DOI:
10.1039/C7RA06464K
(Paper)
RSC Adv., 2017,
7, 34293-34299
Mono and double Mizoroki–Heck reaction of aryl halides with dialkyl vinylphosphonates using a reusable palladium catalyst under aqueous medium†
Received
9th June 2017
, Accepted 30th June 2017
First published on 10th July 2017
Abstract
Operationally-simple and reusable Pd-catalyzed mono and double Mizoroki–Heck reactions of aryl halides and dialkyl vinylphosphonates using iPr2NH as a base in aqueous medium under air were developed. For aryl iodides, the reaction could be conducted at 80 °C under a low catalyst loading (0.1–1 mol%). When aryl bromides were applied, however, a greater amount of catalyst (5 mol%) and a longer reaction time at 120 °C were required. Simply changing dialkyl vinylphosphonates as the limiting reagent led to the formation of 2,2-diaryl vinylphosphonates in good to high yields. After reaction, the residual aqueous solution could be reused for both mono and double Mizoroki–Heck reactions, making the reactions greener and reducing wastage of precious metals and use of harmful organic solvents as the reaction medium.
Introduction
Alkenylphosphonates are important molecules that have been widely applied in organic synthesis,1–14 materials science,15–17 medical chemistry,18–21 and as polymer additives22 and flame retardants.23,24 Transition-metal-catalyzed reactions for the formation of alkenylphosphonates mainly include cross-coupling of P(O)H with vinyl halides or pseudo halides,25–40 olefin cross-metathesis,41 reaction of HP(O) compounds with alkynes42–50 or alkenes,51,52 Suzuki–Miyaura coupling of boronic acids with vinylphosphonates,53–59 and Mizoroki–Heck reaction of vinylphosphonates with various aryl partners.60–66 Although diverse methods have been used and impressive results obtained for the aforementioned reactions, most methods of such catalytic reactions use harmful organic solvents, such as CH2Cl2, toluene, and DMF, as the reaction medium. It would therefore be highly valuable to develop a transition-metal-catalyzed reaction for the synthesis of alkenylphosphonates that does not employ an organic solvent as the reaction medium. The introduction of neat water as the reaction medium possesses several advantages, including a low cost, nontoxicity, non-flammable nature, and easy separation from the organic products and reuse of water-soluble catalysts.67–84 Although heterogeneous supported palladium catalysts have been applied to study recyclability, harmful DMF was employed as the reaction medium.64
On the other hand, transition-metal-catalyzed cross-coupling for the formation of 2,2-diaryl vinylphosphonates is rare. Known procedures include phosphorylation of 1,1-diarylethene by Ag51,85 or Cu,26,27,86 arylzincation of alkynylphosphonates by Co,87 Negishi coupling using vinylic tellurides,88 arylation of alkynylphosphonates with aryl halides or arylboronic acids,53,89,90 and HUSY zeolite-promoted hydrophenylation of diethyl (2-phenylethynyl)phosphonate with benzene.91 However, the coupling of aryl halides and vinylphosphonates via a double Mizoroki–Heck reaction has not yet been explored.
In this study, we developed a green and operationally-simple palladium-catalyzed procedure to synthesize 2-aryl and 2,2-diaryl vinylphosphonates using water as the reaction medium under aerobic conditions. The use of aryl halides as the limiting reagent produces mono Mizoroki–Heck products; on the contrary, double Mizoroki–Heck products are selectively formed when vinylphosphonate plays the role of limiting reagent (Scheme 1). Moreover, the reusability of the residual aqueous solution for these two kinds of reaction was also examined.
