PC were found to be 5.97 and 14.73, respectively. The Se content in the Se–PC was 550 μg g−1 protein as determined by ICP-AES analysis. Interestingly, we also found that, Se–PC could effectively suppress the paraquat (PQ)-induced cell death and morphological changes, reverse PQ-induced DNA fragmentation and nuclear condensation, and block PQ-induced cell apoptosis through inhibition of superoxide overproduction in human kidney HK-2 cells. The results indicate that Se–PC is a promising selenium species for the protection of renal cells from paraquat-induced damage.
1. Introduction
Paraquat (PQ) is a bipyridylium herbicide widely-used to prepare the land for planting and for controlling weeds in many crops around the world.1 It has been demonstrated to be a highly toxic compound for humans and animals.2 Moreover, due to its inherent toxicity and lack of effective treatment, the rate of death caused by PQ is very high. It can cause multiple-organ failure, involving the lung, liver, and kidney.1 For this reason, PQ has been banned in the European Union.3 As a potent free radical generator, PQ toxicity has been found mediated by the production of free radicals, which resulted in oxidative damage to cells.4,5 Therefore, free radical scavengers and antioxidants have been expected to be candidates for preventing PQ toxicity.1,5 Some substances possessing free radical scavenging and antioxidant properties have been tested for protective capability in PQ-induced damage, such as vitamin C, vitamin E, melatonin, glutathione, docosahexaenoic acid, etc.2,5–8
Cyanobacteria are considered biotechnologically interesting organisms since they produce many valuable products, such as phycobiliprotein.9 Some cyanobacteria with nitrogen-fixing ability are able to survive under nitrogen-free environments, which makes these microalgae a good option to cultivate on a large scale for biotechnological purposes, may reduce contamination risk and production costs of cultivation.10 Indeed, as edible blue-green algae, Nostoc species including N. flagelliforme and N. commune have been used as food for thousands of years in China.11 There have been some reports about purification of PC from nitrogen-fixing cyanobacteria.12–14 Moreover, Sun et al.15 recorded that phycocyanin (PC) treatment significantly attenuated PQ-induced acute lung injury in rats. At the same time, oral supplementation inorganic Se showed beneficial effects on the overall antioxidant potential of the animals because it can protect cells against the effects of free radicals.16 There are some reports that show antioxidant activity of selenium-containing phycobiliproteins purified from Se-enriched S. platensis.17–19 In previous determinations, selenium-containing PC (Se–PC) purified from Se-enriched S. platensis showed enhanced antioxidant abilities against oxidative damage in human erythrocytes through inhibition of reactive oxygen species (ROS) generation.20 However, no information about Se–PC for PQ-induced injury treatment is available. In the current study, Se–PC was purified from a selenium-enriched diazotrophic cyanobacterium Nostoc sp., and the protective effects and mechanism of Se–PC against PQ-induced renal injury was investigated.
2. Materials and methods
2.1 Materials
The nitrogen-fixing cyanobacterium Nostoc sp. (FACHB-85) was obtained from the Freshwater Algae Culture Collection of the Institute of Hydrobiology, the Chinese Academy of Sciences and which was originally isolated from rice field in south China. DEAE-Sepharose was purchased from Sigma-Aldrich. The ultrapure water used in all experiments was supplied by a Milli-Q water purification system from Millipore. All the reagents used for cell culture experiments were bought from GIBCO.
2.2 Cultivation of high Se-enriched Nostoc sp. cells
Nostoc sp. was cultured with sterilized N-free mineral medium BG11 at 27 °C with a light illumination of 3000 lx and a light
:
dark cycle of 16 h
:
8 h. For the cultivation of Se-enriched Nostoc sp., a stepwise Se addition procedure was carried out based on the results of toxicological tests, where 2 mg L−1 Se in the form of sodium selenite was added to the medium on day 7, 8, and 9, respectively. The accumulative concentration of Se was 6 mg L−1. On day 14, algal cells were collected by centrifuging at 10
000g for 10 min at −4 °C and then lyophilized.
2.3 Separation and purification of PC and Se–PC by FPLC
The separation and purification procedure of Se–PC and PC was conducted by using the method of stepwise precipitation as we previously described.17,21 The sample was eluted with increasing NaCl concentration gradient. The pooled fractions were centrifuged, purified and desalted on a Sephacryl S-300 column.
