DOI:
10.1039/C7RA07893E
(Paper)
RSC Adv., 2017,
7, 39955-39961
Mercapto functionalized sepiolite: a novel and efficient immobilization agent for cadmium polluted soil
Received
18th July 2017
, Accepted 10th August 2017
First published on 16th August 2017
Abstract
Immobilization agents are the key factor that determine the success of immobilization remediation in heavy metal polluted soil. In this study, mercapto functionalized sepiolite (MSEP) as a novel and efficient immobilization agent was prepared through surface modification and utilized for the remediation of cadmium (Cd)-polluted paddy soil in pot trials. MSEP at trace dosages of 0.1–0.3% could reduce the Cd content of husked rice (Oryza sativa L.) by 65.4–77.9%; this was more efficient than the traditional pH regulating amendments such as clay minerals. Single and sequential procedures demonstrated that MSEP could enhance the fixation or sorption of Cd on soil compositions and reduce the bioavailability of Cd. MSEP had negligible effects on the pH values and the point of zero charge (PZC) of paddy soil, and had no obvious impact on extractable zinc, hydrolyzable nitrogen and available phosphorus in the soil, indicating that it is environmental friendly and compatible. Physiologically, MSEP could increase total antioxidant capacity (T-AOC) and nonprotein thiol (NPT) content and reduce the malondialdehyde (MDA) content of rice root so that MSEP could alleviate the stress of Cd in rice. In general, compared to natural sepiolite as a traditional pH-regulating amendment in the present study, the primary immobilization mechanism and environmental compatibility and friendliness of MSEP are its great advantages. The trace application dosages could save economic costs and facilitate easier large-area application. Thus, we recommend MSEP as a novel and efficient immobilization agent for the remediation for Cd-polluted paddy soil.
1 Introduction
Cadmium (Cd) has been the most frequently detected heavy metal in paddy fields, and a recent nationwide survey report conducted by the Ministry of Environmental Protection and Ministry of Lands and Resources of China revealed that 7% of the investigated sites are contaminated by Cd.1 Rice (Oryza sativa L.) is a crop at high risk of Cd uptake and the accumulation of Cd in rice grain and its subsequent transfer into the food chain is a global environmental issue.2,3 The remediation of Cd-polluted paddy soils is an urgent task for ecological safety and human health.
Among all the remediation methods for heavy metals in agricultural soil, in situ immobilization is cost-effective and environmentally compatible. Immobilization reduces the bioavailability by amendments or immobilization agents.4 The immobilization agent is the key factor that determines the success of the immobilization remediation. Traditional pH regulating agents such as limestone and lime,5 and the adsorbent materials, including sepiolite and palygorskite,6 and biochar7 showed excellent performance in the remediation of Cd from polluted paddy soil. However, the application dosages were huge. For example, palygorskite and sepiolite at dosages of 1.5 and 2.25 kg m−2 were recommended as the immobilization agents for Cd-polluted paddy soil to ensure the safe production of different rice cultivars.6 Biochar produced from wheat straw at the maximum pyrolysis temperature 350–550 °C at a dosage of 3% and 5% (by weight) consistently reduced the rice Cd and lead (Pb) contents in three years.7 The long-term addition of clay minerals in huge dosages would have adverse effects on soil environmental quality and lead to soil compaction. Enhancing the remediation effects and reducing the application dosages to save economic costs are urgently needed for remediation practices.8
In the present study, mercapto functionalized sepiolite (MSEP) was prepared as a novel and efficient immobilization agent to immobilize Cd in paddy soil and alleviate Cd accumulation in rice grain in pot trials. MSEP could remove heavy metals in aqueous solution.9 For example, MSEP could uptake Pb2+ through the mechanisms of chemical adsorption and physical adsorption. However, the application for the remediation of heavy metal polluted soil has not been confirmed to date. The aim of this study was to illustrate the remediation effects of MSEP on Cd pollutant in paddy soil to provide a theoretical basis and practical guide for in situ field scale remediation.