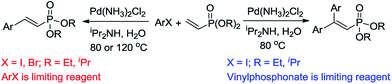 |
| Scheme 1 Pd-catalyzed mono and double Mizoroki–Heck reactions in water. | |
Results and discussion
In previous studies, we found that iPr2NH is the best base to employ for the coupling of aryl halides and dialkyl allylphosphonates;92 therefore, it was also used as the base in the Mizoroki–Heck reaction of aryl halides and dialkyl vinylphosphonate. As shown in Table 1, iodobenzene 1a (1 mmol), diethyl vinylphosphonate 2a (2 mmol), iPr2NH (1.1 mmol), and PdCl2(NH3)2 (5 mol%) were reacted in an open-air reactor at 80 °C using water (3 mL) as the solvent. Under such conditions, 3a was obtained in a near-quantitative yield within 24 h (Entry 1). In order to optimize the conditions, reducing the catalyst loading to 1 mol% afforded 3a in a 98% isolated yield (Entry 2). Upon investigation of the reaction time, it was found that 6 h was sufficient for the reaction to reach completion, indicating the high efficiency of this catalytic system (Entries 3–5). With an auxiliary water-soluble cationic 2,2′-bipyridyl ligand,93 a 99% product yield was achieved under conditions identical to those for Entry 4 (Entry 6). The results showed that the water-soluble cationic 2,2′-bipyridyl ligand may be not crucial in the coupling reaction. Use of this catalyst without an auxiliary ligand to fulfill the Mizoroki–Heck reaction has been described previously, in which Pd nanoparticles were formed during the coupling reaction.92 The reaction rates of vinylphosphonates were faster than those of allylphosphonates, which may be due to the electron-withdrawing phosphonate group of 2a becoming attached directly to the vinyl carbon, which lowers the π* energy level of the olefin, facilitating coordination of the π bond to palladium. Then, the catalyst loading was further reduced to 0.1 mol%, resulting in 68% and 70% product yields, respectively, with a prolonged reaction time (Entries 7 and 8). When 2a was replaced by diisopropyl vinylphosphonate, 2b, a near quantitative yield of 4a was also delivered under the conditions of Entry 4 (Entry 9). Other commonly-used palladium salts were also examined; however, the product yields were much lower than those obtained with PdCl2(NH3)2 (Entries 10–12). Therefore, reaction with 1 mol% PdCl2(NH3)2 in the absence of an auxiliary ligand (Entry 4) was chosen as the optimal condition.
Table 1 Optimization of palladium-catalyzed Mizoroki–Heck reaction of 1a and 2a in watera

|
Entry |
Pd (mol%) |
Time (h) |
Yield (%)b |
Reaction conditions: 1a (1.0 mmol), 2a (2.0 mmol) iPr2NH (1.1 mmol), PdCl2(NH3)2, and H2O (3 mL) at 80 °C under air. Isolated yields. Cationic 2,2′-bipyridyl was used as the ligand. Diisopropyl vinylphosphonate (2b) was used as the reactant. |
1 |
PdCl2(NH3)2 (5) |
24 |
99 |
2 |
(1) |
24 |
98 |
3 |
(1) |
12 |
97 |
4 |
(1) |
6 |
99 |
5 |
(1) |
3 |
81 |
6c |
(1) |
6 |
99 |
7 |
(0.1) |
36 |
68 |
8 |
(0.1) |
48 |
70 |
9d |
(1) |
6 |
99 |
10 |
Pd(OAc)2 (1) |
6 |
72 |
11 |
PdCl2 (1) |
6 |
42 |
12 |
PdCl2(MeCN)2 (1) |
6 |
28 |
Following optimization of the reaction conditions (Table 1, Entry 4), a variety of aryl iodides 1 were reacted with 2 in order to explore the scope of this catalytic system. As shown in Table 2, electron-rich aryl iodides 1b and 1c were arylated to 2 smoothly, delivering the corresponding products in high yields (Entries 1 and 2). With electron-deficient aryl iodides, for instance 1d–j, the reactions took place very efficiently, and the desired products 3d–j and 4d–j were obtained in excellent yields (Entries 3–9). Sterically-congested 1k–m did not affect the reaction, and excellent product yields were achieved (Entries 10–12). 3-Substituted aryl iodides 1n–p, without an electronic effect on the aromatic ring, were able to be applied as reactants to couple with 2, providing the desired products 3n–p and 4n–p in excellent yields (Entries 13–15).
