DOI:
10.1039/C7RA04347C
(Paper)
RSC Adv., 2017,
7, 39564-39575
Further enhanced oil recovery by branched-preformed particle gel/HPAM/surfactant mixed solutions after polymer flooding in parallel-sandpack models
Received
18th April 2017
, Accepted 1st August 2017
First published on 14th August 2017
Abstract
How to further sweep residual oil from unswept areas is crucial to enhance oil recovery after polymer flooding, which is widely used. Branched-preformed particle gel (B-PPG) is a newly developed chemical agent for enhanced oil recovery in heterogeneous reservoirs. In this paper, B-PPG/HPAM/surfactant mixed solutions were investigated to further enhance oil recovery after polymer flooding in parallel-sandpack models by core flood test. First of all, laboratory experiments about fractional flow and enhanced oil recovery were performed to determine the optimal composition and concentration of B-PPG and HPAM mixed solutions. The results show that B-PPG/HPAM mixed solutions have higher abilities to adjust fractional flow in parallel-sandpack models than HPAM alone when the mass percentage of B-PPG is larger than 50%. Moreover, B-PPG/HPAM mixed solutions can displace more oil in low permeability sandpacks and the total oil recovery is the highest when the mass percentage of B-PPG is 50%. The concentration also has a great effect on fractional flow and oil recovery of B-PPG/HPAM mixed solutions. The ability of adjusting fractional flow and enhancing oil recovery increases with increase in concentration. Furthermore, the presence of surfactant in the flooding solutions can further enhance total oil recovery, especially that in high permeability sandpacks, and has no obvious effect on the ability to adjust fractional flow. When the permeability ratio of the parallel sandpacks becomes 1
:
9, the B-PPG/HPAM/surfactant mixed solutions still have strong abilities to adjust fractional flow and enhance the oil recovery. This is because the presence of a surfactant can improve displacement efficiency, while B-PPG and HPAM can enhance sweep efficiency.
1. Introduction
Polymer flooding has drawn more and more attention since hydrolyzed polyacrylamide was found to reduce the mobility of brine and enhance oil recovery.1,2 To date, polymer flooding has been applied successfully in Daqing and Shengli oilfields in China, in Canada and in other countries.3–6 For example, polymer flooding can enhance heavy-oil recovery by more than 25% of oil originally in place (OOIP) in Pelican Lake oilfield in northern Alberta.7
Usually, polymer flooding can improve swept volume to enhance oil recovery by about 10% compared with water flooding because of improved viscosity and viscoelasticity of polymer solutions.8–10 Furthermore, elasticity of polymer imposes a pulling effect on the trapped oil at dead ends and leads to mobilization the trapped oil.11
However, almost half of the geological reserves still remain in the original reservoirs even after polymer flooding.12 Polymer flooding cannot sweep all the residual oil because the conflicts of in-layer or interlayer formation heterogeneity are the most influential factors during the development of reserves using polymer flooding.13,14 Consequently, polymer fingering or channeling phenomena occur when injected polymer solution enters high permeability zones. Therefore, new methods are of crucial significance to further enhance oil recovery after polymer flooding.15
After polymer flooding, many technologies, such as polymer–surfactant flooding,16,17 alkaline-surfactant-polymer flooding,18,19 foam flooding,20–22 and gel treatment,23 have been developed to further enhance oil recovery by improving the swept volume and increasing displacement efficiency. Of these, gel treatment is one of the most effective methods to reduce permeability of reservoirs with fractures or channels and control water mobility in heterogeneous reservoirs.24–27
Qiao et al.28 present a novel modified cationic starch that can react with partially hydrolyzed polyacrylamide (HPAM) remaining in reservoirs to form gels in situ. The obtained gels can reduce polymer channeling and transform successive injected water into mid-low permeability zones that achieve the purpose of deep profile control. The singular sand-pack core flood test results provide experimental evidence that the formed gel system is more effective in plugging high permeability pore passages. Moreover, the parallel core flood tests show that this gel system can significantly enhance oil recovery and effectively reduce water cut.
Feng et al.29 have carried out laboratory studies on multi-profile control and displacement systems to enhance oil recovery after polymer flooding. These systems comprise a gel particle, cross-linking agent and highly effective surfactant. Profile control experiments on the multiple systems after polymer flooding are separately carried out to verify the potential of enhanced oil recovery. The results show that the multiple injection system not only blocks high permeable layers and channels, but also makes full use of medium and low permeability layers, eventually improving the overall sweep efficiency. Owing to surfactant injection, displacement efficiency is simultaneously improved to some extent, which greatly improves oil recovery after polymer flooding.
Therefore, the utilization of gel to control water mobility is an important method to further enhance oil recovery after polymer flooding. Recently, a novel branched-preformed particle gel (B-PPG) has been developed and applied in oilfields.30–32 B-PPG is a preformed particle gel with some branched chains and can increase the viscosity of the suspension. The size of a single B-PPG particle is between 5 and 10 μm, and a large number of particles gather in a high-concentration B-PPG suspension. B-PPG particles reflect a more elastic nature of the gelled system and have the capacity of elastic deformation. Viscoelastic B-PPG particles can change their shape during displacement to pass through pore throats and revert to their original shape after entering a larger pore. With the ability to plug pore throats of different diameters, dynamically and alternatively, B-PPG can adjust flows in different zones of a heterogeneous medium and improve the sweeping efficiency significantly.32
However, B-PPG has a weaker effect on viscosity increase of the flooding solution than HPAM. HPAM is usually used to increase the viscosity of the flooding solution to enhance oil recovery. Neither B-PPG nor HPAM can only increase the swept volume after polymer flooding. Except for the sweep efficiency, displacement efficiency is also important to oil recovery. As is well known, surfactants can decrease the interface tension between oil and water and change the wettability of the rock surface to improve displacement efficiency.
In this paper, B-PPG/HPAM/surfactant mixed solutions were investigated to further enhance oil recovery after polymer flooding in parallel-sandpack models by core flood test. The effect of composition and concentration of B-PPG and HPAM mixed solutions on fractional flow and oil recovery were investigated to determine the optimal B-PPG/HPAM mixed solution. Then the ability of B-PPG/HPAM/surfactant mixed solutions on fractional flow and oil recovery in parallel-sandpack models with different permeability ratios were investigated. The aim of the work is to provide a new method to further enhance oil recovery after polymer flooding in heterogeneous reservoirs.
