DOI:
10.1039/C7RA06183H
(Paper)
RSC Adv., 2017,
7, 46894-46899
Kinetic study of application of graphene oxide as a catalyst to accelerate extraction of total flavonoids from Radix Scutellaria
Received
3rd June 2017
, Accepted 20th August 2017
First published on 4th October 2017
Abstract
A new kinetic model, So's three-step kinetic model, was used to study the kinetics of graphene oxide (GO)-assisted ethanol reflux extraction (GERE). An experimental design to investigate the effect of five single factors (extraction temperature, dosage amount of GO, concentration of ethanol aqueous solution, the ratio of liquid to solid, rotating speed) on the kinetic extraction of total flavonoids was materialized. From the single factors experiments, we found that the extraction process is mainly controlled by internal diffusion, and the extraction rate increases with the growth of GO dosage, ethanol concentration and liquid–solid ratio at 30–70 °C; moreover, the rotating speed had little effect. The effective internal diffusion coefficient (D′) and the activation energy (Ea) of total flavonoids extracted by ERE and GERE were also studied, respectively. Results showed a good prediction of So's three-step kinetic model for extraction kinetics in all experiments, which gave the possibility for estimating the initial rate and extent of GERE.
1. Introduction
Radix Scutellaria is a commonly used herbal medicine that is extensively used for the treatment of inflammation, pyrexia, jaundice, diarrhea and hepatitis, etc.1,2 The major types of flavonoids in Radix Scutellaria are baicalin (BG), baicalein (B), wogonoside (WG), and wogonin (W).3 The pharmacological studies in vivo and in vitro have shown that B and W exhibit stronger bioactivities than their glucuronides BG and WG, particularly with respect to their antioxidant and anti-angiogenesis properties.4,5 Scutellaria baicalensis flavonoids have antibacterial, antifungal, anti-cancer, anti-virus, anti-microbial, anti-inflammatory and anti-metamorphosis, antioxidant and other biological effects.1 At present, the methods of extracting flavonoids from Scutellaria baicalensis are mainly the solvent extraction method,6 ultrasonic extraction method,7 acid hydrolysis method and enzymatic hydrolysis method.8,9 The acid hydrolysis method and the enzymatic hydrolysis method are currently recognized as novel and superior extraction methods, but problems still exist. The former is often restricted by the relatively high cost of highly efficient enzymes and the latter often involve hydrochloric acid or sulfuric acid as a catalyst, bringing a large amount of acidic effluent that may damage the environment. Therefore, it is necessary to develop a novel green process to resolve the problems mentioned above. In a previous study,10 we, for the first time, used graphene oxide (GO) to catalyze the hydrolyzation of Scutellaria flavone glycosides and supplied an alternative approach to green hydrolyzation, extending the application of GO. It was found that the GO-assisted ethanol reflux extraction possesses a synergistic effect between the GO hydrolysis of cell walls and the flavonoids leaching in the ethanol aqueous solution, which significantly increased the extraction rate of the total flavonoids and baicalein.10 For industrial applications and mass production, the kinetics study is necessary, because the kinetics study of GERE can give a good prediction for the extraction kinetics in experiments, which gives the possibility for estimating the initial rate and extent of GERE. Research on GERE extraction kinetics has not been reported. Herein, we used a new kinetics model, So's three-step kinetic model,11 to study the kinetics mechanism of GERE, in order to establish the extraction kinetics equation, which gives the possibility for estimating the initial rate and extent of GO-assisted ethanol reflux extraction (GERE). To achieve these goals, we studied the influence of extraction time, extraction temperature, ethanol concentration, GO's dosage, rotating speed and ratio of solvent to raw material on the extraction yields of the total flavonoids. The conventional ethanol reflux extraction method (ERE) was also carried out and treated as a control.