Table 2 Pd-catalyzed monoarylation of aryl iodides 1 to dialkyl vinylphosphonate 2 in watera
The oxidative addition of aryl bromides to palladium is more difficult than that of iodides. So, increases of the reaction temperature and catalyst loading were required to achieve the coupling reaction when aryl bromides were employed. As illustrated in Table 3, Mizoroki–Heck reaction of 5a and 2 with 5 mol% PdCl2(NH3)2 at 120 °C in a sealable tube for 36 h led to the formation of 3a and 4a in 68% and 69% yields, respectively (Entry 1). Coupling of electron-rich aryl bromides 5b–c with 2 (Entries 2 and 3) was much slower than with electron-poor 5d–j (Entries 4–9). A steric effect on 2-substituted aryl bromides was apparent; therefore, coupling of 5k–m with 2 gave the target products in yields of between 35% and 61%, respectively (Entries 10–12). For 3-substituted aryl bromides, such as 5n–p, the products were given in yields comparable with those produced using 5a (Entries 13–15).
Table 3 Pd-catalyzed monoarylation of aryl bromides 5 to dialkyl vinylphosphonate 2 in watera
When the limiting reagent was changed from aryl halides to vinylphosphonates, we were pleased to find that double Mizoroki–Heck products could be selectively obtained (Table 4). Under a lower catalyst loading, the reaction gave only a 47% yield of 6a when conducted at 80 °C for 48 h (Entry 1). Further increasing the catalyst loading to 5 mol% was required to obtain a satisfactory outcome (Entry 2). Both electron-rich and -deficient aryl iodides were able to couple with 2, giving the desired products in good to high yields (Entries 3–6). However, low yields were furnished when the trifluoromethyl group was located at the para position (Entry 7). It was known that 4-substituted aryl iodides with strong electron-withdrawing functional groups such as NO2- and CF3-render the double Mizoroki–Heck reaction inert.94 Sterically-congested aryl iodides, such as 1k and 1l, were transformed efficiently to the corresponding 2,2-diaryl vinylphosphonates in higher yields, indicating that the steric effect did not hinder this double Mizoroki–Heck reaction (Entries 8 and 9). 3-Substituted aryl iodides 1n–1p were also well-tolerated under such conditions, which provided the corresponding double Mizoroki–Heck products in good to high yields (Entries 10–12).
Table 4 Pd-catalyzed double arylation of aryl iodides 1 to dialkyl vinylphosphonate 2 in watera
The proposed mechanism for both mono and double Mizoroki–Heck reactions was shown in Scheme 2. After the oxidative addition of an aryl halide to Pd(0) nanoparticles, coordination of a dialkyl vinylphosphonate to Pd(II) and subsequent migratory insertion of the aryl group occurred. The following β-hydride elimination delivered a mono Mizoroki–Heck product along with palladium hydride, which was neutralized by a base to regenerate Pd(0) nanoparticles. With regard to the double Mizoroki–Heck reaction, the produced mono Mizoroki–Heck product in the first cycle acted as the alkene for the Pd(II) coordination. After migratory insertion and β-hydride elimination, the double Mizoroki–Heck product was afforded.
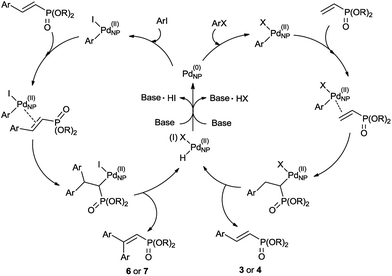 |
| Scheme 2 Proposed mechanism for the mono and double Mizoroki–Heck reaction. | |
On the basis of the broad scope of this catalytic reaction, the reusability of the aqueous catalytic solution was then examined, and the results are summarized in Table 5. Under conditions identical to those described in Table 2, Entry 4, after the initial reaction of 1e and 2a, the reaction mixture was extracted with ethyl acetate (3 × 3 mL) and 3e was purified by column chromatography. The remaining aqueous solution was then charged with 1e, 2a, and base for the reuse run. Only slightly decreased product yields were obtained in the reuse runs (Entry 1). When 5i was employed, we found that the catalytic activity decayed quickly, and the formation of palladium black on the glass reactor was observed due to the higher reaction temperature (Entry 2).92 In reuse studies for the double Mizoroki–Heck reaction, 1p and 2a were chosen as the representative reactants. After extracting with ethyl acetate (3 × 3 mL), a 54% isolated yield of 6p could be still achieved in the second reuse run (Entry 3). This operationally-simple procedure therefore provides opportunities to reduce wastage of precious metals and decrease use of harmful organic solvents as the reaction medium, and meets the criteria of green chemistry.