2.4 Spectroscopic determination
A spectrophotometer method was employed to measure the UV-vis absorption spectra of testing samples. The purity ratio (A620/A280) and the separation factor (A620/A655) were calculated based on the UV-vis spectra.
2.5 Measurement of total Se
ICP-AES method was used to determine Se concentration. The sample was digested with 1 mL of H2O2 and 3 mL of concentrated nitric acid in a digestive stove (Qian Jian Measuring Instrument Co., Ltd., China) at 180 °C for 3 h. The digested product was reconstituted to 10 mL with Milli-Q water and used for total Se determination.
2.6 Cell culture and PQ treatment
Human kidney (HK-2) proximal tubular cell line was purchased from American Type Culture Collection (ATCC, Manassas, VA). The cells were incubated in Dulbecco's modified Eagle's medium (DMEM), supplemented with 10% fetal bovine serum (FBS), 100 U mL−1 penicillin, and 50 U mL−1 streptomycin in a humidified incubator with 5% CO2 atmosphere at 37 °C. HK-2 cells were seeded at a density of 6 × 104 cells mL−1 and pretreated with PQ for 3 h before the application of Se–PC or PC. Both living and dead cells were counted under microscopy with staining by trypan blue solution.
2.7 ABTS+ free radical scavenging activities
ABTS+ free radical scavenging activities of antioxidants were measured according to the method described by Zhang and co-workers.17 Briefly, 50 μL of the tested samples was mixed with 1 mL of ABTS reagent with absorbance of 0.70 ± 0.02 at 734 nm, and then the absorbance at 734 nm was measured after the initiation of mixing for 6 min.
2.8 Determination of caspase-3 activity
The caspase activity of the samples was assessed by measuring the fluorescence intensity of caspase substrates with the excitation and emission wavelength at 380 nm and 440 nm, respectively.22
2.9 TUNEL assay and DAPI staining
DNA fragmentation of HK-2 cells was detected by TUNEL assay followed the same procedure that described previously.22
2.10 Determination of ROS generation
The effects of PQ, Se–PC and PC on the ROS generation were assessed by using a DCFH-DA assay in HK-2 cells as described previously.23 Intracellular ROS level was quantified by measuring the fluorescence intensity of treated cells under a microplate reader (Bio-tek), with the excitation and emission wavelength at 488 nm and 525 nm, respectively.
2.11 Statistical analysis
All results are presented as mean ± standard deviation (SD) (n = 3). Statistical analysis was carried out by using SPSS statistical package (SPSS 13.0 for Windows; SPSS, Inc., Chicago, IL). The difference between two groups was analyzed by two-tailed Student's t test. Differences with P < 0.05 (*) were considered statistically significant. The difference between three or more groups was conducted using the one-way analysis of ANOVA followed by the Student–Newman–Keuls post hoc test. Bars with different characters are statistically different at P < 0.05.
3. Results and discussion
3.1 Purification of Se–PC and PC by FPLC and measurement of Se concentration