2. Experimental
2.1 Materials
Branched-preformed particle gel (B-PPG), hydrolyzed polyacrylamide (HPAM), surfactant, and crude oil samples were provided from Geological Scientific Research Institute of Sinopec Shengli Oilfield Company, SINOPEC. The intrinsic viscosity and hydrolyzed degree of HPAM were 2658 mL g−1 and 20.4%, respectively. The surfactant was petroleum sulfonate with actual active ingredient content of 90%. The crude oil sample from a Shengli oil reservoir was already divested of water and gas. At a reservoir temperature of 70 °C, the oil had a density of 0.915 g cm−3 and a viscosity of 37.4 mPa s. The simulated formation water was prepared in laboratory by NaCl, CaCl2, and MgCl2·H2O purchased from Sinopharm Chemical Reagent Co., Ltd (SCRC). The compositions of the simulated formation water are shown in Table 1. The chemical agents were used without further purification. Certain amounts of B-PPG, HPAM, and surfactants were weighted and dissolved in simulated formation water with the help of stirring for 2 hours. No precipitate was found during the preparation of the mixed solutions.
Table 1 Compositions of simulated formation water
Compositions (mg L−1) |
Total salinity (mg L−1) |
Na+ |
Mg2+ |
Ca2+ |
Cl− |
3419 |
51 |
206 |
5826 |
9502 |
2.2 Core flood test
Fig. 1 shows the schematic of parallel-sandpack tests. The displacement tests were performed by using parallel-packed sandpack holders of 30.0 cm length and 2.5 cm diameter. Both ends of the sandpack holders were equipped with fluid distributors, on which a 200 mesh stainless steel screen was spot-welded to prevent fine sand from flowing out and to provide a more even distribution of injected fluid. The sandpack flood tests were conducted at 70.0 °C in a temperature control box. The coarse (40–60 mesh) and fine sand (100–120 mesh) were packed inside separate sandpack holders to get sandpacks with high and low permeability, respectively. For each test, the fresh sand was packed in the sandpack holders then pressed for 2 minutes by a mechanical hydraulic pump. The required absolute permeability could be obtained by adjusting the mesh of the sand and applied pressure. The permeability of the sandpack with low permeability in the parallel-sandpacks is about 1.0 μm2. The permeability of the sandpack with high permeability is different with the permeability ratio of the both sandpacks. The permeability ratio of the both sandpacks in the parallel-sandpacks is 1
:
3 with no special instruction.
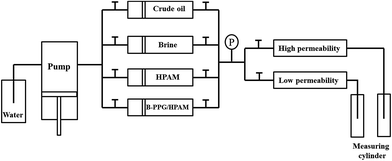 |
| Fig. 1 Schematic of parallel-sandpack tests. | |
The pore volume of the porous media was measured using by subtracting the volume of the sand in the core holder from that of the sandpack holder. The weight of the sand loaded into the sandpack holder was recorded and the volume of the material was determined accurately for each experiment. The porosity could be calculated by the ratio of pore volume to total volume of the sandpack holder.
The sandpacks with high and low permeability were separately saturated with brine and then with crude oil. The crude oil was injected continuously until water cut was less than 2.0%. After saturation, the parallel sandpacks underwent initial water flooding until the water cut was larger than 98%. After that, a 1500 mg L−1 HPAM solution of 0.5 PV was injected and water flooding was continued until the water cut in the produced liquid was larger than 98%. Then, 0.5 PV B-PPG/HPAM mixed solution was injected in the parallel sandpacks and followed by water flooding until the water cut was larger than 98%. In the entire displacement process, the injection rate of the displacing fluids was controlled at 0.5 mL min−1. Fractional flows and oil production of both sandpacks with high and low permeability were measured at regular intervals. The enhanced oil recovery by B-PPG/HPAM mixed solution injection and extended water flooding was adopted to evaluate the efficiency of different slug. The pressure drop across the sandpacks during the displacement was monitored by a digital pressure gauge.
2.3 Viscosity measurements
The experimental solutions of B-PPG/HPAM and B-PPG/HPAM/surfactant were prepared by mechanical stirring at ambient temperature (25 °C). The viscosity measurements were carried out on DV-ii ultra-programmable rheometer (Brookfield Company (U.S.A.)) at temperatures of 70.0 ± 0.1 °C.
3. Results and discussion
3.1 Effects of mass ratio of B-PPG and HPAM on fractional flow and oil recovery
B-PPG and HPAM have different effects on improving the swept volume. B-PPG can block the water channel and divert flow to the low permeability zones, while HPAM can increase viscosity of the flooding solution and enhance flow resistance. In order to know if B-PPG and HPAM have synergistic effects in the mixed solutions, B-PPG/HPAM mixed solutions with different mass ratios were first investigated. Fig. 2 shows fractional flows in high and low permeability sandpacks for different B-PPG/HPAM mixed solutions with a total concentration of 2500 mg L−1. It can be seen that during the water flooding period, the flow in the high-permeability sandpack first decreases and then increases, while the flow in the low-permeability sandpack first increases and then decreases gradually. After about 0.5 PV water flooding, the fractional flow (high permeability
:
low permeability) ratio through the two sandpacks is 100
:
0, i.e., there is nearly no flow in the low-permeability sandpack. During HPAM flooding, fractional flow in the high-permeability sandpack decreases, while that in the low-permeability sandpack increases. After subsequent water flooding, fractional flow ratio becomes 100
:
0 again. Then, during 2500 mg L−1 HPAM flooding of 0.3 PV (shown in Fig. 2A), fractional flow in the high-permeability sandpack decreases greatly, while that in the low-permeability sandpack increases quickly. This means the increase of HPAM concentration from 1500 to 2500 mg L−1 can change the mobility ratio and further enhance fractional flow in the low-permeability sandpack. During the subsequent water flooding, fractional flow in the high-permeability sandpack increases, while that in the low-permeability sandpack decreases. Fig. 2B shows fractional flow in high- and low-permeability sandpacks for 25% B-PPG and 75% HPAM mixed solution with a total concentration of 2500 mg L−1. B-PPG/HPAM mixed solutions have a higher ability to adjust flow between both sandpacks with different permeability. This phenomenon is more and more clear with the increase in B-PPG concentration, as shown in Fig. 2C to E. During B-PPG/HPAM flooding, fractional flow in the high-permeability sandpack decreases greatly, while that in the low-permeability sandpack increases quickly. It is clear that the two fractional flow curves fluctuate alternately. In this period, fractional flow in the low-permeability sandpack is higher than that in the high-permeability sandpack. During subsequent water flooding, the two fractional flow curves also fluctuate. The fractional flow ratio through the two sandpacks is stable at about 60
:
40 during subsequent water flooding. The above fractional flow behaviors of B-PPG/HPAM mixed solution in parallel sandpack tests illustrate that B-PPG has a better property than HPAM to adjust fractional flows in different zones of a heterogeneous medium and improve the swept efficiency. The results also show that B-PPG and HPAM have a synergistic effect to adjust fractional flow.