Table 5 Reuse studies of aryl halides and 2a for mono and double Mizoroki–Heck reactionsa
Entry |
ArX |
Product |
Yield (%)b |
Initial run |
1st reuse |
2nd reuse |
Reaction conditions: ArX (1 mmol for Entries 1 and 2, 2.5 mmol for Entry 3), 2a (2 mmol for Entries 1 and 2, 1 mmol for Entry 3), PdCl2(NH3)2 (1 mol% for Entry 1, 5 mol% for Entries 2 and 3) iPr2NH (1.1 mmol for Entries 1 and 2, 2.5 mmol for Entry 3), and H2O (3 mL) at 80 °C (Entries 1 and 3) or 120 °C (Entry 2). Isolated yields. |
1 |
1e |
3e |
95 |
88 |
76 |
2 |
5i |
3i |
83 |
68 |
23 |
3 |
1p |
6p |
74 |
63 |
54 |
Experimental
General information
Chemicals were purchased from commercial suppliers and were used without further purification. 2b was synthesized according to the published procedure using P(OiPr)3 as the starting material.95 All 1H and 13C NMR spectra were recorded in CDCl3 at 25 °C on a Bruker 300 NMR spectrometer, where chemical shifts (δ in ppm) were determined with respect to the nondeuterated solvent as a reference (1H NMR: CHCl3 at 7.24 ppm; 13C NMR: CDCl3 at 77.0 ppm). 31P NMR spectra for unknown compounds were recorded in CDCl3 at 25 °C on a Varian 400 NMR spectrometer using 85% H3PO4 as an external standard. Melting points were recorded using melting point apparatus and were uncorrected. HRMS for unknown compounds were recorded at the Instrument Center Service of National Central University, Ministry of Science and Technology of Taiwan. All known products obtained were pure, and their spectra (1H and 13C NMR) were in agreement with those of authentic samples (see the ESI† for the spectral data of all mono and double Mizoroki–Heck products).
Typical procedure for the mono Mizoroki–Heck reaction
In a 25 mL reaction tube, PdCl2(NH3)2 (1 mol% for 1 or 5 mol% for 5) was added to H2O (3 mL) and the solution was stirred at room temperature for 10 min. The tube was then charged with 1 or 5 (1.0 mmol), 2 (2.0 mmol), and iPr2NH (1.1 mmol) and stirred at 80 °C for 6 h or 120 °C (for which a sealable tube was used) for 36 h. After cooling the reaction to room temperature, the resulting mixture was extracted with EtOAc (3 × 3 mL). The combined organic phase was dried over MgSO4, and the solvent was then removed under vacuum. Column chromatography on silica gel (hexane/EtOAc = 1/9) afforded the desired product.
Typical procedure for the double Mizoroki–Heck reaction
In a 25 mL reaction tube, PdCl2(NH3)2 (5 mol%) was added to H2O (3 mL) and the solution was stirred at room temperature for 10 min. The tube was then charged with 1 (2.5 mmol), 2 (1.0 mmol), and iPr2NH (2.5 mmol) and stirred at 80 °C for 48 h. After cooling the reaction to room temperature, the resulting mixture was extracted with EtOAc (3 × 3 mL). The combined organic phase was dried over MgSO4, and the solvent was then removed under vacuum. Column chromatography on silica gel (hexane/EtOAc = 1/9) gave the desired product.
