DOI:
10.1039/C7RA02830J
(Paper)
RSC Adv., 2017,
7, 19794-19807
Retracted Article: He-Wei granules (HWKL) combat cisplatin-induced nephrotoxicity and myelosuppression in rats by inhibiting oxidative stress, inflammatory cytokines and apoptosis†
Received
8th March 2017
, Accepted 24th March 2017
First published on 4th April 2017
Abstract
As a highly effective antineoplastic chemotherapeutic drug, cisplatin is widely used clinically to treat a variety of human malignancies. However, its clinical use is severely limited by serious side-effects, of which nephrotoxicity and myelosuppression are considered to be the most important because they significantly reduce patient life quality and hinder effective use. In this study, we investigated whether HWKL, a product obtained from modified Ban-xia xie-xin decoction first described 1700 years ago, exhibits protective effects against cisplatin-induced nephrotoxicity and myelosuppression. After intraperitoneal injection of 7 mg kg−1 cisplatin to Wistar rats, nephrotoxicity and myelosuppression were observed. HWKL reduced cisplatin-induced elevations in blood urea nitrogen (BUN) and serum creatinine (Scr) levels, increased water intake and improved renal tubular lesions. Cisplatin-induced increases in tumor necrosis alpha (TNF-α) protein, interleukin-1 beta (IL-1β) protein, cyclooxygenase-2 (COX-2) protein, extracellular signal-regulated kinase (ERK), TNF-α mRNA and IL-1β mRNA were significantly reduced by HWKL and cisplatin-induced oxidative stress was also inhibited by HWKL. Furthermore, HWKL contributed to increase immunological function to combat cisplatin-induced myelosuppression. Our findings indicate that HWKL inhibits cisplatin-induced nephrotoxicity and myelosuppression via several mechanisms which operate simultaneously and provide basic evidence to confirm that HWKL could be useful in clinical situations as a protective agent to prevent cisplatin-induced nephrotoxicity and myelosuppression.
Introduction
Cisplatin was accidentally discovered to inhibit cell division in 1965 by American biophysicist and chemist Barnett Rosenberg.1 Although many years have passed since then, cisplatin remains one of the most widely used drugs to treat a variety of human cancers in clinical situations although there are now many platinum analogues available because of their potent anticancer effects and low cost.1–4 However, cisplatin can produce severe toxicity during chemotherapy despite its marked antineoplastic properties, including nausea, emesis, nephrotoxicity, myelosuppression and ototoxicity.3 As a dose-dependent antineoplastic drug, the therapeutic efficacy of cisplatin is improved by increasing the dose although this can lead to more severe toxicity.5 More than 70% of pediatric patients receiving cisplatin experience renal dysfunction and due to severe toxicity, the use of cisplatin is restricted. Although there are some therapeutic methods which are used to prevent cisplatin-induced nephrotoxicity and myelosuppression, no recommended specific treatment is currently available.6 Therefore, more effective therapies or combination therapies must soon be developed to treat cancer.7–9
Currently, renal cell apoptosis, inflammation, necrosis and oxidative stress have been shown to contribute to cisplatin-induced nephrotoxicity while oxidative stress still plays an important role in cisplatin-induced myelosuppression.10–13 At present, intensive hydration has been proposed as a possible method for preventing cisplatin-induced nephrotoxicity although the clinic outcome using this method has been unsatisfactory. It has been reported that hydration therapy does not resolve kidney dysfunction in a significant percentage of treated patients11 and, furthermore, there are few effective methods to reduce cisplatin-induced myelosuppression. As a consequence of these unsatisfactory outcomes, discontinuation of cancer treatment using cisplatin therapy remains the only option for patients due to the possibility of severe nephrotoxicity and myelosuppression. Therefore, identifying effective methods for preventing cisplatin-induced renal damage and myelosuppression is still a critical issue in cancer therapeutics.
