DOI:
10.1039/C7RA06904A
(Paper)
RSC Adv., 2017,
7, 39758-39761
Cs2CO3-promoted cross-dehydrogenative coupling of thiophenols with active methylene compounds†
Received
20th June 2017
, Accepted 8th August 2017
First published on 15th August 2017
Abstract
A convenient and efficient α-sulfenylation of carbonyl compounds has been achieved via the halogen-free Cs2CO3-promoted cross-dehydrogenative coupling (CDC) of thiophenols with active methylene compounds using air as the oxidant under mild conditions. This transformation provides a straightforward route to the construction of carbon–sulfur bonds with wide functional group compatibility, which produces α-sulfenylated carbonyl compounds in up to 95% yield.
The development of new methods to construct carbon–sulfur bonds has been of particular interest due to the wide applications of organosulfur compounds in biological chemistry and organic synthesis.1,2 Among them, α-sulfenylated carbonyl compounds are important intermediates for the synthesis of heterocycles,3 β-keto sulfones,4 α,β-unsaturated carbonyl compounds,5 and others.6 Therefore, α-sulfenylation of carbonyl compounds is highly desirable, and a variety of useful synthetic methods have been well documented. The traditional preparation of this class of compounds mainly relies on the use of pre-functionalized substrates including: (1) nucleophilic substitution of α-halogenated carbonyl compounds with thiols7 (Fig. 1a) or disulfides8 (Fig. 1b); and (2) the reactions of carbonyl compounds with thio sources such as sulphenyl halides, disulfides, sulfonothioates, sulfenamides, and N-(phenylthio)succinimide (Fig. 1c).9 However, these methods are limited because the corresponding starting materials are high-cost and/or temperature- or moisture-sensitive. Thus, the development of a convenient and efficient protocol for the synthesis of α-sulfenylated carbonyl compounds remains a challenge.
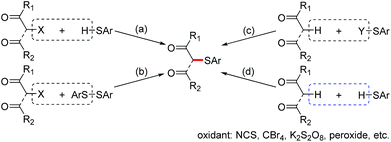 |
| Fig. 1 Strategies for α-sulfenylation of carbonyl compounds. | |
The cross-dehydrogenative coupling (CDC) reactions are powerful methods in organic synthesis that can avoid the use of pre-functionalized substrates.10 The CDC reactions involving thiols have attracted much attention because this strategy represents more straightforward, efficient, and atom-economic to construct carbon–sulfur and sulfur–heteroatom bonds.11 The oxidative CDC has also been applied to α-sulfenylation of carbonyl compounds (Fig. 1d).12 The coupling of thiols with active methylene compounds in the presence of CBr4 has been reported by Liang and co-workers.12a Yadav and co-workers have reported α-sulfenylation of monoketones in the presence of NCS.12b Recently, Hu, Lei and co-workers developed iodine-catalyzed oxidative coupling of 1,3-diketones with thiophenols using DTPB as the oxidant.12c Prabhu and co-workers developed a couple of α-sulfenylations of monoketones or 1,3-diketones using K2S2O8 or DMSO (in the presence of I2) as the oxidant.12d–f However, the current oxidative coupling protocols requires the use of halogenated reagents and/or strong oxidants. In this regard, seeking greener oxidants for this CDC reaction is still a significant issue. Molecular oxygen as the greener and more sustainable oxidant has been widely used in organic synthesis.13 Moreover, inorganic bases have been well utilized for carbon–sulfur and sulfur–heteroatom bond forming reactions.14 With these backgrounds, we envisioned that α-sulfenylated carbonyl compounds might be formed through the CDC reaction of thiols with carbonyl compounds using O2 as the oxidant in the presence of an inorganic base. Herein, we report an efficient halogen-free Cs2CO3-promoted α-sulfenylation of active methylene compounds under air.