2 Materials and methods
2.1 Preparation of mercapto functionalized sepiolite
MSEP was prepared by nano-texturization in aqueous gel.9 Pristine sepiolite (SEP) with the composition of Mg4Si6O15(OH)2·6H2O (JCPDS card no. 13-0595) was obtained from the Tolsa of Spain and 3-mercaptopropyltrimethoxysilane was purchased from Sigma-Aldrich. All reagents were used as received without any purification. The sulfur content of MSEP determined quantitatively via elemental analyses in a CNHS analyzer was 2.53 mmol g−1.
2.2 Experimental design
The soil samples were collected from 20 cm topsoil of a contaminated paddy field in Chenzhou, Hunan province. The soil was a paddy soil derived from the shale weathering. It was severely polluted with cadmium due to historical lead-zinc smelting and mining. The basic physiochemical properties of the soil were as follows: pH 6.8 ± 0.1; cation exchange capacity 12.3 ± 2.1 cmol kg−1, organic matter 5.3 ± 0.2% and total Cd amount 1.06 ± 0.08 mg kg−1.
The pot experiment was conducted in a greenhouse. Soil samples of 10.0 kg were passed through 4 mm mesh and placed in a plastic pot. The non-amended soil was used as the control (CK). SEP and MSEP were added as immobilization agents to the polluted soil. Six treatments were designed that included SEP 1 g kg−1 (Sep-0.1%), 2 g kg−1 (Sep-0.2%) and 3 g kg−1 (Sep-0.3%); MSEP 1 g kg−1 (MSEP-0.1%), 2 g kg−1 (MSEP-0.2%) and 3 g kg−1 (MSEP-0.3%). All treatments were performed in triplicates. The hybrid cultivar of O. sativa L. subsp. hsien Ting was Xinrong Youhuazhan with the whole growth period of 123 d.
2.3 Analytical methods
The pH of soil was measured at a soil
:
water ratio of 1
:
2.5 (w/v) using a pH meter (PB-10, Sartorius, Germany). The point of zero charge (PZC) of the soil sample was determined by potentiometric titration.10 Air-dried soil samples of 5 g were placed in the titrating vessel of automatic potentiometric titrator (ZD-3A, Shanghai Anting) along with 50 mL of NaNO3 solutions (0.1, 0.05 and 0.005 M mol L−1). The sample was stirred for 2 min for pre-equilibration, then continuously stirred and titrated at a certain interval with 0.02 mol L−1 HCl or NaOH solutions made up in the ionic strength controlling solution. The PZC was calculated through the curve of (ΔH–ΔOH) versus pH. The soil samples were digested using HNO3–HF–HClO4 (2
:
2
:
1, v/v/v) solution at a 1
:
25 soil/liquid ratio to determine the total Cd content. Air-dried soil samples (5.0 g) were placed in 50 mL centrifuge tubes and dispersed into 25 mL of 0.025 mol L−1 HCl solutions11 and 1.0 mol L−1 NH4OAc solution, respectively. After shaking for 120 min, the samples were centrifuged at 4300 rpm and the supernatants were collected for the analyses of the plant accessible Cd concentrations in paddy soil. Meanwhile, the sequential extraction procedure followed was: 1.0 mol L−1 MgCl2 for exchangeable fraction (SE), 1.0 mol L−1 NaOAc extraction for carbonate-bound fraction (WSA), 0.04 mol L−1 NH2OH·HCl for Fe–Mn oxide fraction (OX), 30% H2O2/3.2 mol L−1 NH4OAc for organic fraction (OM) and residual fraction (RES).12 The available nitrogen content was determined using alkali-hydrolyzed reduction diffusing method13 and the available phosphorus was measured using an acid-extracted molybdenum colorimetric method with HCl–NH4F digestion.14 The available Cu and Zn concentrations were estimated through diethylenetriamine pentaacetic acid (DTPA) solution extraction.15
A 0.50 g sample of husked rice powder was digested using a 10 mL mixed solution of HNO3–HClO4 (4
:
1, v/v). The Cd concentrations in the soil extract and the digest solutions were detected using inductively coupled plasma mass spectrometer (iCAP Q, Thermo Scientific, U.S).