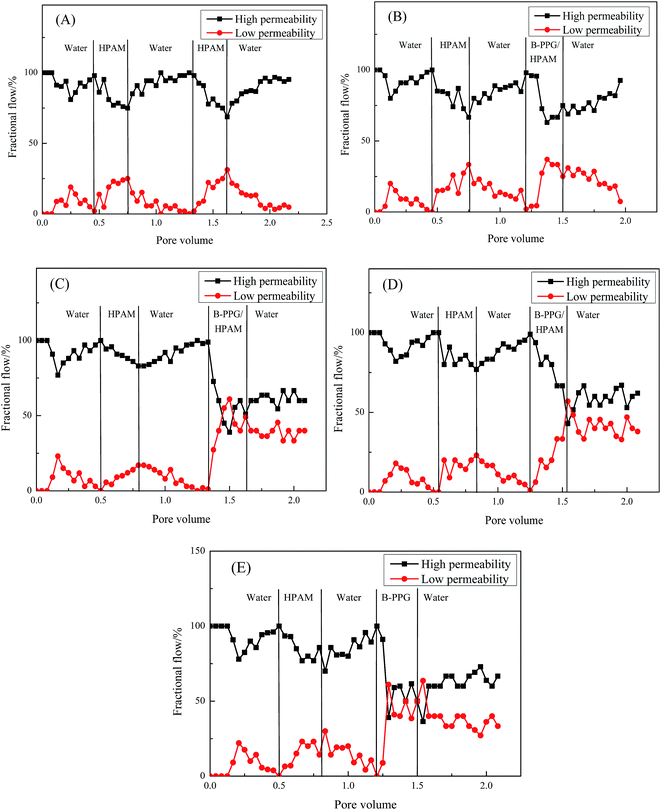 |
| Fig. 2 Fractional flows in high and low permeability sandpacks for different B-PPG/HPAM mixed solutions with a total concentration of 2500 mg L−1. The mass percentages of B-PPG in the mixed solutions of (A)–(E) are 0, 25%, 50%, 75%, and 100%, respectively. | |
The results show that B-PPG and HPAM mixed solutions can adjust fractional flow ratio in the two sandpacks to about 60
:
40 during the period of B-PPG/HPAM flooding and subsequent water flooding when the percentage of B-PPG in the mixed solutions is larger than 50%. Fractional flow includes produced oil and flooding solutions; therefore, the results can reflect the adjustment of flow between the high- and low-permeability sandpacks, not oil recovery. In order to evaluate the ability of enhanced oil recovery, the cumulative oil recoveries of the parallel sandpacks for different B-PPG/HPAM mixed solutions are measured and shown in Fig. 3. During the water flooding period, oil recoveries in both sandpacks increase greatly and the high-permeability sandpack has a larger oil recovery. During HPAM flooding, oil recovery in the low-permeability sandpack continues increasing, while that in the high-permeability sandpack increases slowly. After subsequent water flooding, oil recoveries in both sandpacks increase quickly again. When 2500 mg L−1 HPAM is flooded, oil recovery in the low-permeability sandpack continues increasing, while that in the high-permeability sandpack no longer increases. Oil recovery enhanced by subsequent HPAM and water flooding is much less than that of the former HPAM and water flooding. When a B-PPG/HPAM mixed solution is injected, oil recovery in the low-permeability sandpack sequentially increases, while that in the high-permeability sandpack slightly increases. The oil recovery enhanced by the B-PPG/HPAM mixed solution is much larger than that by HPAM with the same concentration. Table 2 shows oil recoveries of core flood tests with 2500 mg L−1 B-PPG/HPAM mixed solutions. It is clear that oil recovery in the low-permeability sandpack can be enhanced by 20–30% and the total oil recovery can be enhanced by 10–15% by injection of B-PPG/HPAM mixed solutions after polymer flooding. When the percentage of B-PPG is 50%, total oil recovery can be enhanced by 14.9% and oil recovery in the high-permeability sandpack can also be enhanced by 1.5%. That is, 50% B-PPG and 50% HPAM mixed solution has the highest effect on oil recovery enhancement. This means B-PPG and HPAM have a synergistic effect to enhance the oil recovery, especially oil recovery in the low-permeability sandpack.