The reaction conditions were tested by using a model reaction of acetylacetone 1a with 4-bromo-thiophenol 2a in solvents under air atmosphere at room temperature, and the results were shown in Table 1. Initially, no reaction occurred when the reaction of 1a with 2a in CH3CN in the absence of bases under air was carried out (entry 1). To our delight, when Cs2CO3 (1 equiv.) was added, the reaction proceeded smoothly to afford the desired product 3-(4-bromophenylthio)pentane-2,4-dione 3aa in 82% yield (entry 2). When the reaction of 1a with 2a was carried out under N2, only trace amounts of 3aa were detected (entry 3). This result demonstrates that the reaction involved an aerobic oxidative cross-coupling. We then turned to screen other bases (entries 4–9), and found that Cs2CO3 was the optimal base. The increase or decrease of Cs2CO3 amount did not improve the yield (entries 10–14). Notably, the use of catalytic amounts of Cs2CO3 also led to the formation of 3aa in moderate yields (entries 13 and 14). Switching the solvent from CH3CN to THF, dioxane, DMSO, EtOH, or H2O decreased the yield of 3aa (entries 15–19), while the use of DMF afforded the desired product in 98% yield (entry 20). It is noteworthy that disulfide 4a, which was generated via an aerobic oxidative homocoupling of thiol 2a,14a was observed in all cases under air.
Table 1 Optimization of reaction conditionsa,b

|
Entry |
Base (equiv.) |
Solvent |
Yield of 3aa (%) |
Reaction conditions: 1a (0.2 mmol), 2a (0.4 mmol), base, solvent (1 mL), room temperature, open air, 6 h. Yield based on 1a was determined by 1H NMR analysis of crude products using an internal standard. The reaction was carried out under N2. |
1 |
|
CH3CN |
0 |
2 |
Cs2CO3 (1) |
CH3CN |
82 |
3c |
Cs2CO3 (1) |
CH3CN |
Trace |
4 |
K2CO3 (1) |
CH3CN |
65 |
5 |
Na2CO3 (1) |
CH3CN |
0 |
6 |
NaOAc (1) |
CH3CN |
0 |
7 |
K3PO4 (1) |
CH3CN |
45 |
8 |
CsF (1) |
CH3CN |
16 |
9 |
Et3N (1) |
CH3CN |
<10 |
10 |
Cs2CO3 (3) |
CH3CN |
74 |
11 |
Cs2CO3 (2) |
CH3CN |
82 |
12 |
Cs2CO3 (1.5) |
CH3CN |
75 |
13 |
Cs2CO3 (0.5) |
CH3CN |
61 |
14 |
Cs2CO3 (0.2) |
CH3CN |
48 |
15 |
Cs2CO3 (1) |
THF |
73 |
16 |
Cs2CO3 (1) |
Dioxane |
50 |
17 |
Cs2CO3 (1) |
DMSO |
57 |
18 |
Cs2CO3 (1) |
EtOH |
25 |
19 |
Cs2CO3 (1) |
H2O |
0 |
20 |
Cs2CO3 (1) |
DMF |
98 |
We then set out to explore the generality of the CDC reaction of thiols with active methylene compounds. We first applied the optimized conditions to the coupling of various thiols 2 with acetylacetone 1a (Table 2). Pleasingly, the results showed that thiophenol substrates bearing different groups such as electron-withdrawing halogen groups (Br, Cl and F) and electron-donating groups (alkyl, OMe, OH and NH2) at the para, meta or ortho or at both positions of aromatic rings, as well as the bulky 2-naphthalenethiol, were all well tolerated. The corresponding 3aa–3as were isolated in moderate to excellent yields, indicating that the electronic and steric effects were not evident in this reaction. The scale-up reaction was also attempted. When we increased the scale of the reaction from 0.4 to 4 mmol, the yield of 3ad only slightly decreased (from 86% to 79%). We then turned our attention to aliphatic thiols. Unfortunately, α-sulfenylation of 1a with benzylthiol or cyclohexylthiol failed to give the desired 3at or 3au.
Table 2 Scope of thiolsa,b

|
Reaction conditions: 1a (0.4 mmol), 2 (0.8 mmol), and Cs2CO3 (0.4 mmol) in DMF (2 mL) stirring at room temperature under air for 6–12 h. Isolated yield based on 1a. The reaction was performed in a 4 mmol scale. |
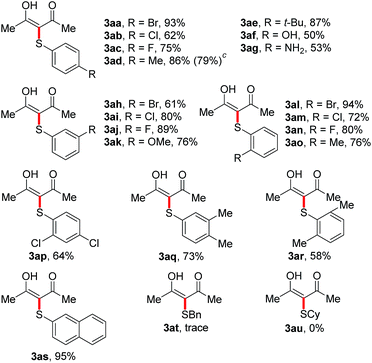 |
Next, the coupling of 4-bromo-thiophenol 2a with a variety of active methylene compounds 1 under the optimized conditions was examined, and the results are illustrated in Table 3. 1,3-Diketones bearing methyl, ethyl, isopropyl and phenyl groups were all applicable to the CDC reaction, leading to the formation of 3ba–3da in 68–85% yields. When ethyl acetoacetate was employed, the reaction also proceeded smoothly to afford 3ea in 82% yield. In addition, dialkyl malonates were also well tolerated, and the desired products 3fa and 3ga were obtained in good yields. We then turned to α-sulfenylation of monosubstituted malonates, and the reaction of 2a with α-alkylmalonates led to the corresponding 3ha and 3ia in 84% and 89% yield, respectively.