2. Materials and methods
2.1 Materials
Scutellaria radix pieces (Inner Mongolia Scutellaria) were purchased from Hefei Pharmacies CO., China. All Scutellaria radix pieces were cleaned, separated from impurities, dried and sieved to 10–20 mesh for the following experiments. GO was prepared by a modified Hummers method.12 The specific prepared process and the characterization results of GO can be seen in our earlier studies.10
2.2 Chemicals and reagents
The flavone standards, baicalin (98%) and baicalein (98%) were purchased from Chinese Food and Drug Testing Institute (Shanghai, China). Ethanol (99.7%) of analytical grade for extraction was bought from Sinopharm Chemical Reagent Co. (Shanghai, China). Deionized water was prepared using a water purification system purchased from HITECH Instruments Co. LTD (Henan, China).
2.3 Extraction procedures
2.3.1 Extraction devices. The HH-2 digital thermostat water bath was purchased from Guohua Electric Co. (Shanghai, China). A UV-2550 UV-visible spectrophotometer was purchased from Shimadzu Corporation (Tokyo, Japan).
2.3.2 GO-assisted ethanol reflux extraction (GERE). Radix Scutellaria powder (10.0 g) was loaded in a 250 mL flask (flat bottom) containing a volume of extraction solvent and an amount of GO according to the experimental design. The flask was then immersed into the digital thermostat water bath to maintain a constant temperature throughout the period of extraction. The temperature of the flask was measured with a mercury-in-glass thermometer having ±0.1 °C divisions. The extraction experiments were started when the system reached the desired temperature. To prevent the loss of solvent during extraction, a condenser was connected to the flask. Using the experimental design, the effect of five single factors (extraction temperature, dosage amount of GO, concentration of ethanol aqueous solution, the ratio of liquid to solid, rotating speed) on the yields of total flavonoids (η) was investigated under the extraction process, and samples were taken at 1, 2, 3, 4, 5, 10, 20, 30, 40, 50, 60, 80, 100, 120, 150, 180, 240, 300, and 360 min. After extraction, the resulting solution was centrifuged, and the supernatant was decanted away for UV analysis. At least two replicates were performed for each extraction condition tested, and all analyses were repeated three times. The yields of total flavonoids (η) was defined as follows: |
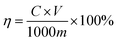 | (1) |
C was the total flavonoids' concentration of the extraction solution (mg mL−1), calculated by the standard curve detected by UV-visible spectrophotometry,13 V was the extraction solution volume (mL), m was the quality of dry raw material (g). Simultaneously, ERE was also performed without GO as a catalyst at the same experimental conditions compared with GERE.
2.3.3 Modeling equations. The extraction of total flavonoids from Scutellaria was assumed to be a three-step extraction. First, the solvent dissolves the solute and penetrates into the Scutellaria cells. Second, the flavonoid particles diffuse from the surface of the Scutellaria cells (inner diffusion), and then finally diffuse into the solvent (external diffusion). External diffusion resistance can be ignored because of extensive stirring during the extraction process. So et al.11 referred to the three stages of these two processes as the washing stage, the first diffusion stage (fast diffusion stage) and the second diffusion stage (slow diffusion stage). So et al.11 described the three-stage model according to Fick's second law, and the extraction rate of the ingredient product can be expressed by the equation: |
ηt = ηwe[1 − exp(−kwt)] + ηd1e[1 − exp(−kd1t)] + ηd2e[1 − exp(−kd2t)]
| (2) |
Generally, the washing stage is extremely fast and kw is very large,14 and hence the exp(−kwt) can be ignored and eqn (2) can be simplified as follows:
|
ηt = ηwe + ηd1e[1 − exp(−kd1t)] + ηd2e[1 − exp(−kd2t)]
| (3) |
The extraction rate of flavonoids can be expressed by the equation:
|
ηe = ηwe + ηd1e + ηd2e
| (4) |
|
ηe − ηt = ηd1e exp(−kd1t) + ηd2e exp(−kd2t)
| (5) |
Eqn (5) is applied to fit the experimental data. ηe, which is the equilibrium extraction rate of Scutellaria flavonoids, can be measured experimentally. The equation parameters kd1, kd2, ηd1e and ηd2e can be obtained by fitting with t and (ηe − ηt) by origin 8.0.