BXXXT was first described in “Shang-Han-Lun” about 1700 years ago for the treatment of many different gastrointestinal symptoms and treating patients with a weak spleen and stomach, internal stasis of heat and cold glomus and fullness below the heart without pain, vomiting and borborygmus, and so on.14–18 However, involving in modern pharmacological applications, it is always used to treat peptic ulcers, gastrointestinal dysfunction, chemotherapeutic toxicity, and others.14–18 Because of people's corporeity and germplasm of medicinal materials over the years, traditional prescriptions could not always remain the permanent clinical effect compared to the old days. Hence, it is necessary to keep pace with the times.16 As same as BXXXT, HWKL (patent number: ZL201110235915.7) consists of seven herbs namely: Pinellia ternate (Thunb.) Breit. (Pinelliae Rhizoma Praeparatum, Araceae, PR), Zingiber officinale Rosc. (Zingiberis Rhizoma Recens, Zingiberaceae, ZB), Panax ginseng C. A. Mey. (Ginseng Radix Et Rhizoma, Araliaceae, GR), Scutellaria baicalensis Georgi. (Scutellariae Radix, Labiatae, SR), Coptis chinensis Franch. (Coptidis Rhizoma, Ranunculaceae, CR), Glycyrrhiza uralensis Fisch. (Glycyrrhizae Radix Et Rhizoma Praeparata Cum Melle, Leguminosae, GHR) and Ziziphus jujuba Mill. (Jujubae Fructus, Rhamnaceae, JF), which are currently being investigated in clinical and laboratory studies. HWKL contains a little more of PR than BXXXT and Zingiber officinale Rosc. (Zingiberis Rhizoma, Zingiberaceae, ZR) is replaced with ZB. HWKL is a kind of modern product derived from the modified formulation of BXXXT using a number of techniques which is currently used in Dalian Orthopedic Hospital and Hospital 463 of People's Liberation Army as a replacement of BXXXT. The recovery rate of gastrointestinal injury could reach 97.2% and the degree of satisfaction of reducing chemotherapy-induced toxicity could reach 71.4% according to the statistics in hospitals. According to previous studies, we found that the principal bioactive compounds of BXXXT were ginsenosides, alkaloids, flavonoids, gingerols and polysaccharides.19 In order to guarantee the quality of HWKL, an efficient and reliable analytical method based on UHPLC-Q-TOF-MS/MS and chemical profiling was developed.
Although a lot of research has been carried out on BXXXT, there have been few studies into the properties and possible mechanism of action of BXXXT.17,18,20 Furthermore, there have been no studies of the pharmacological effects of HWKL on cisplatin-induced nephrotoxicity and myelosuppression. Our present work described here aimed to investigate the protective effects and possible mechanisms of action of HWKL on cisplatin-induced nephrotoxicity and myelosuppression.
Materials and methods
Reagents and chemicals
Cisplatin for injection and ondansetron hydrochloride tablets from Qilu Pharmaceutical Co., Ltd (Shandong, China) were prepared in sterile saline to proper concentration. Domperidone tablets from Xian Janssen Pharmaceutical Ltd (Shanxi, China) was well-distributed in pure water. TNF-α, COX-2, pERK1/2 and IL-1β enzyme linked immunosorbent assay (ELISA) kits were purchased from Shanghai Yuan-Mu bio-medical company (Shanghai, China). Malonaldehyde (MDA), superoxide dismutase (SOD) and glutathione peroxidase (GSH-PX) kit were obtained from Nanjing Jiancheng Bioengineering Institute (Nanjing, China). Ultra-high performance liquid chromatography (UHPLC)-MS grade acetonitrile was purchased from Honeywell Company Inc. (Morris, New Jersey, USA). Formic acid was obtained from ROE Scientific Inc. (USA). Ultra-pure water was prepared using a Milli-Q SP system (Millipore, Bedford, MA, USA). All other reagents were of highest purity and commercially available by Shenyang Lab Science and Trade Co., Ltd (Shenyang, China).
Composition and preparation of samples
All the crude herbal drugs were purchased from Shenyang Guo-Da Pharmacy (Shenyang, Liaoning) and were confirmed as genuine medicinal materials by Professor Jun Yin of Pharmacognostical department. All the herbarium was stored in school of traditional medicine.
BXXXT
Six crude herbal drugs (PR 12 g, ZR 9 g, GR 9 g, SR 9 g, GHR 9 g and JF 6 g) were immersed in purified water for 2 h except the herb SR and decocted with 14-fold amount of purified water for 2 h, 2×. CR (3 g) was immersed in purified water for 2 h and decocted with 12-fold purified water for 2 h, 3×. After filtration, two decoctions were mixed and evaporated, and then spray dried with insoluble dextran into a granulated powder, packaged and stored in foil containers at 4 °C. The BXXXT granule was dissolved in pure water to a proper concentration when used.
HWKL
Six crude herbal drugs (PR, ZB, GR, SR, GHR and JF, ratio 6
:
4
:
3
:
3
:
3
:
5) were immersed in purified water for 2 h except the herb SR and decocted with 14-fold amount of purified water for 2 h, 2×. CR (3 g) was immersed in purified water for 2 h and decocted with 12-fold purified water for 2 h, 3×. After filtration, two decoctions were mixed and evaporated, and then spray dried with insoluble dextran into a granulated powder, packaged and stored in foil containers at 4 °C. The HWKL granule was dissolved in pure water to a proper concentration when used.