The protein content of the rice root was determined using Coomassie brilliant blue method. Approximately 1.0 g of rice root was homogenized in 9 mL 0.9% normal saline and used for protein estimation. The contents of malondialdehyde (MDA), non-protein thiols (NPT) and total antioxidant capacity (T-AOC) of rice root were determined by plant malondialdehyde assay kit (530 nm microplate reader colorimetric method), total thiol assay kit (450 nm microplate reader colorimetric method) and the total antioxidant capacity assay kit (520 nm visible spectrophotometer colorimetric method), respectively as developed by the Nanjing Jiancheng Bioengineering Institute.
2.4 Statistical analysis
All data were statistically analysed using one-way ANOVA at a significance level of p < 0.05 with SPSS 22.0. Single-step multiple comparisons of means were performed via Tukey's post hoc test.
3 Results and discussion
3.1 Effects of MSEP and SEP on Cd accumulation in husked rice
As shown in Fig. 1, the average Cd content of husked rice in CK was 0.26 mg kg−1, which exceeded the maximum level of the National Standard of China GB 2762-2012 “Maximum Levels of Contaminants in Foods” (0.2 mg kg−1). The Cd contents of husked rice were reduced slightly by SEP, but still exceeded the maximum permitted level and no statistical differences were observed between CK and SEP treatments at the three dosages (p > 0.05). However, MSEP significantly reduced the Cd contents of husked rice by 65.4–77.9% compared to the CK. The least value was 0.05 mg kg−1 even at the dosage of 1 g kg−1. The concentration of heavy metals in grains is a critical factor to be assess with regard to the success of remediation methods. Although the contents of Cd in husked rice was not proportional to the dosage of MSEP, the decrease in the accumulation of Cd in rice grain confirmed the immobilization effect of MSEP on Cd pollutant in paddy soil.
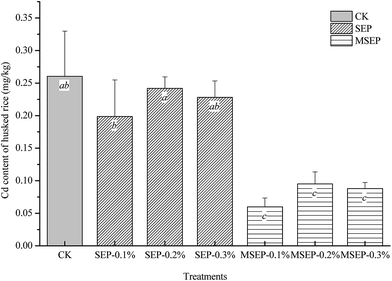 |
| Fig. 1 Effect of MSEP and SEP on Cd content of husked rice.* The same letters within the individual error bars are not significantly different (p > 0.05); those with different letters are significantly different (p < 0.05) (n = 6). | |
In previous studies on remediation of Cd-polluted paddy soil listed in Table 1, most of the immobilization agents used were pH regulating amendments such as limestone and clay minerals. The application dosages of these immobilization agents in field implementation and pot experiments were much higher than the dosages of MSEP in the current study. For example, natural sepiolite at a dosage of 0.5–1.0 g kg−1 was recommended for the remediation of Cd-polluted acid paddy soil,6,16,17 which was 10 times of the current dosage of MSEP. The high performance at trace dosage is one of the advantages of MSEP. Although the surface modification process increased the cost of MSEP, compared to SEP, the immobilization efficiency was enhanced and the application dosages were reduced to about 10% of pristine sepiolite. Further, less application dosage would save the transportation and labor cost in the large-area field-scale utilization in the future. The total application cost of MSEP was less than that of SEP.