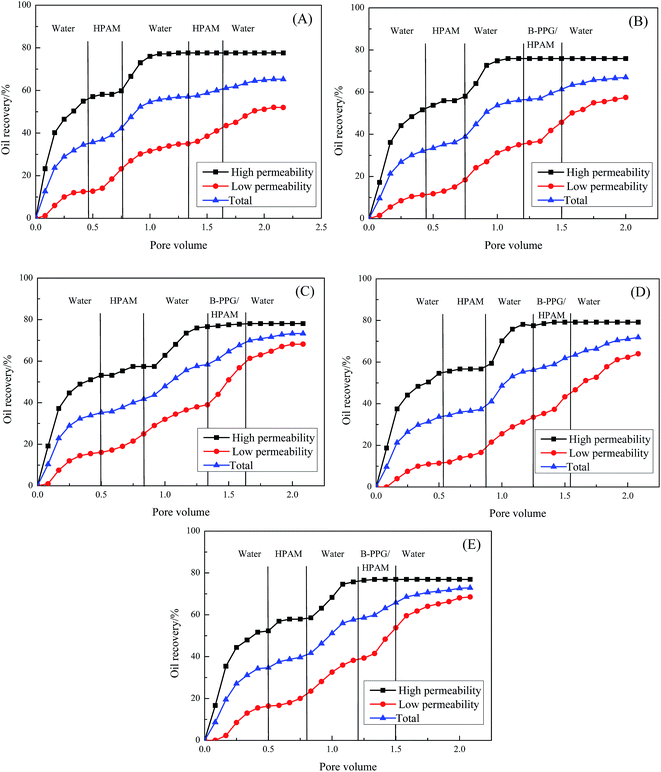 |
| Fig. 3 Cumulative oil recovery of the parallel sandpacks for different B-PPG/HPAM mixed solutions with a total concentration of 2500 mg L−1. The mass percentages of B-PPG in the mixed solutions of (A)–(E) are 0, 25%, 50%, 75%, and 100%, respectively. | |
Table 2 Summary of core flood tests with 2500 mg L−1 B-PPG/HPAM mixed solutions
Mass percentage of B-PPG in the mixed solution |
Sandpacks |
Oil recovery after water flooding/% |
Oil recovery after HPAM flooding/% |
Oil recovery enhanced by HPAM flooding/% |
Oil recovery after B-PPG/HPAM flooding/% |
Oil recovery enhanced by B-PPG/HPAM flooding/% |
0% |
High permeability |
57.1 |
77.6 |
20.5 |
77.6 |
0.0 |
Low permeability |
12.7 |
35.0 |
22.3 |
52.0 |
17.0 |
Total |
35.7 |
57.0 |
21.3 |
65.3 |
8.3 |
25% |
High permeability |
53.8 |
75.9 |
22.2 |
75.9 |
0.0 |
Low permeability |
11.8 |
36.7 |
24.9 |
57.5 |
20.8 |
Total |
33.2 |
56.8 |
23.6 |
67.0 |
10.2 |
50% |
High permeability |
53.2 |
76.6 |
23.4 |
78.1 |
1.5 |
Low permeability |
16.1 |
39.0 |
22.9 |
68.2 |
29.2 |
Total |
35.3 |
58.9 |
23.1 |
73.3 |
14.9 |
75% |
High permeability |
54.6 |
78.5 |
23.9 |
79.2 |
0.7 |
Low permeability |
11.4 |
34.5 |
23.1 |
64.0 |
29.5 |
Total |
33.7 |
57.0 |
23.3 |
71.8 |
14.8 |
100% |
High permeability |
52.3 |
76.9 |
24.6 |
76.9 |
0.0 |
Low permeability |
16.4 |
39.2 |
22.8 |
68.5 |
29.3 |
Total |
34.7 |
58.1 |
23.9 |
72.9 |
13.8 |
Viscosity is an important factor to improve sweep efficiency of polymer flooding.6,33,34 Fig. 4 shows the variations in viscosity and oil recovery enhanced by B-PPG and HPAM mixed solutions with different B-PPG mass percentages. Viscosity decreases with the increase in B-PPG mass percentage, which indicates that B-PPG has a weaker effect viscosity enhancement than HPAM. Meanwhile, enhanced oil recovery first increases and then decreases slightly. These results show that B-PPG/HPAM mixed solutions have stronger effects on enhanced oil recovery than HPAM, though the mixed solutions have lower viscosities than HPAM. B-PPG can increase the swept volume by blocking the water channel and diverting the flow to unswept low-permeability zones, while HPAM can enhance the swept volume just by increasing the viscosity of the flooding solution. The 50% B-PPG/50% HPAM mixed solution has the highest enhanced oil recovery because the suitable viscosity of the mixed solutions can further improve sweep efficiency. Therefore, B-PPG and HPAM have a synergistic effect to adjust fractional flow and enhance oil recovery.
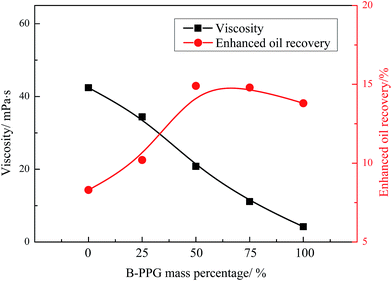 |
| Fig. 4 Variations in viscosity and total oil recovery by B-PPG/HPAM mixed solutions with different B-PPG mass percentages. | |
3.2 Effects of concentration of B-PPG and HPAM mixed solutions on fractional flow and oil recovery
The 50% B-PPG and 50% HPAM mixed solution with a total concentration of 2500 mg L−1 has a higher oil recovery than B-PPG or HPAM alone. Meanwhile, the application of 50% B-PPG and 50% HPAM mixed solution can decrease the cost compared with B-PPG alone. Furthermore, the effects of B-PPG and HPAM concentration on fractional flow and enhanced oil recovery have also been investigated. Fig. 5 shows fractional flows in high- and low-permeability sandpacks for different B-PPG/HPAM mixed solutions. When the concentration of B-PPG and HPAM is lower than 2000 mg L−1, fractional flow in the high-permeability sandpack decreases during 0.3 PV B-PPG/HPAM flooding and fractional flow decreases with increase in B-PPG/HPAM concentration. After B-PPG/HPAM flooding, fractional flow in the high-permeability sandpack increases during subsequent water flooding. When the concentration of B-PPG and HPAM is higher than 2500 mg L−1, fractional flows in both sandpacks fluctuate alternately. Fractional flow in the low-permeability sandpack is even higher than that in the high-permeability sandpack. Moreover, after B-PPG/HPAM flooding, fractional flows in both sandpacks maintain stable values during subsequent water flooding. The results indicate that the effect of adjusting fractional flows both sandpacks is enhanced with an increase in B-PPG/HPAM concentration.