3. Results and discussion
3.1 Kinetics of Scutellaria flavonoids extraction
3.1.1 Effect of extraction temperature. First, to study the effect of extraction temperature on GERE and ERE extraction kinetics, the extraction temperature was varied from 30 °C to 70 °C on the rotational speed 270 rpm, and the samples were taken at 1, 2, 3, 4, 5, 10, 20, 30, 40, 50, 60, 80, 100, 120, 150, 180, 240, 300, and 360 min. The other parameters of ERE and GERE were set as the optimal conditions based on our previous investigations.10 Table 1 shows the results of experimental data fitted with eqn (5).
Table 1 Effect of temperature on the kinetic extraction of flavonoids from Scutellaria
T/°C |
ERE |
GERE |
kd1 × 102/min−1 |
kd2 × 102/min−1 |
ηd1e/% |
ηd2e/% |
y0 |
R2 |
Chi^2/DoF × 104 |
kd1 × 102/min−1 |
kd2 × 102/min−1 |
ηd1e/% |
ηd2e/% |
y0 |
R2 |
Chi^2/DoF × 104 |
30 |
9.86 |
0.53 |
68.6 |
9.8 |
−0.012 |
0.999 |
0.29 |
11.42 |
1 |
70.8 |
10.5 |
−0.0024 |
0.999 |
0.77 |
40 |
9.98 |
0.54 |
68.9 |
9.9 |
−0.0109 |
0.999 |
0.31 |
11.55 |
1.03 |
71.4 |
10.8 |
0.0033 |
0.999 |
0.94 |
50 |
10.12 |
0.56 |
69.2 |
9.96 |
−0.0095 |
0.999 |
0.33 |
12.88 |
1.08 |
73.6 |
11.6 |
−0.0197 |
0.999 |
1.95 |
60 |
10.53 |
0.72 |
69.5 |
10.1 |
−0.0038 |
0.999 |
0.38 |
13.6 |
1.11 |
75.9 |
12 |
0.00398 |
0.999 |
0.99 |
70 |
11.32 |
0.96 |
70.9 |
10.3 |
0.00154 |
0.999 |
0.73 |
13.9 |
1.12 |
76.4 |
12.01 |
0.00368 |
0.999 |
1.25 |
As can be seen from Table 1, for both ERE and GERE, the R2 is above 0.99 and the Chi^2/DoF is below 0.02%, indicating that the three-stage model derived from the Fick's second law feasibly describes the extraction rate of Scutellaria flavonoids. Irrespective of ERE or GERE, ηd1e is much larger than ηd2e, indicating that the extraction of total flavonoids of Scutellaria is mainly done in the fast diffusion stage. The results indicated that kd1, kd2, ηd1e and ηd2e by ERE and GERE increased with temperature growth. This is, on one hand, because temperature growth increases the speed of molecular diffusion, enables the increase of permeate, and promotes the diffusion and dissolution of flavonoids. On the other hand, Fick's law shows that the extraction temperature is proportional to the rate of extraction, when the temperature is increased, the solvent viscosity is reduced, which leads to reduced resistance by Scutellaria flavonoids through the solid–liquid interface. This is due to the growth of the mass transfer rate. This is consistent with the result that Mezuane et al.15 found in the ethanol reflux extraction of olive oil that both kd1 and ηd1e increase with temperature increasing. kd1 was 9.86 × 10−2 to 13.9 × 10−2 min−1 at 30–70 °C, similar to that reported in literature16 by ultrasonic extraction of total flavonoids from Prunella vulgaris. From Table 1, it is clear that kd1, kd2, ηd1e and ηd2e by GERE are significantly higher than that by ERE. It may be attributed to GO destruction of the structure of the cell wall,10,13 which reduces the resistance of the diffusion phase and accelerates both the fast diffusion stage and the slow diffusion stage.