Table 3 Scope of active methylene compoundsa,b

|
Reaction conditions: 1 (0.4 mmol), 2a (0.8 mmol), and Cs2CO3 (0.4 mmol) in DMF (2 mL) stirring at room temperature under air for 6–12 h. Isolated yield based on 1. |
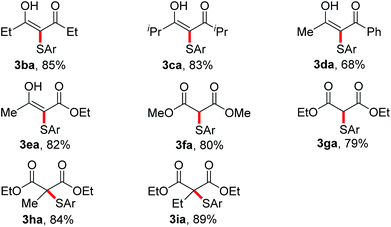 |
To gain more insight into the mechanism of the CDC reaction, a series of control experiments were conducted (Scheme 1). When radical scavenger BQ and BHT was introduced into the reaction, the yield of 3aa reduced from 93% to 0% and 17%, respectively (eqn (1)), suggesting that this transformation might proceed via a radical pathway. In consideration of the generation of disulfides in all cases, the reaction of thiol 2a with Cs2CO3 under air was carried out, leading to the formation of disulfide 4a in quantitative yield (eqn (2)). The above results suggest that Cs2CO3 could increase the oxidation rate of thiols with dioxygen and disulfide was produced via a thiyl radical homocoupling.14a,15 In addition, the reaction of 1a with disulfide 4a under the standard conditions gave 3aa in good yields regardless of the presence of air (eqn (3) and (4)), which demonstrates that disulfide might be an intermediate in the CDC reaction. Moreover, the reaction of 1a with 4a in the absence of Cs2CO3 failed to give 3aa (eqn (5)), which indicates Cs2CO3 is indispensable in this reaction.
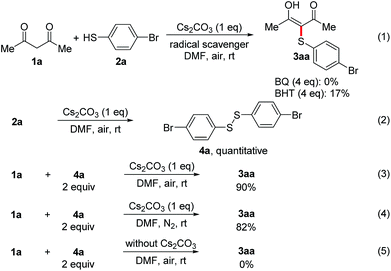 |
| Scheme 1 Mechanistic studies. | |
According to the literatures and our observations, a plausible reaction mechanism is outlined in Scheme 2. Initially, thiyl radical is generated from the autoxidation of thiol 2 in the presence of Cs2CO3 and dioxygen, and thiyl radical undergoes homocoupling to produce disulfide 4.11j,14a,15,16 Meanwhile, active methylene compound 1 reacts with Cs2CO3 to form intermediate 5. Finally, the nucleophilic attack of the in situ-generated enolate 5 on disulfide 4 affords α-sulfenylated carbonyl compound 3.
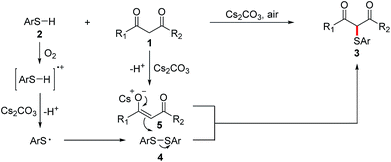 |
| Scheme 2 Proposed mechanism. | |
Conclusions
In conclusion, we have developed the Cs2CO3-promoted cross-dehydrogenative coupling (CDC) of thiophenols with active methylene compounds, which provides a highly convenient and efficient protocol for the synthesis of α-sulfenylated carbonyl compounds with wide functional group compatibility under mild conditions. To the best of our knowledge, this finding is the first example of aerobic CDC reaction of thiols with carbonyl compounds. We envision that the reaction mode outlined here will have potential applications in organic synthesis.
Conflicts of interest
There are no conflicts to declare.
Acknowledgements
This work was supported by the Science and Technology Planning Project of Guangdong Province (No. 2015A020211026 and 2017A010103044), 100 Young Talents Programme of Guangdong University of Technology (220413506), and the Open Fund of the Key Laboratory of Functional Molecular Engineering of Guangdong Province (2016kf07, South China University of Technology).
Notes and references
-
(a) B. M. Trost, Chem. Rev., 1978, 78, 363 CrossRef CAS;
(b) C.-F. Lee, Y.-C. Liu and S. S. Badsara, Chem.–Asian J., 2014, 9, 706 CrossRef CAS PubMed;
(c) P. Chauhan, S. Mahajan and D. Enders, Chem. Rev., 2014, 114, 8807 CrossRef CAS PubMed.