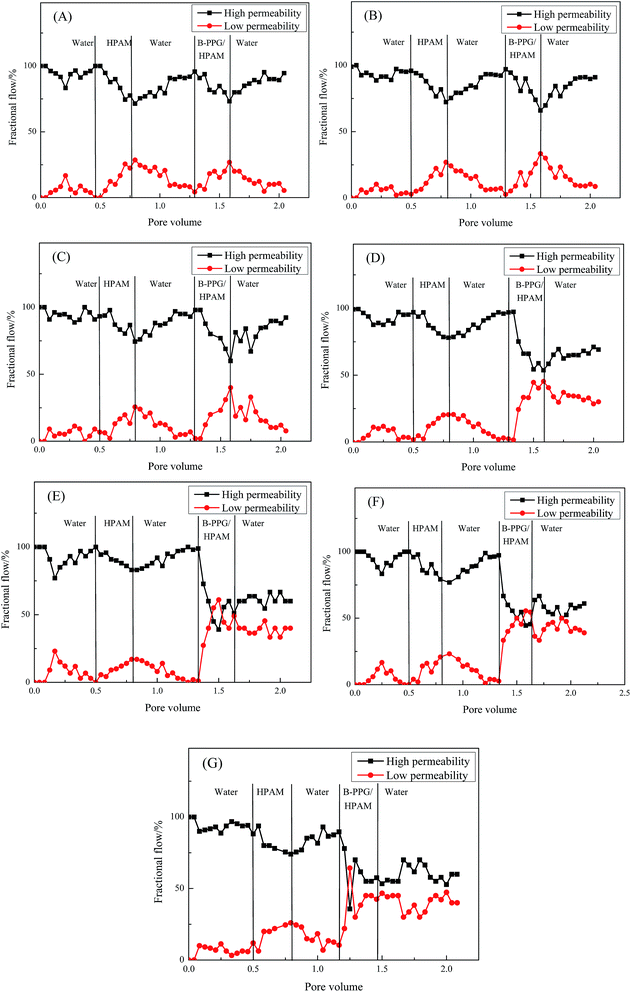 |
| Fig. 5 Fractional flows in high- and low-permeability sandpacks for different concentrations of B-PPG/HPAM mixed solutions. The total concentration of B-PPG/HPAM mixed solutions of (A)–(G) are 1500, 1750, 2000, 2250, 2500, 3000, and 3500 mg L−1. The mass percentage of B-PPG in the mixed solutions is 50%. | |
Fig. 6 shows oil recovery enhancement by B-PPG/HPAM mixed solutions with different concentrations. Oil recoveries in the high- and low-permeability sandpacks and total oil recovery all increase with increase in B-PPG/HPAM concentration. Oil recovery enhancement in the low permeability sandpack is much larger than that in the high-permeability one. With increase in B-PPG/HPAM concentrations, the effect of adjusting fractional flow in both sandpacks is enhanced and oil recoveries are improved, especially in the low-permeability sandpack. The curve of total oil recovery vs. concentration is in the shape of an S. Oil recovery increases slightly with increase in concentration when the concentration is lower than 2000 mg L−1. When the concentration is from 2000 to 2500 mg L−1, total oil recovery increases rapidly, and then beyond 2500 mg L−1, oil recovery increases slowly. Meanwhile, Fig. 6 also shows viscosity variation of B-PPG/HPAM mixed solutions with concentration. The viscosity of B-PPG/HPAM mixed solutions increases with concentration increase. The variation in oil recovery vs. concentration is not consistent with the curves of viscosity vs. concentration. Therefore, 2500 mg L−1 is the optimal concentration for B-PPG/HPAM mixed solutions to enhance oil recovery after polymer flooding.
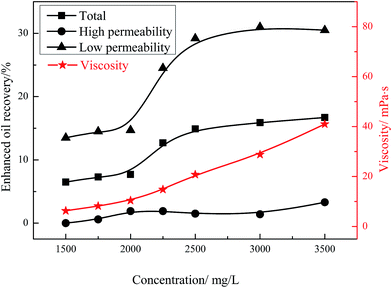 |
| Fig. 6 Enhanced oil recovery and viscosity variations in B-PPG/HPAM mixed solutions of different concentrations. The mass percentage of B-PPG in the mixed solutions is 50%. | |
3.3 Effects of surfactant on fractional flow and oil recovery of B-PPG and HPAM
Oil recovery is equal to sweep efficiency multiplied by displacement efficiency. B-PPG and HPAM have a synergistic effect on oil recovery enhancement by improving the swept volume. In order to improve displacement efficiency, a surfactant is added to B-PPG/HPAM mixed solutions. Fig. 7 shows fractional flow in the high- and low-permeability sandpacks for different B-PPG/HPAM/surfactant mixed solutions. Fractional flows in both sandpacks fluctuate alternately during B-PPG/HPAM/surfactant flooding. Fractional flow in the low-permeability sandpack is higher than that in the high-permeability sandpack. Furthermore, after B-PPG/HPAM/surfactant flooding, fractional flows in both sandpacks can maintain stable values during subsequent water flooding. B-PPG/HPAM/surfactant mixed solutions also have an excellent ability to adjust fractional flows in the high- and low-permeability sandpacks. The increase in surfactant concentration has no obvious effect on fractional flows in the high- and low-permeability sandpacks.
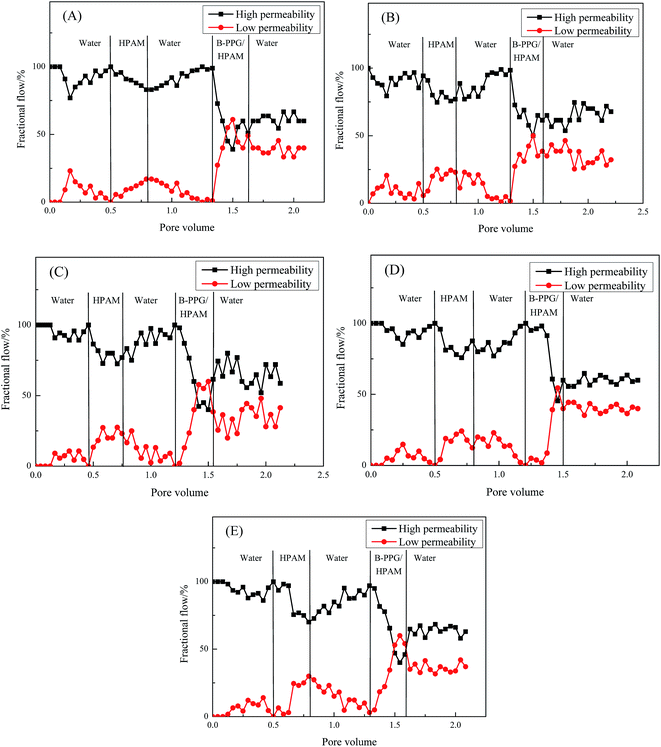 |
| Fig. 7 Fractional flows in high- and low-permeability sandpacks for different B-PPG/HPAM/surfactant mixed solutions. The concentrations of B-PPG and HPAM in the mixed solutions are 1250 and 1250 mg L−1. The concentrations of surfactant in the mixed solutions of (A) to (E) are 0.10%, 0.20%, 0.30%, 0.40%, and 0.50%, respectively. | |
Fig. 8 shows enhanced oil recoveries of core flood tests with different B-PPG/HPAM/surfactant mixed solutions. When surfactant concentration is lower than 0.3%, oil recovery enhancement in the high-permeability sandpack increases, while that in the low-permeability sandpack has no obvious increasing trend. When the concentration is higher than 0.3%, enhanced oil recoveries in both sandpacks increase greatly. The improvement in enhanced oil recovery in the low-permeability sandpack is the main contribution to enhancement of total oil recovery.