Table 1 Contradistinction of immobilization agents for Cd-polluted soil
Immobilization agents |
Dosagea |
Scale |
Cd in brown rice (mg kg−1) |
Reference |
The dosage in field experiments (kg m−2) can be transferred into the percentage by weight (%) based on the soil density and area. For example, 2.25 kg m−2 was about 15 g kg−1. |
Sepiolite |
8 g kg−1 |
Pot |
0.44 → 0.29 |
27 |
Sepiolite |
2.25 kg m−2 |
Field |
0.72 → 0.18 |
6 |
Sepiolite |
2.25 kg m−2 |
Field |
0.5 → 0.4 |
28 |
Limestone + sepiolite |
8 g kg−1 |
Field |
2.6 → 2.0 |
20 |
Bentonite |
24 g kg−1 |
Pot |
0.44 → 0.14 |
27 |
Biochar from wheat straw |
2 kg m−2 |
Field |
3.1 → 0.7 |
29 |
Biochar from farm residuals |
40 g kg−1 |
Pot |
1.44 → 1.03 |
30 |
Biochar from wheat straw |
4 kg m−2 |
Field |
3.15 → 1.73 |
31 |
Biochar from cotton straw |
10.0 g kg−1 |
Pot |
0.29 → 0.19 |
32 |
Hydroxyhistidine + zeolite |
8 g kg−1 |
Field |
2.6 → 2.1 |
20 |
MSEP |
1 g kg−1 |
Pot |
0.26 → 0.06 |
Current study |
3.2 Effects of MSEP and SEP on chemical fraction of Cd in paddy soil
MSEP as novel immobilization agent was designed to reduce the bioavailability of heavy metals in soil but not the total concentration. The plant accessible fraction was reduced, which is much important in decreasing environmental risks. The changes in mobile Cd fraction as affected by the treatments are presented in Fig. 2. The HCl and NH4OAC solutions were usually employed to determine the available fraction of heavy metals in soil due to the good correlations between their extraction concentrations and the uptake of Cd by crops.18 The HCl extractable Cd content showed a significant positive correlation with Cd content of husked rice6 and has been recommended as extraction solution for bioavailability of heavy metals in soil in Japan.19 However, in this experiment, no statistically significant differences were observed among CK and SEP treatments in the extractable Cd contents (p > 0.05). The recommended application dosage of natural sepiolite for the remediation of Cd-polluted acid paddy soil was 10 g kg−1.6 This shows that SEP as traditional immobilization agents has significant remediation effect only at high application dosage, and the immobilization effect at trace amount of dosage can be ignored. MSEP at a dosage of 1 g kg−1 had remarkable immobilization effect suggesting that the high performance of MSEP in the aspect of chemical extractable bioavailability.
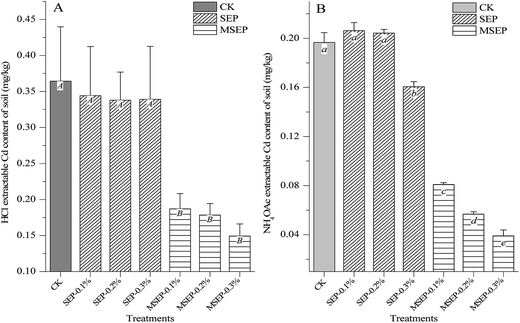 |
| Fig. 2 Changes in chemical extractable Cd contents in paddy soil. (A) HCl extractable Cd content of soil; (B) NH4OAc extractable Cd content of soil. | |
The sequential extractable fraction of Cd in soils can reflect the effect of MSEP on the chemical fractions. As shown in Fig. 3, the exchangeable Cd, residual Cd, and Fe/Mn oxide-bound Cd have dominated fractions for CK. SEP treatments at three different dosages had no effect on exchangeable Cd, but MSEP could reduce SE-Cd, which led to a reduction in Cd by 42.8–79.6% compared to CK (p < 0.05). The exchangeable fractions decreased with the increase of MSEP dosage from 0.1–0.3%. It also reduced the carbonate-bound fractions remarkably compared to CK. The concentrations of exchangeable and