3.1.2 Effect of ethanol concentration. The experiments studying the effect of ethanol concentration on the extraction kinetics were performed at a ratio of solvent to raw material of 15 (mL g−1), an extraction temperature of 70 °C, and a dosage of GO for GERE of 1.1 mg g−1.10 The results are shown in Table 2. As can be seen from Table 2, for both ERE and GERE, the kd1, kd2, ηd1e and ηd2e increased with the growth in concentration of aqueous ethanol to the maximum at 60% and then decreased.
Table 2 Effect of ethanol concentration on the kinetic extraction of flavonoids from Scutellaria
C/% |
ERE |
GERE |
kd1 × 102/min−1 |
kd2 × 102/min−1 |
ηd1e/% |
ηd2e/% |
y0 |
R2 |
Chi^2/DoF × 104 |
kd1 × 102/min−1 |
kd2 × 102/min−1 |
ηd1e/% |
ηd2e/% |
y0 |
R2 |
Chi^2/DoF × 104 |
40 |
10.48 |
0.65 |
69.7 |
10.05 |
−0.00501 |
0.999 |
0.43 |
10.8 |
0.67 |
71.5 |
9.79 |
−0.0048 |
0.999 |
0.46 |
50 |
10.88 |
0.84 |
69.8 |
10.12 |
0.00147 |
0.999 |
0.56 |
13.6 |
1.07 |
75.6 |
12.22 |
0.0045 |
0.999 |
0.94 |
60 |
11.32 |
0.96 |
70.9 |
10.3 |
0.00154 |
0.999 |
0.73 |
13.9 |
1.12 |
76.4 |
12.01 |
0.00368 |
0.999 |
1.25 |
70 |
11.01 |
0.94 |
70.3 |
9.92 |
0.00702 |
0.999 |
0.59 |
12.01 |
1.09 |
71.8 |
10.66 |
0.00324 |
0.999 |
0.9 |
The increase in the extraction ability of total flavonoids with the introduction of ethanol to water can be attributed to the increase in permeability of plant tissues, which enables better mass transfer by diffusion. Previous literature17,18 also reported that the optimal ethanol concentration in the extraction of flavonoids from Radix Scutellariae is about 40–75%. When ethanol aqueous solution has similar polarity with Scutellaria's flavonoids, the rate of total flavonoids is higher according to the “like dissolves like” principle. Therefore, it is reasonable that the optimum concentration of ethanol is 60%. From Table 2, the kd1, kd2, ηd1e and ηd2e by GERE at each alcohol concentration are higher than that by ERE, because GO has a synergistic effect on the hydrolysis of cell walls and the leaching of flavonoids with an ethanol solution, which effectively reduces the mass transfer resistance and enables better mass transfer by diffusion.
3.1.3 Effect of GO dosage. The dosage of GO is a key parameter that decides the activity of the catalytic reaction and influences the extraction kinetics. Table 3 shows the effect of GO dosage on the kinetics of Scutellaria flavonoids extraction at 70 °C.