Typical procedure for reuse of the residual aqueous solution for mono and double Mizoroki–Heck reactions
The reaction was conducted following the previously-described procedure. After reaction, the aqueous reaction mixture was extracted three times with EtOAc (3 mL) under vigorous stirring, and the organic product was isolated from the combined organic phase according to the previously-described procedure. The residual aqueous solution was then charged with 1 or 5, 2, and iPr2NH for the next run.
Conclusions
In conclusion, we have successfully developed an operationally-simple and environmentally-benign Pd-catalyzed reaction for mono and double Mizoroki–Heck arylation of aryl halides and dialkyl vinylphosphonates using the greenest solvent, water, as the reaction medium under air. 2-Arylvinylphosphonates or 2,2-diarylvinylphosphonates can be selectively obtained by simply employing aryl halides or dialkyl vinylphosphonates as the limiting reagent. In addition, although the employment of aryl bromide showed poor reusability, the residual aqueous solution can be reused several times, making the reaction greener and greatly reduces the wastage of precious metals and use of harmful organic solvents.
Acknowledgements
This research was financially supported by the Ministry of Science and Technology of Taiwan (MOST 105-2113-M-027-004). We thank Prof. Yi-Tsu Chan (National Taiwan University) for obtaining the 31P NMR spectra.
References
- I. P. Beletskaya and M. A. Kazankova, Russ. J. Org. Chem., 2002, 38, 1391 CrossRef CAS
. - F. Alonso, I. P. Beletskaya and M. Yus, Chem. Rev., 2004, 104, 3079 CrossRef CAS PubMed
. - A. L. Schwan, Chem. Soc. Rev., 2004, 33, 218 RSC
. - D. Leca, L. Fensterbank, E. Lacôte and M. Malacria, Chem. Soc. Rev., 2005, 34, 858 RSC
. - S. Marque and P. Tordo, Top. Curr. Chem., 2005, 250, 43 CAS
. - O. Delacroix and A. C. Gaumont, Curr. Org. Chem., 2005, 9, 1851 CrossRef CAS
. - S. N. Arbuzova, N. K. Gusarova and B. A. Trofimov, ARKIVOC, 2006, 12 CAS
. - L. Coudray and J. L. Montchamp, Eur. J. Org. Chem., 2008, 3601 CrossRef CAS PubMed
. - S. Van der Jeught and C. V. Stevens, Chem. Rev., 2009, 109, 2672 CrossRef CAS PubMed
. - T. Janecki, J. Kędzia and T. Wąsek, Synthesis, 2009, 1227 CrossRef CAS
. - D. S. Glueck, Top. Organomet. Chem., 2010, 31, 65 CrossRef CAS
. - F. M. J. Tappe, V. T. Trepohl and M. Oestreich, Synthesis, 2010, 3037 CAS
. - D. Zhao and R. Wang, Chem. Soc. Rev., 2012, 41, 2095 RSC
. - H. Yorimitsu, Beilstein J. Org. Chem., 2013, 9, 1269 CrossRef CAS PubMed
. - H. Steininger, M. Schuster, K. D. Kreuer, A. Kaltbeitzel, B. Bingol, W. H. Meyer, S. Schauff, G. Brunklaus, J. Maier and H. W. Spiess, Phys. Chem. Chem. Phys., 2007, 9, 1764 RSC