- For selected examples, see:
(a) F.-P. Gendron, E. Halbfinger, B. Fischer, M. Duval, P. D'Orléans-Juste and A. R. Beaudoin, J. Med. Chem., 2000, 43, 2239 CrossRef CAS PubMed;
(b) G. L. Regina, A. Coluccia, A. Brancale, F. Piscitelli, V. Gatti, G. Maga, A. Samuele, C. Pannecouque, D. Schols, J. Balzarini, E. Novellino and R. Silvestri, J. Med. Chem., 2011, 54, 1587 CrossRef PubMed;
(c) G. L. Regina, R. Bai, W. Rensen, A. Coluccia, F. Piscitelli, V. Gatti, A. Bolognesi, A. Lavecchia, I. Granata, A. Porta, B. Maresca, A. Soriani, M. L. Iannitto, M. Mariani, A. Santoni, A. Brancale, C. Ferlini, G. Dondio, M. Varasi, C. Mercurio, E. Hamel, P. Lavia, E. Novellino and R. Silvestri, J. Med. Chem., 2011, 54, 8394 CrossRef PubMed;
(d) M. Klečka, R. Pohl, J. Čejka and M. Hocek, Org. Biomol. Chem., 2013, 11, 5189 RSC;
(e) I. M. Yonova, C. A. Osborne, N. S. Morrissette and E. R. Jarvo, J. Org. Chem., 2014, 79, 1947 CrossRef CAS PubMed.
-
(a) T. Sasaki, K. Hayakawa and H. Ban, Tetrahedron, 1982, 38, 85 CrossRef CAS;
(b) S. Kukolja, S. E. Draheim, J. L. Pfeil, R. D. G. Cooper, B. J. Grvaves, R. E. Holmes, D. A. Neel, G. W. Huffman, J. A. Webber, M. D. Kinnick, R. T. Vasileff and B. J. Foster, J. Med. Chem., 1985, 28, 1886 CrossRef CAS PubMed;
(c) J. M. Matthews, N. Qin, R. W. Colburn, S. L. Dax, M. Hawkins, J. J. McNally, L. Reany, M. A. Youngman, J. Baker, T. Hutchinson, Y. Liu, M. L. Lubin, M. Neeper, M. R. Brandt, D. J. Stone and C. M. Flores, Bioorg. Med. Chem. Lett., 2012, 22, 2922 CrossRef CAS PubMed.
-
(a) H. Loghmani-Khouzani and D. Hajiheidari, J. Fluorine Chem., 2010, 131, 561 CrossRef CAS;
(b) M.-Y. Chang, Y.-C. Cheng and Y.-J. Lu, Org. Lett., 2014, 16, 6252 CrossRef CAS PubMed;
(c) M. T. Saraiva, G. P. Costa, N. Seus, R. F. Schumacher, G. Perin, M. W. Paixão, R. Luque and D. Alves, Org. Lett., 2015, 17, 6206 CrossRef CAS PubMed.
-
(a) S. Hok and N. E. Schore, J. Org. Chem., 2006, 71, 1736 CrossRef CAS PubMed;
(b) A. Kumar, S. Sharma, V. D. Tripathi and S. Srivastava, Tetrahedron, 2010, 66, 9445 CrossRef CAS.
-
(a) B. M. Trost, T. N. Salzmann and K. Hiroi, J. Am. Chem. Soc., 1976, 98, 4887 CrossRef CAS;
(b) M. Orena, G. Porzi and S. Sandri, Tetrahedron Lett., 1992, 33, 3797 CrossRef CAS;
(c) D. Enders, O. Piva and F. Burkamp, Tetrahedron, 1996, 52, 2893 CrossRef CAS;
(d) J. H. Rigby, M. S. Laxmisha, A. R. Hudson, C. H. Heap and M. J. Heeg, J. Org. Chem., 2004, 69, 6751 CrossRef CAS PubMed;
(e) T. Guney and G. A. Kraus, Org. Lett., 2013, 15, 613 CrossRef CAS PubMed.