The results for purification of Se–PC from Se-enriched Nostoc sp. are shown in Fig. 1 and Table 1. First, a stepwise precipitation procedure using (NH4)2SO4 at 30% and 50% saturation degree was conducted.20 Contaminating proteins were precipitated in (NH4)2SO4 and Se–PC fraction was precipitated in (NH4)2SO4 at 50% saturation. As shown in Table 1, the stepwise precipitation enhanced the purity ratio (A620/A280) and the separation factor (A620/A655) of Se–PC to 2.94 and 5.17, respectively. The peak of Se–PC appeared at about 176 min and a rational separation of Se–PC from other algal proteins was visualized (Fig. 1A). The purity ratio and separation factor of Se–PC reached 4.26 and 10.86, respectively. An effective removal of other algal proteins before 120 min might be the key reason for achieving the high purity ratio of Se–PC. The purity was similar to previous works also employing DEAE ion exchange chromatography for PC purification from cyanobacteria.24–26 Sephacryl S-300 size exclusion chromatography was used for further purification of Se–PC. As a result, a sharp peak of Se–PC appeared in Fig. 1B. The purity ratio and separation factor of Se–PC after this step were increased to 5.97 and 14.73, respectively. As compared with methods addressed by other authors, the purification method in the present study gave a higher purity of Se–PC.24–26 Se content of protein obtained in different purification steps was shown in Table 1. After purification by ion exchange and size exclusion chromatography, Se–PC with the Se content at 550 μg g−1 was ultimately obtained. It is higher than that of Se–PC purified from S. platensis in our previous study.20
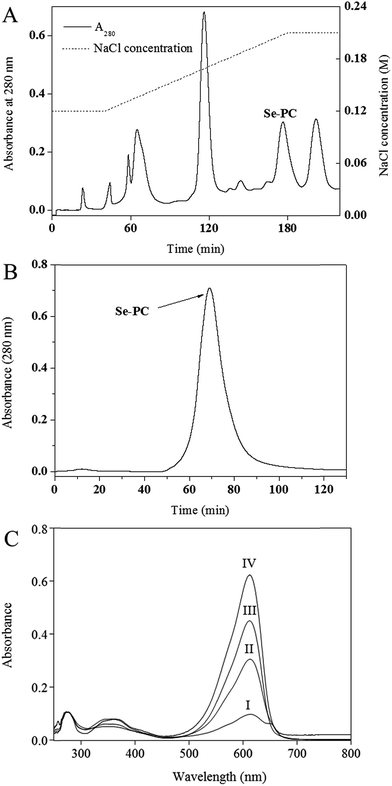 |
| Fig. 1 (A) DEAE-Sepharose chromatography of Nostoc sp. selenium–phycocyanin (Se–PC): the sample was eluted with increasing NaCl concentration gradient, first at 0.12 M for 40 min, followed by 0.12–0.21 M for 40 to 180 min and then maintained at 0.21 M until 220 min. The flow rate was 1.5 mL min−1. (B) Sephacryl S-300 chromatography of Nostoc sp. Se–PC: the sample was eluted with 2 mM Na-phosphate buffer (pH 7.0) at 1 mL min−1. (C) UV-vis spectra of Se–PC obtained in different steps of purification: (I) crude extract; (II) fraction obtained from 50% ammonium sulfate precipitation; (III) pooled fractions obtained from DEAE-Sepharose chromatography; (IV) pooled fractions obtained from Sephacryl S-300 chromatography. | |
Table 1 Purity ratio, separation factor and Se concentration of Se–PC obtained in different purification steps
Purification step |
Purity ratio (A620/A280) |
Separation factor (A620/A655) |
Se concentration (μg g−1 protein) |
Crude extract |
0.93 |
1.69 |
243 |
Precipitation with 50% saturation of (NH4)2SO4 |
2.94 |
5.17 |
317 |
DEAE-Sepharose fast flow chromatography |
4.26 |
10.86 |
— |
Sephacryl S-300 chromatography |
5.97 |
14.73 |
550 |
3.2 Characterization of Se–PC by UV-vis
The characteristic absorption peak at 620 nm and strong absorbance below 300 nm in the UV-vis spectra of various Se–PC fractions (shown in Fig. 1C) corroborated the presence of the Se–PC protein. The reduced absorption intensity of peaks at 280 nm and 655 nm indicated the enhanced purity of Se–PC in terms of purity ratio (A620/A280) and separation factor (A620/A655) (Table 1).
3.3 Antioxidant activity of PC and Se–PC
ABTS˙ free radical scavenging assay was used to determine the antioxidant activity of PC and Se–PC.21 In the present study, the antioxidant activity of Se–PC is represented as the inhibition percentage. As shown in Fig. 2, the suppression effect of Se–PC on ABTS oxidation was stronger than that of PC, indicating the greater antioxidant activity of Se–PC in hydrophilic environment. For instance, Se–PC shows dose-dependent inhibition of ABTS oxidation, and the IC50 (half maximal inhibitory concentration) of Se–PC (1.60 μM) and PC (3.44 μM) further confirmed this better antioxidant activity of Se–PC than that of PC.