Table 3 Effect of dosage of GO on the kinetic extraction of flavonoids from Scutellaria
GO/mg g−1 |
kd1 × 102/min−1 |
kd2 × 102/min−1 |
ηd1e/% |
ηd2e/% |
y0 |
R2 |
Chi^2/DoF × 104 |
0 |
11.32 |
0.96 |
70.9 |
10.3 |
0.00154 |
0.999 |
0.73 |
0.55 |
13.34 |
0.97 |
72.8 |
11.6 |
0.0031 |
0.999 |
7.36 |
1.1 |
13.9 |
1.12 |
76.4 |
12.01 |
0.00368 |
0.999 |
1.25 |
2.2 |
10.86 |
0.68 |
71.56 |
9.86 |
−0.00428 |
0.999 |
4.84 |
As seen from Table 3, the kd1, kd2, ηd1e and ηd2e first increased with an increase in the GO dosage (g), achieving a maximum at 1.1 mg g−1 and then decreased. Moreover, the acidity of the solvent was significantly enhanced with the addition of GO.10 This is because the addition of GO, on one hand, destroys the cell wall structure, and has a synergistic effect with the ethanol solution; on the other hand, it may lead to more baicalin decomposing into baicalein.1,3,10 Therefore, when the dosage of GO is less than 1.1 mg g−1, the destruction of the cell wall is enhanced with increasing amounts of GO, resulting in reduced resistance of extraction and the growth of the mass transfer rate, thus increasing kd1, kd2, ηd1e and ηd2e. When the amount of added GO exceeded 1.1 mg g−1, the dosage of GO increased baicalin decomposing into baicalein and a lot of glucose, which enhanced the resistance of the flavonoids diffusing to the solvent and decreased the mass transfer rate, thus reducing kd1, kd2, ηd1e and ηd2e. Moreover, as can be observed from the curve between the dosage of GO and pH shown in Fig. 1, the acidity of the solvent is obviously enhanced with the addition of GO. The higher acidity of solvent leads to more baicalin decomposing into baicalein, and hence the yields of total flavonoids decline. It has been reported that baicalin and baicalein are very unstable in strong acidic environment,1,3 which may also cause a decline in the yields of total flavonoids.
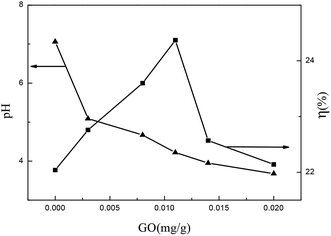 |
| Fig. 1 pH and yields of total flavonoids under different GO dosages. | |
3.1.4 Effect of the ratio of solvent to raw material. The effect of the ratio of solvent to raw material S (mL g−1) on the extraction kinetics is shown in Table 4. The experiments were carried out in the above optimization conditions.
Table 4 Effect of liquid–solid ratio on the kinetic extraction of flavonoids from Scutellaria
S/mL g−1 |
ERE |
GERE |
kd1 × 102/min−1 |
kd2 × 102/min−1 |
ηd1e/% |
ηd2e/% |
y0 |
R2 |
Chi^2/DoF × 104 |
kd1 × 102/min−1 |
kd2 × 102/min−1 |
ηd1e/% |
ηd2e/% |
y0 |
R2 |
Chi^2/DoF × 104 |
10 |
10.25 |
0.57 |
69.6 |
10.1 |
−0.0067 |
0.999 |
0.45 |
12.9 |
0.85 |
69.9 |
10.5 |
−0.0329 |
0.999 |
2.27 |
12 |
10.46 |
0.66 |
69.9 |
10.2 |
−0.0050 |
0.999 |
0.45 |
13.7 |
0.92 |
71.9 |
11.74 |
0.00281 |
0.999 |
0.85 |
15 |
11.32 |
0.96 |
70.9 |
10.3 |
0.00154 |
0.999 |
0.73 |
13.9 |
1.12 |
76.4 |
12.01 |
0.00368 |
0.999 |
1.25 |
18 |
11.74 |
1.1 |
71 |
10.9 |
0.00236 |
0.999 |
0.92 |
12.09 |
1.23 |
71.7 |
11.35 |
0.00338 |
0.999 |
1.02 |
From Table 4, it can be seen that kd1, kd2, ηd1e and ηd2e by ERE increase with the growth of liquid/solid ratio. This is because the growth of S increases the mass transfer interfacial area and mass transfer rate.19 However, for GERE, kd1, kd2, ηd1e and ηd2e first increased with the growth of the liquid/solid ratio up to the maximum at 15 (mL g−1) and then decreased. This is because GO's concentration was reduced when the ratio of solvent to raw material increased, which weakened its catalytic activity and reduced the mass transfer rate.