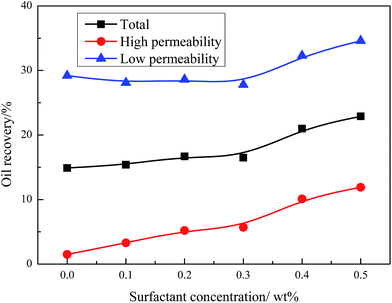 |
| Fig. 8 Enhanced oil recovery variations of 1250 mg L−1 B-PPG/1250 mg L−1 HPAM and surfactant mixed solutions with surfactant concentration. | |
In order to further prove the efficiency of surfactant on oil recovery enhancement, the core flood tests of B-PPG and HPAM mixed solutions with the same cost as B-PPG/HPAM/surfactant mixed solutions are carried out. According to this principle, 0.4% surfactant can be changed to 3000 mg L−1 HPAM or 2000 mg L−1 B-PPG. Fig. 9 shows fractional flows in the high- and low-permeability sandpacks for different B-PPG/HPAM/surfactant mixed solutions. The 1250 mg L−1 B-PPG and 4250 mg L−1 HPAM mixed solution shows a more obvious adjustment in fractional flow than the B-PPG/HPAM/surfactant one. Especially, during subsequent water flooding period, fractional flow in the low-permeability sandpack is much higher than that in the high-permeability sandpack. The 3250 mg L−1 B-PPG and 1250 mg L−1 HPAM mixed solution also shows a more obvious adjustment in fractional flow than the B-PPG/HPAM/surfactant one. At the final stage of subsequent water flooding, stable fractional flow in the low-permeability sandpack is higher than that in the high-permeability sandpack. Table 3 shows oil recoveries for these tests. The B-PPG/HPAM/surfactant mixed solution has a higher total oil recovery and a much higher oil recovery in the high-permeability sandpack than other mixed solutions. This means the replacement of surfactant by HPAM or B-PPG can enhance the ability of adjusting fractional flow, but cannot enhance oil recovery, especially oil recovery in the high-permeability sandpack. The presence of surfactant in B-PPG/HPAM mixed solutions has a more important effect on oil recovery enhancement than increase in B-PPG or HPAM concentration.
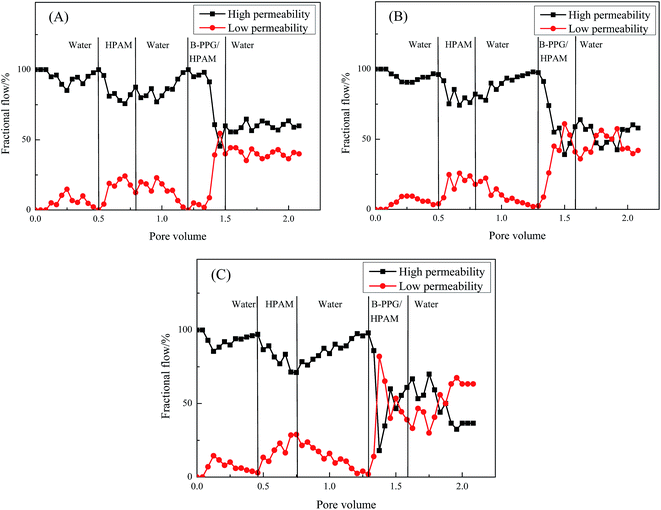 |
| Fig. 9 Fractional flows in high- and low-permeability sandpacks for different B-PPG/HPAM/surfactant mixed solutions. (A) 1250 mg L−1 B-PPG, 1250 mg L−1 HPAM, and 0.40% surfactant; (B) 1250 mg L−1 B-PPG and 4250 mg L−1 HPAM; (C) 3250 mg L−1 B-PPG and 1250 mg L−1 HPAM. | |
Table 3 Summary of core flood tests with different B-PPG/HPAM/surfactant mixed solutions
Flooding solutions |
Sandpacks |
Oil recovery after water flooding/% |
Oil recovery after HPAM flooding/% |
Oil recovery enhanced by HPAM flooding/% |
Oil recovery after flooding of mixed solution/% |
Oil recovery enhanced by flooding of mixed solution/% |
1250 mg L−1 B-PPG, 1250 mg L−1 HPAM, and 0.40% surfactant |
High permeability |
51.1 |
74.7 |
23.6 |
84.8 |
10.1 |
Low permeability |
10.0 |
45.1 |
35.1 |
77.4 |
32.3 |
Total |
31.0 |
60.2 |
29.2 |
81.2 |
21.0 |
1250 mg L−1 B-PPG and 4250 mg L−1 HPAM |
High permeability |
53.4 |
75.3 |
21.9 |
78.1 |
2.8 |
Low permeability |
16.3 |
41.3 |
25.1 |
72.0 |
30.7 |
Total |
35.4 |
58.8 |
23.4 |
75.1 |
16.3 |
3250 mg L−1 B-PPG and 1250 mg L−1 HPAM |
High permeability |
53.5 |
72.5 |
19.0 |
74.0 |
1.5 |
Low permeability |
14.6 |
43.2 |
28.6 |
72.8 |
29.6 |
Total |
34.7 |
58.3 |
23.7 |
73.4 |
15.1 |
3.4 Fractional flow and oil recovery of B-PPG, HPAM and surfactant mixed solutions in different heterogeneous sandpacks
B-PPG/HPAM/surfactant mixed solutions have a good ability to adjust fractional flow and enhance oil recovery in the parallel sandpacks with a permeability ratio of 1
:
3. The core flood tests with different heterogeneous sandpacks are carried out to investigate the effect of B-PPG/HPAM/surfactant mixed solutions on the adjustment of fractional flow and enhancement of oil recovery. Fig. 10 shows fractional flow of B-PPG/HPAM/surfactant mixed solutions in the high- and low-permeability sandpacks with different heterogenous media. When the permeability ratios are 1
:
6 and 1
:
9, although the abilities of B-PPG/HPAM/surfactant mixed solutions to adjust fractional flow become weak, the mixed solutions have stronger abilities than the former HPAM flooding. Table 4 shows oil recoveries of core flood tests of B-PPG/HPAM/surfactant mixed solutions in different heterogeneous sandpacks. It is clear that oil recovery after polymer flooding and that after B-PPG/HPAM/surfactant mixed flooding both decrease with increase in heterogeneity. However, total oil recovery enhancement by B-PPG/HPAM/surfactant mixed solutions show no great decrease. These results can prove that B-PPG/HPAM/surfactant mixed solutions have strong abilities to adjust fractional flow in both the high- and low-permeability sandpacks and enhance the oil recovery, even when the permeability ratio becomes 1
:
9.