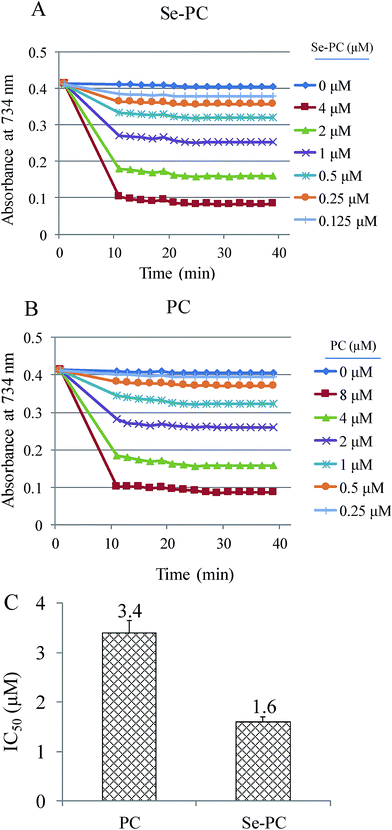 |
| Fig. 2 (A) Antioxidant activity of selenium–phycocyanin (Se–PC) by scavenging ABTS free radicals. (B) Antioxidant activity of PC by scavenging ABTS free radicals. (C) The IC50 indicating the half maximal inhibitory concentration at 30 min. | |
3.4 Se–PC attenuates PQ-induced nephrotoxicity in HK-2 cells
Our previous studies have showed that selenium-containing allophycocyanin purified from selenium-enriched S. platensis showed enhanced hepatoprotective effects through reversing oxidative stress-induced cytotoxicity in HepG2 human hepatoma carcinoma cells.27 To confirm the possibility that Se incorporation into PC is able to enhance its properties in prevention of PQ-induced nephrotoxicity, MTT reduction assay was used to evaluate the possible protective effects of Se–PC on HK-2 cells. As shown in Fig. 3A and B, the treatment of HK-2 cells with PQ time-dependently decreased HK-2 cell viability. For instance, cells treated with 800 μM PQ for 24 and 48 h decreased the cell viability by 25.7% and 46.8%, respectively. However, the pretreatment with Se–PC significantly relieved this cytotoxic effect, and Se–PC exhibited better protective effect than that of PC. Pretreatment with 4 μM Se–PC significantly increased the cell viability from 53.2% (PQ, 48 h) to 77.3% (Se–PC) while with 4 μM PC the cell viability did not significantly change in contrasted with PQ treatment. For instance, Se–PC treatment (less than 8 μM) showed no significant cytotoxicity toward HK-2 cells (Fig. 3C). Only with 16 μM Se–PC, the viability of HK-2 cells was drastically decreased by 14.7%.
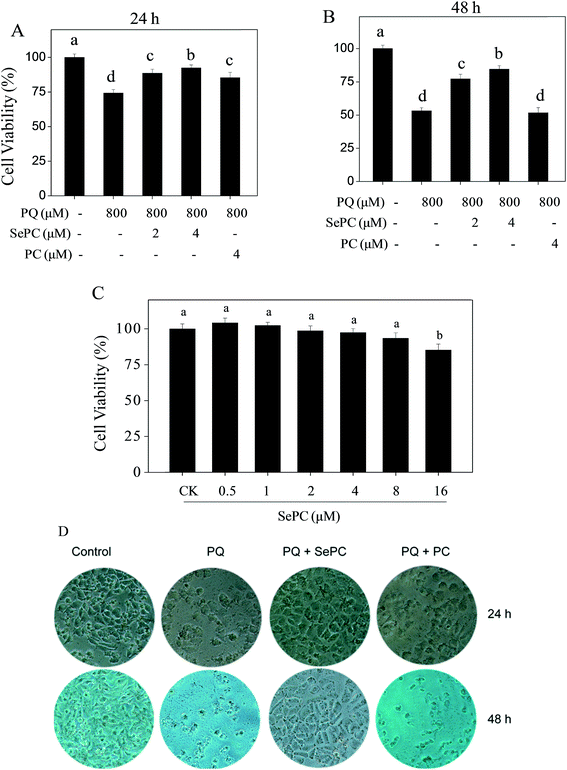 |
| Fig. 3 (A) Effect of phycocyanin (PC) and selenium–phycocyanin (Se–PC) on cell viability of HK2 cells after 24 h exposure to paraquat (PQ). (B) Effect of PC and Se–PC on cell viability of HK2 cells after 48 h exposure to PQ. (C) Effect of different concentrations of Se–PC on cell viability in PQ-induced HK2 cells. (D) Effect of PC and Se–PC on cell morphology of PQ-induced HK2 cells. Different letters indicate statistically significant differences (P < 0.05). | |
In order to confirm the protective effect of Se–PC, a phase-contrast microscopy was employed to observe the cell morphological changes. As shown in Fig. 3D, the exposure of HK-2 cells to 800 μM PQ for 24 and 48 h caused a significant drop of cell density and elimination of cell-to-cell contact. However, these changes caused by PQ treatment were significantly reversed by the combined treatment with 4 μM Se–PC. In contrast, PC treatment showed worse protective effect than that of Se–PC. Based on the above results, it suggested that Se incorporation into PC enhances its ability to suppress PQ-caused cytotoxicity in HK-2 cells.