3.1.5 Effect of the rotating speed. The effect of rotating speed on the extraction kinetics was investigated at rotating speeds ranging between 180 and 350 rpm. The results of the rotating speed experiments are shown in Table 5.
Table 5 Effect of rotational speed on the kinetic extraction of flavonoids from Scutellaria
n/rpm |
ERE |
GERE |
kd1 × 102/min−1 |
kd2 × 102/min−1 |
ηd1e/% |
ηd2e/% |
y0 |
R2 |
Chi^2/DoF × 104 |
kd1 × 102/min−1 |
kd2 × 102/min−1 |
ηd1e/% |
ηd2e/% |
y0 |
R2 |
Chi^2/DoF × 104 |
180 |
10.35 |
0.65 |
70.41 |
9.88 |
−0.0087 |
0.999 |
1.07 |
12.9 |
1.02 |
72.4 |
14.8 |
0.00346 |
0.999 |
0.36 |
270 |
11.32 |
0.96 |
70.9 |
10.3 |
0.00154 |
0.999 |
0.73 |
13.9 |
1.12 |
76.4 |
12.01 |
0.00368 |
0.999 |
1.25 |
350 |
11.44 |
0.98 |
70.95 |
10.55 |
0.00193 |
0.999 |
0.82 |
13.67 |
1.27 |
76.3 |
12.1 |
0.0052 |
0.999 |
0.94 |
Generally,20 the rotating speed affects the extraction process, which is controlled only by diffusion or both diffusion and chemical reaction. However, for the extraction process controlled by the internal reaction, the extraction rate has nothing to do with the rotating speed. Table 5 shows that irrespective of ERE or GERE kd1, kd2, ηd1e and ηd2e show little change with rotating speed, which means that rotating speed has little effect on the extraction kinetics, further indicating that the extraction process of total flavonoids is controlled by the inner diffusion.
3.2 Apparent diffusion coefficient (D′) and the activation energy (Ea)
As seen, the Scutellaria particles sieved to 10–20 mesh are not spherical but cylindrical, and it can be assumed that the apparent diffusion coefficient of each stage on the extraction process is consistent with the apparent diffusion coefficient of Fick's second law based on the model proposed by Kang et al.21 |
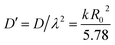 | (6) |
The extraction of D′ by Scutellaria's total flavonoids can be calculated according to Table 1 and eqn (6), and the results are shown in Table 6. From Table 6, it can be observed that the value of D′ is in the range 3.57–5.03 × 10−12/(m2 s−1). The ADC from GERE has not been reported in the literature. Zhao et al.22 reported that the ADC of polysaccharides ranged from 10−12 to 10−11/(m2 s−1), which is similar to our experimental data. Generally,20 the ADC (D) of rhizome is about 10−11/(m2 s−1), for example, the D of glycyrrhizic acid extracted by the aqueous ammonia method from licorice root was 5.1 × 10−11/(m2 s−1), the D of valerian in valerian root extracted by ethanol was 0.82 × 10−11/(m2 s−1).