. - S. Kirumakki, J. Huang, A. Subbiah, J. Yao, A. Rowland, B. Smith, A. Mukherjee, S. Samarajeewa and A. Clearfield, J. Mater. Chem., 2009, 19, 2593 RSC
. - V. Spampinato, N. Tuccitto, S. Quici, V. Calabrese, G. Marletta, A. Torrisi and A. Licciardello, Langmuir, 2010, 26, 8400 CrossRef CAS PubMed
. - T. Minami and J. Motoyoshiya, Synthesis, 1992, 333 CrossRef CAS
. - H. B. Lazrek, A. Rochdi, H. Khaider, J.-L. Barascut, J.-L. Imbach, J. Balzrini, M. Witvrouw, C. Pannecouque and E. De Clercq, Tetrahedron, 1998, 54, 3807 CrossRef CAS
. - P. Page, C. Blonski and J. Perie, Eur. J. Org. Chem., 1999, 2853 CrossRef CAS
. - D. L. Jakeman, A. J. Ivory, M. P. Williamson and G. M. Blackburn, J. Med. Chem., 1998, 41, 4439 CrossRef CAS PubMed
. - S. Jin and K. E. Gonsalves, Macromolecules, 1998, 31, 1010 CrossRef CAS
. - G. A. Russell, C.-F. Yao, H. I. Tashtoush, J. E. Russell and D. F. Dedolph, J. Org. Chem., 1991, 56, 663 CrossRef CAS
. - M. Ruiz, M. C. Fernandez, S. Conde, A. Diaz and J. M. Quintela, Synlett, 1999, 1903 CrossRef CAS
. - S. Thielges, P. Bisseret and J. Eustache, Org. Lett., 2005, 7, 681 CrossRef CAS PubMed
. - G. Evano, K. Tadiparthi and F. Couty, Chem. Commun., 2011, 47, 179 RSC
. - K. Jouvin, A. Coste, A. Bayle, F. Legrand, G. Karthikeyan, K. Tadiparthi and G. Evano, Organometallics, 2012, 31, 7933 CrossRef CAS
. - L. Liu, Y. Wang, Z. Zeng, P. Xu, Y. Gao, Y. Yin and Y. Zhao, Adv. Synth. Catal., 2013, 355, 659 CrossRef CAS
. - Y. Wu, L. Liu, K. Yan, P. Xu, Y. Gao and Y. Zhao, J. Org. Chem., 2014, 79, 8118 CrossRef CAS PubMed
. - L. Liu, Y. Lv, Y. Wu, X. Gao, Z. Zeng, Y. Gao, G. Tang and Y. Zhao, RSC Adv., 2014, 4, 2322 RSC
. - J.-F. Xue, S.-F. Zhou, Y.-Y. Liu, X. Pan, J.-P. Zou and O. T. Asekun, Org. Biomol. Chem., 2015, 13, 4896 CAS
. - D. A. Holt and J. M. Erb, Tetrahedron Lett., 1989, 30, 5393 CrossRef CAS
. - M. A. Kazankova, I. G. Trostyanskaya, S. V. Lutsenko and I. P. Beletskaya, Tetrahedron Lett., 1999, 40, 569 CrossRef CAS
. - T. Okuchi, T. Yano, T. Fukamachi, J. Ichikawa and T. Minami, Tetrahedron Lett., 1999, 40, 5337 CrossRef
. - P. Zhong, Z. X. Xiong and X. Huang, Synth. Commun., 2000, 30, 273 CrossRef CAS
. - T. Hirao, T. Masunaga, Y. Ohshiro and T. Agawa, Tetrahedron Lett., 1980, 21, 3595 CrossRef CAS
. - N. B. Karlstedt, M. V. Anokhin and I. P. Beletskaya, Russ. Chem. Bull., Int. Ed., 2013, 62, 2498 CrossRef CAS
. - T. Ogawa, N. Usuki and N. Ono, J. Chem. Soc., Perkin Trans. 1, 1998, 2953 RSC
. - T. Hirao, T. Masunaga, N. Yamada, Y. Ohshiro and T. Agawa, Bull. Chem. Soc. Jpn., 1982, 55, 909 CrossRef CAS
. - X. Zhang, H. Liu, X. Hu, G. Tang, J. Zhu and Y. Zhao, Org. Lett., 2011, 13, 3478 CrossRef CAS PubMed