3.5 The effects of PC and Se–PC on PQ-induced apoptosis in HK-2 cells
Induction of cell apoptosis by exotic insults is an effective way to inhibit cell proliferation.21,28 Caspase is fundamentally important for regulating cell apoptosis.29 Caspase-3 as the executor of apoptosis can inactivate PARP and initiate cell apoptosis.30 Accumulated evidences have indicated that cisplatin-induced nephrotoxicity involves activation of caspase-3 in renal tubule epithelial.31 Therefore, our results suggest that PQ-induced cytotoxicity against HK-2 cells was achieved by induction of apoptosis, as convinced by the activation of caspase-3.
As shown in Fig. 4A, the treatment of HK-2 cells with 800 μM PQ significantly activated the caspase-3 from 100% to 194.2%, indicating the existence of apoptosis in HK-2 cells. However, PQ-induced activation of caspase-3 was markedly suppressed by Se–PC co-treatment. For instance, treatment with 4 μM Se–PC dramatically decreased the caspase-3 activity from 194.2% (PQ) to 131.1% (Se–PC). Moreover, 4 μM Se–PC alone caused no change in caspase-3 activity. The results above clearly suggest that Se–PC inhibits PQ-induced apoptosis in HK-2 cells through the inactivation of caspase-3, and Se incorporation into PC enhances its ability in inhibition of PQ-induced HK-2 cell apoptosis.
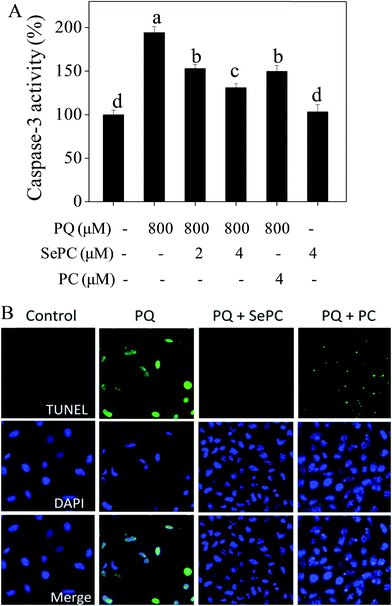 |
| Fig. 4 (A) Selenium–phycocyanin (Se–PC) inhibits paraquat (PQ) induced activation of caspase-3. (B) Se–PC reverses PQ-induced DNA fragmentation and nuclear condensation in HK-2 cells. | |
Apoptotic cells may undergo nuclear condensation and DNA fragmentation.22 DNA fragmentation is a critical biochemical hallmark of apoptotic cells. TUNEL enzymatic labeling can be employed to especially detect the DNA fragmentation in early stage of apoptotic cells.32 Fig. 4B showed that 800 μM PQ treatments caused evident DNA fragmentation of HK-2 cells, as convinced by the bright green fluorescence. However, PQ-induced DNA fragmentation was completely reversed by Se–PC co-treatment, as convinced by the decreased number of TUNEL-positive cells. This protective effect of Se–PC on HK-2 cells was further confirmed by DAPI staining. For instance, cells exposed to 800 μM PQ exhibited typical nuclear condensation. However, Se–PC co-treatment effectively blocked PQ-induced morphological alterations. Moreover, Se–PC showed better protective effect than that of PC. These results further confirmed that Se incorporation in PC reduces DNA fragmentation and nuclear condensation, which are indicators of apoptosis. These effects contribute to the general trend of preventing PQ-induced apoptosis.
3.6 Se–PC prevents PQ-induced superoxide overproduction in HK-2 cells
Reactive oxygen species (ROS), including hydroxyl radical and superoxide anion, bring out severe harm to intracellular proteins, membrane lipids and DNA which ultimately results in cell apoptosis.22,23 Emerging studies have reported that ROS production is related to several types of human pathological conditions, including cisplatin-induced nephrotoxicity.33,34 Therefore, intracellular superoxide anion level was examined by DHE fluorescent probe.