Table 6 Expansive diffusion coefficient in rapid diffusion stage D′ × 1012/(m2 s−1)
T/°C |
ERE |
GERE |
30 |
3.57 |
4.13 |
40 |
3.61 |
4.18 |
50 |
3.66 |
4.66 |
60 |
3.81 |
4.92 |
70 |
4.09 |
5.03 |
As we know, D′ is related to not only temperature but also the particle size. It is assumed that the correction coefficient λ of the mass transfer path is independent of temperature, and the relationship between λ and R0 is λ = mR0−n.23 The mass transfer path of GERE changes because GO damage to the cell wall of Scutellaria changes the cell permeability. The effect of GO depends on temperature,8,10,12 and hence λ is not only related to R0, but also related to temperature. Therefore, it can be assumed that the relationship between λ and temperature and particle size is
|
λ = (mR0−n)/(T − Tc)2θ
| (7) |
The relationship between the diffusion coefficient D and the extraction temperature follows the Arrhenius formula
|
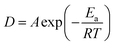 | (8) |
Hence, the diffusion coefficient D′ of ERE and GERE can be obtained as follows:
|
 | (9) |
|
 | (10) |
Take the logarithm on both sides of (9) and (10)
|
ERE: ln D′ = ln(A/m2) − Ea/(RT) + 2n ln R0
| (11) |
|
GERE: ln D′ = ln(A/m2) − Ea/(RT) + 2n ln R0 + 2θ ln(T − Tc)2
| (12) |
ln
D′ and 1/T were linearly analyzed according to the data of Table 6 and the formulas (11) and (12), and the relationship between D′ and T was obtained as follows when ERE was extracted at 30–70 °C:
|
ln D′ = ln(A/m2) − 2.45/T + 2n ln R0
| (13) |
For GERE (the dosage of GO is 1.1 mg g−1), the fitting equation is
|
ln D′ = ln(A/m2) − 1.69/T + 2n ln R0 + 2θ ln(T − Tc)2
| (14) |
Calculated from the above two formulas, Ea was 20.37 kJ mol−1 for ERE and 13.2 kJ mol−1 for GERE. This further indicated that the addition of GO greatly accelerates the progress of the reaction.
4. Conclusion
The extraction process of total flavonoids from Scutellaria baicalensis is well described by a three-stage model based on Fick's second law. The extraction rate increased with the growth of GO dosage, ethanol concentration and liquid–solid ratio at 30–70 °C, and the rotating speed had little effect. This result is highly consistent with our previous study on single factor experiments.10 In this study, the effective internal diffusion coefficient D′ of flavonoids extracted by ERE and GERE was studied. The results showed that, for ERE, ln
D′ = ln(A/m2) − 2.45/T + 2n
ln
R0 and Ea = 20.37 kJ mol−1, and for GERE, ln
D′ = ln(A/m2) − 1.69/T + 2n
ln
R0 + 2θ
ln(T − Tc)2 and Ea = 13.2 kJ mol−1, which was 35.2% lower than that by ERE. At 30–70 °C, the apparent diffusion coefficient (D′) of GERE extraction is 4.13–5.03 × 10−12/(m2 s−1), and the D′ of ERE is 3.57–4.09 × 10−12/(m2 s−1).
Conflicts of interest
There are no conflicts to declare.
References
- H. B. Li, Y. Jiang and F. Chen, Separation methods used for Scutellaria baicalensis active components, J. Chromatogr. B: Anal. Technol. Biomed. Life Sci., 2004, 812, 277–290 CrossRef CAS.
- L. Wang, Y. Ling, Y. Chen, C. L. Li, F. Feng, Q. D. You, N. Lu and Q. L. Guo, Flavonoid baicalein suppresses adhesion, migration and invasion of MDA-MB-231 human breast cancer cells, Cancer Lett., 2010, 297, 42–48 CrossRef CAS PubMed.
- L. L. Dong, Y. J. Fu, Y. G. Zu, M. Luo, W. Wang, X. J. Li and J. Li, Application of cavitation system to accelerate the endogenous enzymatic hydrolysis of baicalin and wogonoside in Radix Scutellariae, Food Chem., 2012, 131, 422–429 CrossRef.
- Z. H. Gao, K. X. Huang, X. L. Yang and H. B. Xu, Free radical scavenging and antioxidant activities of flavonoids extracted from the radix of Scutellaria baicalensis Georgi, Biochim. Biophys. Acta, 1999, 1472, 643–650 CrossRef CAS.
- J. J. Liu, T. S. Huang, W. F. Cheng and F. J. Lu, Baicalein and baicalin are potent inhibitors of angiogenesis: inhibition of endothelial cell proliferation, migration and differentiation, Int. J. Cancer, 2003, 106, 559–565 CrossRef CAS PubMed.
- F. Zhou, Extraction of Total Flavonoids from Scutellaria baicalensis Georgi and Separation of Baicalin, Wuhan University of Engineering, Wuhan, 2007 Search PubMed.