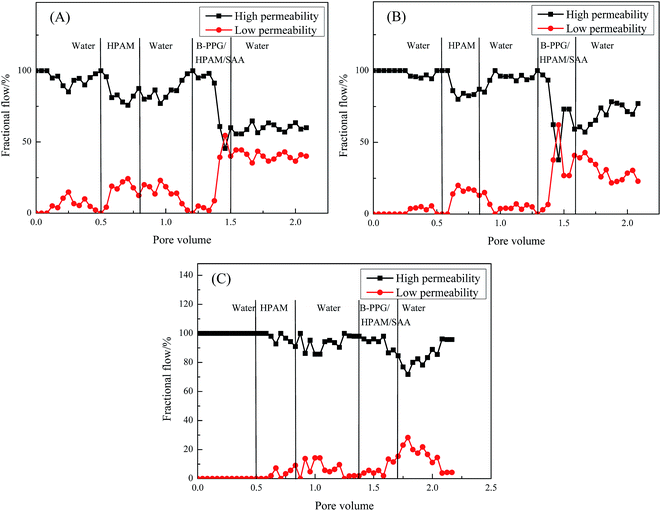 |
| Fig. 10 Fractional flows of the 1250 mg L−1 B-PPG/1250 mg L−1 HPAM/0.40% surfactant mixed solutions in the heterogenous high- and low-permeability sandpacks. The permeability ratios of (A) to (C) are 1 : 3, 1 : 6, and 1 : 9, respectively. | |
Table 4 Summary of core flood tests of B-PPG, HPAM and surfactant mixed solutions in different heterogeneous sandpacks
Permeability ratio |
Sandpacks |
Oil recovery after water flooding/% |
Oil recovery after HPAM flooding/% |
Oil recovery enhanced by HPAM flooding/% |
Oil recovery after B-PPG/HPAM/surfactant flooding/% |
Oil recovery enhanced by B-PPG/HPAM/surfactant flooding/% |
1 : 3 |
High permeability |
51.1 |
74.7 |
23.6 |
84.8 |
10.1 |
Low permeability |
10.0 |
45.1 |
35.1 |
77.4 |
32.3 |
Total |
31.0 |
60.2 |
29.2 |
81.2 |
21.0 |
1 : 6 |
High permeability |
55.2 |
73.3 |
18.1 |
83.5 |
10.2 |
Low permeability |
5.2 |
28.4 |
23.2 |
65.1 |
36.7 |
Total |
30.7 |
53.2 |
22.5 |
76.0 |
22.8 |
1 : 9 |
High permeability |
56.5 |
77.4 |
20.9 |
86.3 |
8.9 |
Low permeability |
0.0 |
15.1 |
15.1 |
48.7 |
33.6 |
Total |
29.2 |
47.3 |
18.1 |
68.1 |
20.8 |
4. Conclusions
Laboratory experiments have been carried out to investigate fractional flow and oil recovery enhancement of B-PPG and HPAM mixed solutions after HPAM flooding in parallel-sandpack models. B-PPG and HPAM have a synergistic effect to adjust fractional flow and enhance oil recovery, especially oil recovery in the low permeability sandpack. The composition and concentration of B-PPG and HPAM significantly affect the ability to adjust fractional flow and enhance oil recovery. When the mass percentage of B-PPG is 50% and total concentration is 2500 mg L−1, the mixed solution has the best ability to adjust fractional flow and enhance oil recovery. Furthermore, the presence of a surfactant in mixed solution can further enhance total oil recovery and oil recovery in the high-permeability sandpack. B-PPG/HPAM/surfactant mixed solutions have strong abilities to adjust fractional flow and enhance oil recovery, even when the permeability ratio of the parallel sandpacks becomes 1
:
9. B-PPG/HPAM/surfactant mixed solutions can further improve both sweep efficiency and displacement efficiency to enhance oil recovery after polymer flooding. Therefore, B-PPG/HPAM/surfactant mixed solutions are suitable to further enhance oil recovery oilfields, especially for reservoirs with high heterogeneity after polymer flooding.
Acknowledgements
We gratefully acknowledge financial support from the Natural Science Foundation of China (Grant No. 51204197), the Fundamental Research Funds for the Central Universities (17CX05005) and Program for Changjiang Scholars and Innovative Research Team in University (IRT1294).
References
- H. L. Chang, Polymer flooding technology yesterday today and tomorrow, J. Pet. Technol., 1978, 30, 1113–1128 CrossRef CAS.
- R. Jewett and G. Schurz, Polymer flooding-a current appraisal, J. Pet. Technol., 1970, 22, 675–684 CrossRef.
- H. Chang, Z. Zhang, Q. Wang, Z. Xu, Z. Guo, H. Sun, X. Cao and Q. Qiao, Advances in polymer flooding and alkaline/surfactant/polymer processes as developed and applied in the People's Republic of China, J. Pet. Technol., 2006, 58, 84–89 CrossRef.
- G. Li, L. Zhai, G. Xu, Q. Shen, H. Mao and M. Pei, Current tertiary oil recovery in China, J. Dispersion Sci. Technol., 2000, 21, 367–408 CrossRef CAS.
- H. Saboorian-Jooybari, M. Dejam and Z. Chen, Heavy oil polymer flooding from laboratory core floods to pilot tests and field applications: Half-century studies, J. Pet. Sci. Eng., 2016, 142, 85–100 CrossRef CAS.
- J. Wang and M. Dong, Optimum effective viscosity of polymer solution for improving heavy oil recovery, J. Pet. Sci. Eng., 2009, 67, 155–158 CrossRef CAS.
- E. Delamaide, A. Zaitoun, G. Renard and R. Tabary, Pelican Lake field: first successful application of polymer flooding in a heavy-oil reservoir, SPE Reservoir Eval. Eng., 2014, 17, 340–354 CrossRef.
- Z. Zhang, J. Li and J. Zhou, Microscopic Roles of “Viscoelasticity” in HPMA polymer flooding for EOR, Transp. Porous Media, 2011, 86, 199–214 CrossRef CAS.
- S. A. Shedid, Influences of fracture orientation on oil recovery by water and polymer flooding processes: An experimental approach, J. Pet. Sci. Eng., 2006, 50, 285–292 CrossRef CAS.