. - A. K. Chatterjee, T.-L. Choi and R. H. Grubbs, Synlett, 2001, 1034 CrossRef CAS
. - L.-B. Han, C.-Q. Zhao, S. Onozawa, M. Goto and M. Tanaka, J. Am. Chem. Soc., 2002, 124, 3842 CrossRef CAS PubMed
. - L.-B. Han, C. Zhang, H. Yazawa and S. Shimada, J. Am. Chem. Soc., 2004, 126, 5080 CrossRef CAS PubMed
. - M. Niu, H. Fu, Y. Jiang and Y. Zhao, Chem. Commun., 2007, 272 RSC
. - S. K. Nune and M. Tanaka, Chem. Commun., 2007, 2858 RSC
. - V. P. Ananikov, L. L. Khemchyan, I. P. Beletskaya and Z. A. Starikova, Adv. Synth. Catal., 2010, 352, 2979 CrossRef CAS
. - G. Evano, A.-C. Gaumont, C. Alayrac, I. E. Wrona, J. R. Giguere, O. Delacroix, A. Bayle, K. Jouvin, C. Theunissen, J. Gatignol and A. C. Silvanus, Tetrahedron, 2014, 70, 1529 CrossRef CAS
. - I. G. Trostyanskaya and I. P. Beletskaya, Tetrahedron, 2014, 70, 2556 CrossRef CAS
. - L.-B. Han and M. Tanaka, J. Am. Chem. Soc., 1996, 118, 1571 CrossRef CAS
. - L. Liu, Y. Wu, Z. Wang, J. Zhu and Y. Zhao, J. Org. Chem., 2014, 79, 6816 CrossRef CAS PubMed
. - Q. Gui, L. Hu, X. Chen, J. Liu and Z. Tan, Chem. Commun., 2015, 51, 13922 RSC
. - J.-W. Yuan, L.-R. Yang, P. Mao and L.-B. Qu, RSC Adv., 2016, 6, 87058 RSC
. - I. Pergament and M. Srebnik, Org. Lett., 2001, 3, 217 CrossRef CAS PubMed
. - Y. Kobayashi and A. D. William, Org. Lett., 2002, 4, 4241 CrossRef CAS PubMed
. - G. W. Kabalka and S. K. Guchhait, Org. Lett., 2003, 5, 729 CrossRef CAS PubMed
. - G. W. Kabalka, S. K. Guchhait and A. Naravane, Tetrahedron Lett., 2004, 45, 4685 CrossRef CAS
. - Y. Kobayashi and A. D. William, Adv. Synth. Catal., 2004, 346, 1749 CrossRef CAS
. - R. Zhuang, J. Xu, Z. Cai, G. Tang, M. Fang and Y. Zhao, Org. Lett., 2011, 13, 2110 CrossRef CAS PubMed
. - X. Mi, M. Huang, H. Guo and Y. Wu, Tetrahedron, 2013, 69, 5123 CrossRef CAS
. - A. Burini, S. Cacchi, P. Pace and B. R. Pietroni, Synlett, 1995, 677 CrossRef CAS
. - Y. Na, S. Park, S. B. Han, H. Han, S. Ko and S. Chang, J. Am. Chem. Soc., 2004, 126, 250 CrossRef CAS PubMed
. - L. K. Hwang, Y. Na, J. Lee, Y. Do and S. Chang, Angew. Chem., Int. Ed., 2005, 44, 6166 CrossRef CAS PubMed
. - W. Al-Maksoud, J. Mesnager, F. Jaber, C. Pinel and L. Djakovitch, J. Organomet. Chem., 2009, 694, 3222 CrossRef CAS
. - J. Tarabay, W. Al-Maksoud, F. Jaber, C. Pinel, S. Prakash and L. Djakovitch, Appl. Catal., A, 2010, 388, 124 CrossRef CAS
. - H. Brunner, N. Le Cousturier de Courcy and J.-P. Genet, Synlett, 2000, 201 CrossRef CAS
. - N. N. Demik, M. M. Kabachnij, Z. S. Novikova and I. P. Beletskaya, Zh. Org. Khim., 1995, 31, 64 CAS
. - E. Levin, E. Ivry, C. E. Diesendruck and N. G. Lemcoff, Chem. Rev., 2015, 115, 4607 CrossRef CAS PubMed