As shown in Fig. 5, PQ caused significant increase of superoxide anion level in HK-2 cells. For instance, treatment of cells with 800 μM PQ notably promoted the superoxide anion level to 260.4%. However, co-treatment of the HK-2 cells with 4 μM Se–PC significantly prevented the superoxide anion rate to 142.6%. The protective effect of PC at 4 μM is similar to that of Se–PC at 2 μM. However, the effect of Se–PC at 4 μM was the strongest achieved. Moreover, no significant change in superoxide anion level was detected after treatment with 4 μM Se–PC alone. Fluorescent images detected by microscope further confirmed the protective effects of Se–PC, as convinced by weaken red fluorescence (Fig. 5B). The results indicate that Se–PC prevents PQ-induced superoxide overproduction in HK-2 cells, and Se incorporation into PC enhances its antioxidant activity.
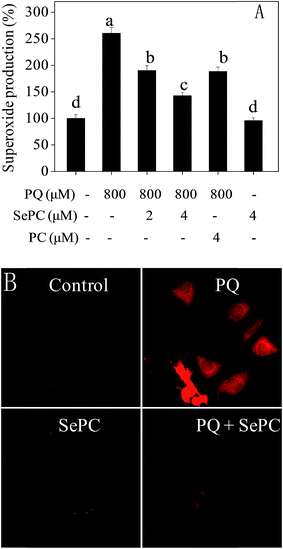 |
| Fig. 5 (A) Selenium–phycocyanin (Se–PC) blocks paraquat (PQ) induced superoxide overproduction in HK-2 cells. (B) Se–PC reduces PQ-induced intracellular superoxide anion level examined using DHE fluorescent probe. | |
In summary, a fast liquid chromatographic method for separation and purification of Se–PC from Se-enriched Nostoc sp. has been established. The purified Se–PC drastically prevented PQ-caused oxidative damage and nephrotoxicity in HK-2 proximal tubular cells. Mechanism investigation revealed that Se–PC blocked PQ-induced cell apoptosis through restraining the overproduction of superoxide. In conclusion, we suggest that Se–PC is a promising Se species for the advanced treatment of oxidative stress-mediated renal injury.
Conflicts of interest
There are no conflicts to declare.
Acknowledgements
This work was supported by the Natural Science Foundation of China (31000246), the Major State Basic Research Development Program (973) of China (No. 2011CB100400-G), YangFan Innovative & Entepreneurial Research Team Project (201312H05), Guangdong Special Support Program and Guangdong Frontier Key Technological Innovation Special Funds (2014B050505012), the Science and Technology Planning Project of Guangdong Province (2015A020209152, 2016A030303050) and Fundamental Research Funds for the Central Universities.
References
- R. J. Dinis-Oliveira, J. A. Duarte, A. Sánchez-Navarro, F. Remião, M. L. Bastos and F. Carvalho, Crit. Rev. Toxicol., 2008, 38, 13–71 CrossRef CAS PubMed.
- J. Chen, T. Zeng, Y. Bi, Z. Zhong, K. Xie and X. Zhao, Inhalation Toxicol., 2013, 25, 9–16 CrossRef CAS PubMed.
- F. Maya, J. M. Estela and V. Cerdà, Talanta, 2011, 85, 588–595 CrossRef CAS PubMed.
- M. R. Juchau, A. G. Fantel, C. Harris and B. K. Beyer, Environ. Health Perspect., 1986, 60, 131–136 CrossRef.
- Z. E. Suntres, Toxicology, 2002, 180, 65–77 CrossRef CAS PubMed.
- E. A. Awadalla, Exp. Toxicol. Pathol., 2012, 64, 431–434 CrossRef CAS PubMed.
- G. W. Burton, Proc. Nutr. Soc., 1994, 53, 251–262 CrossRef CAS PubMed.
- R. J. Reiter, Prog. Neurobiol., 1998, 56, 359–384 CrossRef CAS PubMed.
- N. K. Sharma, S. P. Tiwari, K. Tripathi and A. K. Rai, J. Appl. Phycol., 2011, 23, 1059–1081 CrossRef CAS.
- C. Halfmann, L. Gu and R. Zhou, Green Chem., 2014, 16, 3175–3185 RSC.
- D. Han, Y. Bi and Z. Hu, Handbook of microalgal culture: biotechnology and applied phycology, ed. A. Richmond, Oxford: Blackwell Science, 2004, pp. 304–311 Search PubMed.
- A. Ramos, F. Acién, J. M. Fernández-Sevilla, C. V. González and R. Bermejo, J. Chem. Technol. Biotechnol., 2010, 85, 783–792 CrossRef CAS.