carbonate-bound fractions, which can be absorbed by plants reduced after the addition of MSEP. It indicated the high performance of the immobilization effect in the species distribution. Meanwhile, MSEP resulted in an increase in Fe/Mn oxide-bound Cd content by about 120% and the organic matter-bound Cd content by 75.1–99.8%. The increase in Fe/Mn oxide-bound Cd fraction is an interesting phenomenon, as it did not introduce new minerals containing Fe/Mn oxides. This can be attributed to enhanced sorption of Cd on soil after the addition of MSEP. The selected paddy soil contained high amounts of Fe/Mn oxides, which had the potential of fixation or sorption of heavy metal cations in soil solutions. The addition of MSEP enhances the sorption of Cd on Fe/Mn oxides, which can be regarded as the indirect impact of MSEP on the chemical fractions. Further studies are required to elucidate the underlying mechanisms. The slight increase of organic matter-bound fractions can be ascribed to the mercapto functional group. The increase of exchangeable Cd, Fe/Mn oxides, and organic matter-bound Cd and the decrease in carbonate-bound Cd led to the increase of residual Cd. The traditional pH regulating immobilization agents, such as lime and limestone,20 shift the exchangeable Cd into carbonate-bound Cd and do not affect the Fe/Mn oxides and organic matter bound fractions. The carbonate-bound fraction has the potential risk of releasing into the soil again when environmental conditions change. MSEP shifted exchangeable and carbonate bound fractions to Fe/Mn oxide- and organic matter-bound and residual fractions, which would be stable in the long term. The immobilization effect of MSEP on Cd and Cu can be stimulated by the sorption mechanism in aqueous solutions. For MSEP, the complexation of Cd2+ with mercapto groups existed in addition to the complexation with surface hydroxyl groups.21,22
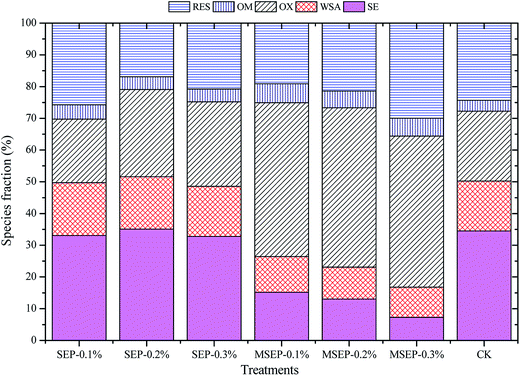 |
| Fig. 3 Effects of MSEP and SEP on species distribution of Cd in paddy soil. | |
3.3 Effects of MSEP and SEP on soil environmental quality
The average pH value of soil in CK was 6.8 (Fig. 4A), indicating that it is a neutral soil. Neither SEP nor MSEP had remarkable effects on the soil pH (p > 0.05) due to the pH-buffering effect of soils. The PZC determined in NaNO3 solutions was about 7.2 (Fig. 4B). The MSEP and SEP in the present study had negligible effects on PZC of the paddy soil. Further, the pH values of the paddy soil were less than PZC, indicating that the surface of soil particles was of positive charge, which inhibited the sorption of metal cations on the soil particles. In the previous studies, increased pH has been considered the main factor for the decrease in bioavailability of heavy metals.4 For example, natural minerals containing CaCO3 increased the pH value of paddy soils and led to a remarkable reduction in extractable Cd contents and an increase of carbonate-bound fraction.6 High application dosage of pH regulating immobilization agents in the long term would lead to soil compaction. MSEP at trace dosage reduces the risk of adverse impact. MSEP had negligible effects on the pH and PZC, indicating soil compatibility and environmental friendliness.