-
(a) B. C. Ranu and R. Jana, Adv. Synth. Catal., 2005, 347, 1811 CrossRef CAS;
(b) M. A. Rashid, H. Reinke and P. Langer, Tetrahedron Lett., 2007, 48, 2321 CrossRef CAS;
(c) M. A. Rashid, N. Rasool, M. Adeel, H. Reinke, C. Fischer and P. Langer, Tetrahedron, 2008, 64, 3782 CrossRef CAS;
(d) K. Shibatomi, A. Narayama, Y. Soga, T. Muto and S. Iwasa, Org. Lett., 2011, 13, 2944 CrossRef CAS PubMed.
-
(a) X. Huang and W.-X. Zheng, Synth. Commun., 1999, 29, 1297 CrossRef CAS;
(b) B. C. Ranu and T. Mandal, J. Org. Chem., 2004, 69, 5793 CrossRef CAS PubMed;
(c) C. Peppe and L. Borges de Castro, Can. J. Chem., 2009, 87, 678 CrossRef CAS.
- For recent examples, see:
(a) V. K. Yadav, K. G. Babu and M. Parvez, J. Org. Chem., 2004, 69, 3866 CrossRef CAS PubMed;
(b) W. Wang, H. Li, J. Wang and L. Liao, Tetrahedron Lett., 2004, 45, 8229 CrossRef CAS;
(c) K. Deng, J. Chalker, A. Yang and T. Cohen, Org. Lett., 2005, 7, 3637 CrossRef CAS PubMed;
(d) M. Jereb and A. Togni, Org. Lett., 2005, 7, 4041 CrossRef CAS PubMed;
(e) M. Jereb and A. Togni, Chem.–Eur. J., 2007, 13, 9384 CrossRef CAS PubMed;
(f) H. Anbou, R. Umeda and Y. Nishiyama, Bull. Chem. Soc. Jpn., 2011, 84, 1248 CrossRef CAS;
(g) M. Arisawa, Y. Nihei and M. Yamaguchi, Tetrahedron Lett., 2012, 53, 5729 CrossRef CAS;
(h) L.-H. Zou, D. L. Priebbenow, L. Wang, J. Mottweiler and C. Bolm, Adv. Synth. Catal., 2013, 355, 2558 CrossRef CAS;
(i) R. Rahaman, N. Devi and P. Barman, Tetrahedron Lett., 2015, 56, 4224 CrossRef CAS;
(j) Y.-W. Liu, S. S. Badsara, Y.-C. Liu and C.-F. Lee, RSC Adv., 2015, 5, 44299 RSC;
(k) N. Devi, R. Rahaman, K. Sarma and P. Barman, Eur. J. Org. Chem., 2016, 2016, 384 CrossRef CAS.
- For recent reviews, see:
(a) C.-J. Li, Acc. Chem. Res., 2009, 42, 335 CrossRef CAS PubMed;
(b) C. S. Yeung and V. M. Dong, Chem. Rev., 2011, 111, 1215 CrossRef CAS PubMed;
(c) C. Liu, H. Zhang, W. Shi and A. Lei, Chem. Rev., 2011, 111, 1780 CrossRef CAS PubMed;
(d) C. Zhang, C. Tang and N. Jiao, Chem. Soc. Rev., 2012, 41, 3464 RSC;
(e) S. A. Girard, T. Knauber and C.-J. Li, Angew. Chem., Int. Ed., 2014, 53, 74 (Angew. Chem., 2014, 126, 76) CrossRef CAS PubMed.
- For recent examples, see:
(a) S. K. R. Parumala and R. K. Peddinti, Green Chem., 2015, 17, 4068 RSC;
(b) K. Yan, D. Yang, P. Sun, W. Wei, Y. Liu, G. Li, S. Lu and H. Wang, Tetrahedron Lett., 2015, 56, 4792 CrossRef CAS;
(c) D. Yang, K. Yan, W. Wei, J. Zhao, M. Zhang, X. Sheng, G. Li, S. Lu and H. Wang, J. Org. Chem., 2015, 80, 6083 CrossRef CAS PubMed;
(d) J.-P. Wan, S. Zhong, L. Xie, X. Cao, Y. Liu and L. Wei, Org. Lett., 2016, 18, 584 CrossRef CAS PubMed;
(e) D. Yang, P. Sun, W. Wei, L. Meng, L. He, B. Fang, W. Jiang and H. Wang, Org. Chem. Front., 2016, 3, 1457 RSC;
(f) Z. Huang, D. Zhang, X. Qi, Z. Yan, M. Wang, H. Yan and A. Lei, Org. Lett., 2016, 18, 2351 CrossRef CAS PubMed;
(g) Y. Zhu, T. Chen, S. Li, S. Shimada and L.-B. Han, J. Am. Chem. Soc., 2016, 138, 5825 CrossRef CAS PubMed;
(h) J.-G. Sun, H. Yang, P. Li and B. Zhang, Org. Lett., 2016, 18, 5114 CrossRef CAS PubMed;
(i) Y. Siddaraju and K. R. Prabhu, J. Org. Chem., 2016, 81, 7838 CrossRef CAS PubMed;
(j) S. Song, Y. Zhang, A. Yeerlan, B. Zhu, J. Liu and N. Jiao, Angew. Chem., Int. Ed., 2017, 129, 2527 CrossRef;
(k) P. Wang, S. Tang, P. Huang and A. Lei, Angew. Chem., Int. Ed., 2017, 56, 3009 CrossRef CAS PubMed;
(l) Y. Siddaraju and K. R. Prabhu, J. Org. Chem., 2017, 82, 3084 CrossRef CAS PubMed;
(m) Y. Siddaraju and K. R. Prabhu, Org. Biomol. Chem., 2017, 15, 5191 RSC.