- J. Hou, Z. Li, S. Zhang, X. Cao, Q. Du and X. Song, Computerized tomography study of the microscopic flow mechanism of polymer flooding, Transp. Porous Media, 2009, 79, 407–418 CrossRef CAS.
- A. Afsharpoor, K. Ma, A. Duboin, K. Mateen, S. Jouenne and P. Cordelier, Micro-Scale Experiment and CFD Modeling of Viscoelastic Polymer; Trapped Oil Displacement and Deformation at the Dead-End, in SPE Improved Oil Recovery Symposium, Society of Petroleum Engineers, 2014 Search PubMed.
- D. Wang, R. S. Seright, Z. Shao and J. Wang, Key aspects of project design for polymer flooding, in SPE Annual Technical Conference and Exhibition, Society of Petroleum Engineers, 2007, pp. 1117–1124 Search PubMed.
- A. D. Gupta, G. A. Pope, K. Sepehrnoori and M. Shook, Effects of Reservoir Heterogeneity on Chemically Enhanced Oil Recovery, SPE-14889-PA, 1988, vol. 3, pp. 479–488 Search PubMed.
- H. Emami Meybodi, R. Kharrat and M. H. Ghazanfari, Effect of Heterogeneity of Layered Reservoirs on Polymer Flooding: An Experimental Approach Using 5-Spot Glass Micromodel, in SPE-113820-MS, Society of Petroleum Engineers, 2008, pp. 1–9 Search PubMed.
- F.-L. Zhao, Y.-F. Wang, C.-L. Dai, S. Ren and C. Jiao, Techniques of enhanced oil recovery after polymer flooding, J. China Univ. Pet., Ed. Nat. Sci., 2006, 30, 86–89 Search PubMed.
- D. Guo, H. Xin, X. Cui and H. Xie, Study on enhanced oil recovery by using OCS surfactant/polymer binary system after polymer flooding, Adv. Fine Petrochem., 2006, 7, 1–3 Search PubMed.
- H. Gong, G. Xu, Y. Zhu, Y. Wang, D. Wu, M. Niu, L. Wang, H. Guo and H. Wang, Influencing factors on the properties of complex systems consisting of hydrolyzed polyacrylamide/triton X-100/cetyl trimethylammonium bromide: viscosity and dynamic interfacial tension studies, Energy Fuels, 2008, 23, 300–305 CrossRef.
- X. Lu and Y. Zhang, EOR methods after polymer flooding and their action mechanisms, Pet. Geol. Oilfield Dev. Daqing, 2007, 26, 113–118 CAS.
- J. Vargo, J. Turner, V. Bob, M. J. Pitts, K. Wyatt, H. Surkalo and D. Patterson, Alkaline-surfactant-polymer flooding of the Cambridge Minnelusa field, SPE Reservoir Eval. Eng., 2000, 3, 552–558 CrossRef CAS.
- H. Liu, J. Wang, L. Yang, D. Jiang, C. Lv, C. Cui and S. Gao, Studies on a foam system of ultralow interfacial tension applied in Daqing oilfield after polymer flooding, J. Chem., 2013, 2013, 105274 Search PubMed.
- Q. Wang, X. Song, G. Zhou, P. Guo, X. Li, H. Li and X. Li, Experiment of enhancing oil recovery by foam flooding post polymer displacement, J. Jianghan Pet. Inst., 2004, 26, 105–107 CAS.
- Q. Hou, Y. Zhu, Y. Luo and R. Weng, Studies on foam flooding EOR technique for daqing reservoirs after polymer flooding, in SPE Improved Oil Recovery Symposium, Society of Petroleum Engineers, 2012 Search PubMed.
- R. Sydansk, Y. Xiong, A. Al-Dhafeeri, R. Schrader and R. Seright, Characterization of partially formed polymer gels for application to fractured production wells for water-shutoff purposes, in SPE/DOE Symposium on Improved Oil Recovery, Society of Petroleum Engineers, 2004 Search PubMed.
- B. Bai, Y. Li and X. Liu, New development of water shutoff and profile control in oilfields in China, Oil Drill. Prod. Technol., 1999, 20, 64–68 Search PubMed.
- Y. Wu, T. Tang, B. Bai, X. Tang, J. Wang and Y. Liu, An experimental study of interaction between surfactant and particle hydrogels, Polymer, 2011, 52, 452–460 CrossRef CAS.
- F. B. Thomas, D. B. Bennion, G. Anderson, B. Meldrum and W. Heaven, Water shut-off treatments-reduce water and accelerate oil production, J. Can. Pet. Technol., 2000, 39, 25–29 Search PubMed.
- R. Sydansk and G. Southwell, More than 12 years of experience with a successful conformance-control polymer gel technology, in SPE/AAPG Western Regional Meeting, Society of Petroleum Engineers, 2000 Search PubMed.
- R. Qiao and W. Zhu, Evaluation of modified cationic starch for impeding polymer channeling and in-depth profile control after polymer flooding, J. Ind. Eng. Chem., 2010, 16, 278–282 CrossRef CAS.
- Q. Feng, X. Chen and M. Sun, Study of the multiple-profile control system to enhance oil recovery after polymer flooding, J. Pet. Explor. Prod. Technol., 2012, 2, 133–139 CrossRef CAS.
- X. Cui, Z. Li, X. Cao, X. Song, X. Chen and X. Zhang, A novel PPG enhanced surfactant-polymer system for EOR, in SPE Enhanced Oil Recovery Conference, Society of Petroleum Engineers, 2011 Search PubMed.
- C. Xiaoyan, Method study for application of heterogeneous oil displacement agent, Oil Drill. Prod. Technol., 2009, 5, 027 Search PubMed.
- Q. Sang, Y. Li, L. Yu, Z. Li and M. Dong, Enhanced oil recovery by branched-preformed particle gel injection in parallel-sandpack models, Fuel, 2014, 136, 295–306 CrossRef CAS.
- T. S. Urbissinova and E. Kuru, Effect of elasticity during viscoelastic polymer flooding: a possible mechanism of increasing the sweep efficiency, J. Can. Pet. Technol., 2010, 49, 49–56 CrossRef CAS.
- R. Ponnapati, O. Karazincir, E. Dao, R. Ng, K. Mohanty and R. Krishnamoorti, Polymer-functionalized nanoparticles for improving waterflood sweep efficiency: Characterization and transport properties, Ind. Eng. Chem. Res., 2011, 50, 13030–13036 CrossRef CAS.
|
This journal is © The Royal Society of Chemistry 2017 |