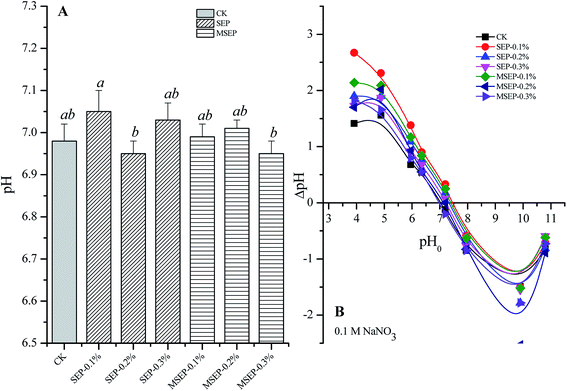 |
| Fig. 4 Effects of MSEP and SEP on pH and PZC of paddy soil. (A) pH value of paddy soil; (B) PZC of paddy soil. | |
The Cu and Zn are essential elements for plant growth, but once they exceed the normal range, there will be heavy metal pollutions. Both SEP and MSEP reduced the DTPA extractable Cu in paddy soil (Fig. 5), however, MSEP had negligible effects on DTPA extractable Zn. Available nitrogen and phosphorus are critical for the normal growth of the plant. The addition of SEP and MSEP had no obvious impacts on the available nitrogen and phosphorus contents of the paddy soil (Fig. 5), which could be attributed to the negligible impacts of MSEP on pH value. The pH of the soil is an important factor that significantly affects the available nitrogen and phosphorus contents in paddy soil. Natural sepiolite increased soil pH remarkably and had the risk to reduce the available phosphorus contents, thus was recommended in combination with phosphate fertilizers in remediation practice.23 Compared to that the natural sepiolite, MSEP has no adverse impact on the available nutrients and is thus environmentally friendly.
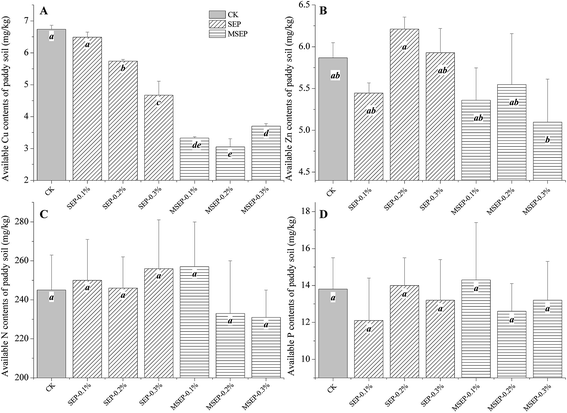 |
| Fig. 5 Available contents of Cu, Zn, N and P in paddy soil. (A) DTPA extractable Cu content of soil; (B) DTPA extractable Zn content of soil; (C) available N content of soil; (D) available P content of soil. | |
3.4 Effects of MSEP and SEP on physiological indicators of rice root
MDA is the final product of peroxidation of membrane lipids and is usually employed as an indicator of lipid peroxidation under various stresses, including heavy metal stress.24,25 The addition of amendments could decrease the MDA contents of rice root by 27.76–32.40% and 55.48–58.69% for SEP and MSEP, respectively. These results indicate that the oxidative stresses of Cd on rice root were alleviated. Meanwhile, T-AOC of rice root increased by 13.92–25.04% and 23.41–32.91% for SEP and MSEP, respectively (Fig. 6B). The elevated total antioxidant capacity along with reduced MDA revealed the remediation effect of MSEP on Cd pollutant in paddy soil. Non-protein thiol, as an important antioxidant in mitigating Cd-induced oxidative stress plays an important role in phytochelatins synthesis, which has a proven role in Cd detoxification.26 NPT content in rice root after the addition of MSEP increased significantly compared to CK (Fig. 6C). Further studies are required to elucidate the changes and mechanisms of MSEP action.
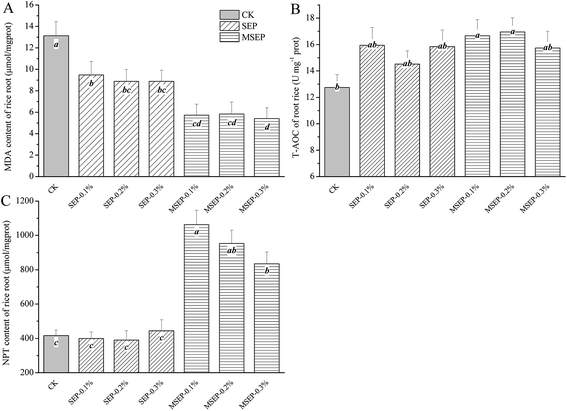 |
| Fig. 6 Dynamics of MDA, T-AOC and NPT of rice root under different treatments. (A) MDA content of rice root; (B) T-AOC of rice root; (C) NPT content of rice root. | |
4 Conclusion
MSEP at trace dosages of 0.1–0.3% could reduce the Cd contents of husked rice by 65.4–77.9%. It could enhance the fixation or sorption of Cd on soil compositions and reduce its bioavailability. It could also increase the total antioxidant capacity and nonprotein thiols contents, and reduce the MDA content of rice root to alleviate the stress of Cd in rice. Thus, MSEP as a novel and efficient immobilization agent can be recommended for the remediation of Cd-polluted paddy soil.