. - B. Li and P. H. Dixneuf, Chem. Soc. Rev., 2013, 42, 5744 RSC
. - M.-O. Simon and C.-J. Li, Chem. Soc. Rev., 2012, 41, 1415 RSC
. - R. N. Butler and A. G. Coyne, Chem. Rev., 2010, 110, 6302 CrossRef CAS PubMed
. - K. H. Shaughnessy, Chem. Rev., 2009, 109, 643 CrossRef CAS PubMed
. - I. T. Horváth, Green Chem., 2008, 10, 1024 RSC
. - C.-J. Li and B. M. Trost, Proc. Natl. Acad. Sci. U. S. A., 2008, 105, 13197 CrossRef CAS PubMed
. - S. Liu and J. Xiao, J. Mol. Catal. A: Chem., 2007, 270, 1 CrossRef CAS
. - D. Dallinger and C. O. Kappe, Chem. Rev., 2007, 107, 2563 CrossRef CAS PubMed
. - I. T. Horváth and P. T. Anastas, Chem. Rev., 2007, 107, 2167 CrossRef PubMed
. - C.-J. Li and L. Chen, Chem. Soc. Rev., 2006, 35, 68 RSC
. - C.-J. Li, Chem. Rev., 2005, 105, 3095 CrossRef CAS PubMed
. - N. E. Leadbeater, Chem. Commun., 2005, 2881 RSC
. - R. N. Butler and A. G. Coyne, Org. Biomol. Chem., 2016, 14, 9945 CAS
. - K. Piradashvili, E. M. Alexandrino, F. R. Wurm and K. Landfester, Chem. Rev., 2016, 116, 2141 CrossRef CAS PubMed
. - M. B. Gawande, V. B. D. Bonifácio, R. Luque, P. S. Branco and R. S. Varma, Chem. Soc. Rev., 2013, 42, 5522 RSC
. - N. Mase and C. F. Barbas III, Org. Biomol. Chem., 2010, 8, 4043 CAS
. - A. Chanda and V. V. Fokin, Chem. Rev., 2009, 109, 725 CrossRef CAS PubMed
. - J. Zhao, P. Li, X. Li, C. Xia and F. Li, Chem. Commun., 2016, 52, 3661 RSC
. - L.-L. Mao, A.-X. Zhou, N. Liu and S.-D. Yang, Synlett, 2014, 2727 CAS
. - K. Murakami, H. Yorimitsu and K. Oshima, Org. Lett., 2009, 11, 2373 CrossRef CAS PubMed
. - D. Alves, R. F. Schumacher, R. Brandão, C. W. Nogueira and G. Zeni, Synlett, 2006, 1035 CAS
. - I. Pergament and M. Srebnik, Tetrahedron Lett., 2001, 42, 8059 CrossRef CAS
. - K. V. Sajna, V. Srinivas and K. C. K. Swamy, Adv. Synth. Catal., 2010, 352, 3069 CrossRef CAS
. - D. S. Ryabukhin and A. V. Vasilyev, Mendeleev Commun., 2016, 26, 500 CrossRef CAS
. - W.-T. Liao, X.-J. Yang, Y.-Y. Tseng, C.-C. Wu, L.-J. Liu and F.-Y. Tsai, Asian J. Org. Chem., 2015, 4, 1112 CrossRef CAS
. - S.-N. Chen, W.-Y. Wu and F.-Y. Tsai, Tetrahedron, 2008, 64, 8164 CrossRef CAS
. - D. Xu, C. Lu and W. Chen, Tetrahedron, 2012, 68, 1466 CrossRef CAS
. - P. Fourgeaud, C. Midrier, J.-P. Vors, J.-N. Volle, J.-L. Pirat and D. Virieux, Tetrahedron, 2010, 66, 758 CrossRef CAS
.
Footnote |
† Electronic supplementary information (ESI) available: Spectroscopic characterization data of all mono and double Mizoroki–Heck products; HRMS data; copies of 1H, 13C, and 31P NMR spectra for unknown compounds. See DOI: 10.1039/c7ra06464k |
|
This journal is © The Royal Society of Chemistry 2017 |
Click here to see how this site uses Cookies. View our privacy policy here.