- G. C. Chen, et al., Study on Microwave-assisted Extraction of Baicalin from Scutellaria baicalensis, J. Chem. Ind. Eng., 2005, 25, 81–83 Search PubMed.
- M. S. Featherand and J. F. Harris, The acid-catalyzed hydrolysis of glycopyranosides, Hydrolysis Glucopyranose, 1965, 30, 153–157 Search PubMed.
- B. Ismail and K. Hayes, Beta-glycosidase activity toward different glycosidic forms of isoflavones, J. Agric. Food Chem., 2005, 53, 4918–4924 CrossRef CAS PubMed.
- X. Wang and F. Wei, et al., Application of graphene oxide as a catalyst to accelerate extraction of total flavonoids and the hydrolysis of baicalin from Radix Scutellaria, Sep. Purif. Technol., 2014, 133, 421–428 CrossRef.
- G. C. So and D. G. Macdonald, Kinetics of oil extraction from canola (rapeseed), Can. J. Chem. Eng., 1986, 64(1), 80–86 CrossRef.
- D. C. Marcano, D. V. Kosynkin, D. C. Marcano, D. V. Kosynkin, J. M. Berlin, A. Sinitskii, Z. Z. Sun, A. Slesarev, B. L. Alemany, W. Lu and J. M. Tour, Improved synthesis of graphene oxide, ACS Nano, 2010, 4, 4806–4814 CrossRef CAS PubMed.
- Y. Wang, Z. Li, J. Wang, J. Li and Y. Lin, Graphene and graphene oxide: biofunctionalization and applications in biotechnology, Trends Biotechnol., 2011, 29, 205–212 CrossRef CAS PubMed.
- A. R. Linares, S. L. Hase and M. L. Vergara, et al., Modeling yerba mate aqueous extraction kinetics: Influence of temperature, J. Food Eng., 2010, 97(4), 471–477 CrossRef.
- S. Mezuane and H. Kadi, Kinetics and thermodynamics of oil extraction from olive cake, J. Am. Oil Chem. Soc., 2008, 85(4), 391–396 CrossRef.
- H. T. Zhu, C. Chao, L. Chen and Q. B. Wang, Study on extraction kinetics of total flavonoids from Prunella vulgaris, Journal of Chinese Pharmacists, 2012, 15(4), 459–461 CAS.
- Y. C. Yang, M. C. Wei, T. C. Huang, S. Z. Lee and S. S. Lin, Comparison of modified ultrasound-assisted and traditional extraction methods for the extraction of baicalin and baicalein from Radix Scutellariae, Ind. Crops Prod., 2013, 45, 182–190 CrossRef CAS.
- L. L. Dong, Y. J. Fu, Y. G. Zu, J. Li, X. J. Li and T. Efferth, Negative pressure cavitation accelerated processing for extraction of main bioactive flavonoids from Radix Scutellariae, Chem. Eng. Process., 2011, 50, 780–789 CrossRef CAS.
- L. Feng, S. Zhang and Z. Liu, Graphene based gene transfection, Nanoscale, 2011, 3, 1252–1257 RSC.
- S. F. Li and Z. Y. Jiang, Advanced Pharmaceutical Separation Engineering, Chemical Industry Press, Beijing, 2008, pp. 8–47 Search PubMed.
- J. S. Kang, Study on enzymatic extraction of Astragalus polysaccharides, Hefei University of Technology, Hefei, 2012 Search PubMed.
- P. Zhao, Study on extraction and purification of polysaccharide from Coltsfoot, Northwestern Polytechnical University, Shaanxi, 2010 Search PubMed.
- X. D. He, S. H. Liu and G. L. Li, et al., Preparation of oxidized graphene and its catalytic hydrolysis of soybean isoflavones, J. Chem. Eng. Chin. Univ., 2012, 26(1), 56–60 CAS.
|
This journal is © The Royal Society of Chemistry 2017 |