Conflicts of interest
There are no conflicts to declare.
Acknowledgements
This research was supported by the Central Public Research Institutes Basic Funds for Research and Development (2016-szjj-wrxf-lxf), National Natural Science Foundation of China (No. 41401362) and the Funds for Science and Technology Innovation Project from the Chinese Academy of Agricultural Sciences (No. CAAS-XTCX-2016018).
References
- F.-J. Zhao, Y. Ma, Y.-G. Zhu, Z. Tang and S. P. McGrath, Environ. Sci. Technol., 2015, 49, 750–759 CrossRef CAS PubMed.
- M. Rizwan, S. Ali, M. Adrees, H. Rizvi, M. Zia-ur-Rehman, F. Hannan, M. F. Qayyum, F. Hafeez and Y. S. Ok, Environ. Sci. Pollut. Res., 2016, 23, 17859–17879 CrossRef CAS PubMed.
- M. Rizwan, S. Ali, M. Adrees, H. Rizvi, M. Zia-ur-Rehman, F. Hannan, M. F. Qayyum, F. Hafeez and Y. S. Ok, Environ. Sci. Pollut. Res., 2016, 1–21, DOI:10.1007/s11356-016-6436-4.
- N. Bolan, A. Kunhikrishnan, R. Thangarajan, J. Kumpiene, J. Park, T. Makino, M. B. Kirkham and K. Scheckel, J. Hazard. Mater., 2014, 266, 141–166 CrossRef CAS PubMed.
- S.-C. Kim, H. S. Kim, B.-H. Seo, G. Owens and K.-R. Kim, Geoderma, 2016, 270, 83–88 CrossRef CAS.
- X. Liang, J. Han, Y. Xu, Y. Sun, L. Wang and X. Tan, Geoderma, 2014, 235–236, 9–18 CrossRef CAS.
- R. Bian, S. Joseph, L. Cui, G. Pan, L. Li, X. Liu, A. Zhang, H. Rutlidge, S. Wong, C. Chia, C. Marjo, B. Gong, P. Munroe and S. Donne, J. Hazard. Mater., 2014, 272, 121–128 CrossRef CAS PubMed.
- N. S. Bolan, T. Makino, A. Kunhikrishnan, P.-J. Kim, S. Ishikawa, M. Murakami, R. Naidu and M. B. Kirkham, in Advances in Agronomy, ed. L. S. Donald, Academic Press, Amsterdam, 2013, vol. 119, pp. 183–273 Search PubMed.
- X. Liang, Y. Xu, G. Sun, L. Wang, Y. Sun, Y. Sun and X. Qin, Chem. Eng. J., 2011, 174, 436–444 CrossRef CAS.
- L. W. Zelazny, L. He and A. Vanwormhoudt, in Methods of Soil Analysis Part 3 Chemical Methods, ed. J. M. Bigham, Soil Science Society of America, American Society of Agronomy, Madison, Wisconsin, 1996, pp. 1231–1254 Search PubMed.
- T. Ibaraki, K. Kadoshige and M. Murakami, Soil Sci. Plant Nutr., 2005, 51, 893–898 CrossRef CAS.
- A. Tessier, P. G. C. Campbell and M. Bisson, Anal. Chem., 1979, 51, 844–851 CrossRef CAS.