-
(a) J. Tan, F. Liang, Y. Wang, X. Cheng, Q. Liu and H. Yuan, Org. Lett., 2008, 10, 2485 CrossRef CAS PubMed;
(b) J. S. Yadav, B. V. S. Reddy, R. Jain and G. Baishya, Tetrahedron Lett., 2008, 49, 3015 CrossRef CAS;
(c) H. Cao, J. Yuan, C. Liu, X. Hu and A. Lei, RSC Adv., 2015, 5, 41493 RSC;
(d) B. V. Varun, K. Gadde and K. R. Prabhu, Org. Lett., 2015, 17, 2944 CrossRef CAS PubMed;
(e) B. V. Varun, K. Gadde and K. R. Prabhu, Org. Biomol. Chem., 2016, 14, 7665 RSC;
(f) Y. Siddaraju and K. R. Prabhu, Org. Lett., 2016, 18, 6090 CrossRef CAS PubMed.
- For recent reviews, see:
(a) Z. Shi, C. Zhang, C. Tang and N. Jiao, Chem. Soc. Rev., 2012, 41, 3381 RSC;
(b) W. Wu and H. Jiang, Acc. Chem. Res., 2012, 45, 1736 CrossRef CAS PubMed;
(c) A. N. Campbell and S. S. Stahl, Acc. Chem. Res., 2012, 45, 851 CrossRef CAS PubMed;
(d) S. E. Allen, R. R. Walvoord, R. Padilla-Salinas and M. C. Kozlowski, Chem. Rev., 2013, 113, 6234 CrossRef CAS PubMed;
(e) C. Liu, D. Liu and A. Lei, Acc. Chem. Res., 2014, 47, 3459 CrossRef CAS PubMed.
-
(a) W.-L. Dong, G.-Y. Huang, Z.-M. Li and W.-G. Zhao, Phosphorus, Sulfur Silicon Relat. Elem., 2009, 184, 2058 CrossRef CAS;
(b) X. Zhang, X. Zhou, H. Xiao and X. Li, RSC Adv., 2013, 3, 22280 RSC;
(c) Y. Liu, Y. Zhang, C. Hu, J.-P. Wan and C. Wen, RSC Adv., 2014, 4, 35528 RSC;
(d) X. Liu, H. Cui, D. Yang, S. Dai, T. Zhang, J. Sun, W. Wei and H. Wang, RSC Adv., 2016, 6, 51830 RSC;
(e) W. He, X. Hou, X. Li, L. Song, Q. Yu and Z. Wang, Tetrahedron, 2017, 73, 3133 CrossRef CAS.
-
(a) T. J. Wallance and A. Schriesheim, J. Org. Chem., 1962, 27, 1514 CrossRef;
(b) T. J. Wallance, A. Schriesheim and W. Bartok, J. Org. Chem., 1963, 28, 1311 CrossRef.
- H. Wang, Q. Lu, C. Qian, C. Liu, W. Liu, K. Chen and A. Lei, Angew. Chem., Int. Ed., 2016, 55, 1094 CrossRef CAS PubMed.
Footnote |
† Electronic supplementary information (ESI) available: General information and copies of 1H and 13C NMR spectra. See DOI: 10.1039/c7ra06904a |
|
This journal is © The Royal Society of Chemistry 2017 |
Click here to see how this site uses Cookies. View our privacy policy here.