- M. C. Santiago-Santos, T. Ponce-Noyola, R. Olvera-Ramírez, J. Ortega-López and R. O. Cañizares-Villanueva, Process Biochem., 2004, 39, 2047–2052 CrossRef CAS.
- A. A. Tchernov, K. M. Minkova and N. B. Houbavenska, J. Biotechnol., 1999, 69, 69–73 CrossRef CAS.
- Y. Sun, J. Zhang, Y. Yan, M. Chi, W. Chen, P. Sun and S. Qin, Environ. Toxicol. Pharmacol., 2011, 32, 168–174 CAS.
- R. Kaur and H. S. Sandhu, Environ. Toxicol. Pharmacol., 2008, 26, 45–48 CrossRef CAS PubMed.
- H. Zhang, T. Chen, J. Jiang, Y. S. Wong, F. Yang and W. Zheng, J. Agric. Food Chem., 2011, 59, 8683–8690 CrossRef CAS PubMed.
- Z. Huang, B. J. Guo, R. N. S. Wong and Y. Jiang, Food Chem., 2007, 100, 1137–1143 CrossRef CAS.
- J. Riss, K. Décordé, T. Sutra, M. Delage, J. C. Baccou, N. Jouy, J. P. Brune, H. Oréal, J. P. Cristol and J. M. Rouanet, J. Agric. Food Chem., 2007, 55, 7962–7967 CrossRef CAS PubMed.
- T. F. Chen, Y. S. Wong and W. Zheng, Phytochemistry, 2006, 67, 2424–2430 CrossRef CAS PubMed.
- T. F. Chen and Y. S. Wong, J. Agric. Food Chem., 2008, 56, 4352–4358 CrossRef CAS PubMed.
- C. Fan, J. Chen, Y. Wang, Y. S. Wong, Y. Zhang, W. Zheng, W. Cao and T. Chen, Free Radical Biol. Med., 2013, 65, 305–316 CrossRef CAS PubMed.
- T. F. Chen and Y. S. Wong, Biomed. Pharmacother., 2009, 63, 105–113 CrossRef CAS PubMed.
- B. Soni, B. Kalavadia, U. Trivedi and D. Madamwar, Process Biochem., 2006, 41, 2017–2023 CrossRef CAS.
- B. Soni, U. Trivedi and D. Madamwar, Bioresour. Technol., 2008, 99, 188–194 CrossRef CAS PubMed.
- S. G. Yan, L. P. Zhu, H. N. Su, X. Y. Zhang, X. L. Chen, B. C. Zhou and Y. Z. Zhang, J. Appl. Phycol., 2011, 23, 1–6 CrossRef CAS.
- C. Fan, J. Jiang, X. Yin, K. H. Wong, W. Zheng and T. Chen, Food Chem., 2012, 134, 253–261 CrossRef CAS.
- T. F. Chen and Y. S. Wong, J. Agric. Food Chem., 2008, 56, 10574–10581 CrossRef CAS PubMed.
- S. J. Riedl and Y. Shi, Nat. Rev. Mol. Cell Biol., 2004, 5, 897–907 CrossRef CAS PubMed.
- K. S. Putt, G. W. Chen, J. M. Pearson, J. S. Sandhorst, M. S. Hoagland, J. T. Kwon, S. K. Hwang, H. Jin, M. I. Churchwell and M. H. Cho, Nat. Chem. Biol., 2006, 2, 543–550 CrossRef CAS PubMed.
- R. Cetin, E. Devrim, B. Kilicoglu, A. Avci, O. Candir and I. Durak, J. Appl. Toxicol., 2006, 26, 42–46 CrossRef CAS PubMed.
- Y. Li, X. Li, Y. S. Wong, T. Chen, H. Zhang, C. Liu and W. Zheng, Biomaterials, 2011, 32, 9068–9076 CrossRef CAS PubMed.
- L. Ferder, F. Inserra and M. Martinez-Maldonado, Curr. Hypertens. Rep., 2006, 8, 191–198 CrossRef CAS PubMed.
- M. J. Sung, D. H. Kim, Y. J. Jung, K. P. Kang, A. S. Lee, S. Lee, W. Kim, M. Davaatseren, J. T. Hwang and H. J. Kim, Kidney Int., 2008, 74, 1538–1547 CrossRef CAS PubMed.
|
This journal is © The Royal Society of Chemistry 2017 |