- N. Bélanger, D. Paré and W. H. Hendershot, in Soil Sampling and Methods of Analysis, ed. M. R. Carter and E. G. Gregorich, Canadian Society of Soil Science, CRC Press, Boca Raton, FL, 2006, pp. 317–330 Search PubMed.
- C. Peng, S. Lai, X. Luo, J. Lu, Q. Huang and W. Chen, Sci. Total Environ., 2016, 557–558, 231–239 CrossRef CAS PubMed.
- M. Pansu and J. Gautheyrou, Handbook of Soil Analysis Mineralogical, Organic and Inorganic Methods, Springer-Verlag, Berlin Heidelberg, 2006 Search PubMed.
- Y. Sun, G. Sun, Y. Xu, L. Wang, D. Lin, X. Liang and X. Shi, J. Environ. Sci., 2012, 24, 1799–1805 CrossRef CAS.
- Y. Sun, G. Sun, Y. Xu, L. Wang, X. Liang and D. Lin, Geoderma, 2013, 193–194, 149–155 CrossRef CAS.
- E. Meers, G. Du Laing, V. Unamuno, A. Ruttens, J. Vangronsveld, F. M. G. Tack and M. G. Verloo, Geoderma, 2007, 141, 247–259 CrossRef CAS.
- T. Kikuchi, M. Okazaki, S. D. Kimura, T. Motobayashi, J. Baasansuren, T. Hattori and T. Abe, J. Hazard. Mater., 2008, 154, 294–299 CrossRef CAS PubMed.
- H. Zhou, X. Zhou, M. Zeng, B.-H. Liao, L. Liu, W.-T. Yang, Y.-M. Wu, Q.-Y. Qiu and Y.-J. Wang, Ecotoxicol. Environ. Saf., 2014, 101, 226–232 CrossRef CAS PubMed.
- X. Liang, J. Han, Y. Xu, L. Wang, Y. Sun and X. Tan, Appl. Surf. Sci., 2014, 322, 194–201 CrossRef CAS.
- J. Han, X. Liang, Y. Xu and Y. Xu, J. Ind. Eng. Chem., 2015, 23, 307–315 CrossRef CAS.
- J. Han, X. Liang, Y. Xu, Y. Xu, Y. Lei and R. Jiang, Acta Sci. Circumstantiae, 2014, 34, 2853–2860 CAS.
- K. Shah, R. G. Kumar, S. Verma and R. S. Dubey, Plant Sci., 2001, 161, 1135–1144 CrossRef CAS.
- M. J. Hassan, G. Shao and G. Zhang, J. Plant Nutr., 2005, 28, 1259–1270 CrossRef CAS.
- S. S. Gill and N. Tuteja, Plant Signaling Behav., 2011, 6, 215–222 CrossRef CAS.
- Y. Sun, G. Sun, Y. Xu, W. Liu, X. Liang and L. Wang, J. Environ. Manage., 2016, 166, 204–210 CrossRef CAS PubMed.
- Q.-H. Zhu, D.-Y. Huang, G.-X. Zhu, T.-D. Ge, G.-S. Liu, H.-H. Zhu, S.-L. Liu and X.-N. Zhang, Acta Agric. Scand., Sect. B, 2010, 60, 110–116 CAS.
- R. Bian, D. Chen, X. Liu, L. Cui, L. Li, G. Pan, D. Xie, J. Zheng, X. Zhang, J. Zheng and A. Chang, Ecol. Eng., 2013, 58, 378–383 CrossRef.
- T. He, J. Meng, W. Chen, Z. Liu, T. Cao, X. Cheng, Y. Huang and X. Yang, BioResources, 2017, 12, 622–642 CAS.
- L. Cui, L. Li, A. Zhang, G. Pan, D. Bao and A. Chang, BioResources, 2011, 6, 2605–2618 CAS.
- J. Li and Y. Xu, Environ. Sci. Pollut. Res., 2015, 22, 5580–5586 CrossRef CAS PubMed.
|
This journal is © The Royal Society of Chemistry 2017 |
Click here to see how this site uses Cookies. View our privacy policy here.