Sample for UHPLC-Q-TOF-MS/MS
The HWKL powder (50 mg) was subjected to proper amount of 80 °C water and then vortexed for one minute, placed in a boiling water bath for 30 additional minutes with one minute vortexing for every ten minute. The sample was then cooled and centrifuged and the resulting supernatant was filter sterilized (0.22 μm) and subjected to LC-MS analysis (1.0 μL each time).
UHPLC-Q-TOF-MS/MS analysis
The analysis was performed on an Agilent series 1290 UHPLC instrument (Agilent Technologies, Santa Clara, CA, USA) equipped with an automatic degasser, a quaternary pump, and an auto-sampler. The oven temperature was maintained at 40 °C. The temperature of auto-sampler was fixed at 4 °C. Chromatographic separations were achieved on an Agilent Extend C18 column (1.8 μm, 50 mm × 2.1 mm). Flow rate was 0.3 mL min−1 and the sample injection volume was 1.0 μL. The gradient profile was optimized as follows: the mobile phase consisted of (A) 0.2% formic acid in water and (B) acetonitrile with the following gradient elution: 0–6 min, 10–60% B; 6–9 min, 60–95% B; 9–11 min, 95% B; 11–11.1 min, 95–10% B; 11.1–18 min, 10% B. The mass spectrometric analysis was performed with a UHPLC-Q-TOF-MS/MS (Agilent Technologies, Santa Clara, CA, USA) equipped with an ESI source with Jet Stream technology using the following parameters: drying gas (N2) flow rate, 7.0 L min−1; drying gas temperature, 300 °C; nebulizer, 35 psig, sheath gas temperature 300 °C. The mass spectrometer was operated both in positive and negative ion mode and mass spectra were scanned in the range of m/z 50–1500 in both modes. A reference mass solution containing reference ions at m/z 112.9856 and 1033.9881 was used as the lock mass for accurate mass measurement in the negative mode while the reference mass solution containing reference ions at 121.0508 and 922.0098 was used in the positive mode. The data were analyzed by Agilent Mass Hunter Workstation Version B.03.01 Software.
Animals and treatment
54 nine-week-old male Wistar rats weighing 250–280 g were purchased from Liaoning Changsheng biotechnology company (Benxi, China). Rats were maintained in standard polypropylene transparent cages covered with steel grids under environmentally controlled conditions (23 ± 2 °C relative humidity 50 ± 10%, 12 h dark/light cycles, food and water ad libitum). All of the experimental protocols were approved by the Animal Care and Use Committee of Shenyang Pharmaceutical University (SYPU-IACUC-C2016-0316-207). All experiments were performed in accordance with published National Institutes of Health guidelines. Experiments commenced at least 7 days after the arrival of animals to the laboratory.
After 7 days acclimatization period, rats were randomly divided into the following 9 groups (n = 6) namely: blank group, cisplatin group, cisplatin + ondansetron group, cisplatin + domperidone group, cisplatin + BXXXT group (1.38 g kg−1), cisplatin + HWKL low dose group (1.18 g kg−1), cisplatin + HWKL middle dose group (2.36 g kg−1, equal to clinical dose of human beings), cisplatin + HWKL high dose group (4.725 g kg−1), HWKL group (2.36 g kg−1). In experimental observation period, rats in the blank and cisplatin groups were treated with pure water according to body weight every day, rats in simple HWKL group were treated with HWKL 2.36 g kg−1, i.g. every day, rats in cisplatin + ondansetron group were treated with ondansetron 1.3 mg kg−1 (equal to clinical dose of human beings), i.g. every day, rats in cisplatin + domperidone group were treated with domperidone 3 mg kg−1 (equal to clinical dose of human beings), i.g. every day, rats in cisplatin + BXXXT group were treated with BXXXT 1.38 g kg−1 (equal to clinical dose), i.g. every day, rats in cisplatin + HWKL low dose, cisplatin + HWKL middle dose and cisplatin + HWKL high dose groups were, respectively, treated with HWKL 1.18, 2.36 and 4.725 g kg−1 (equal to 0.5-fold, 1-fold and 2-fold clinical doses), respectively. The samples involved in the experiment were prepared in different concentrations to make sure the same administration volume. Cisplatin (7 mg kg−1, i.p.) was administrated 1.5 h after each treatment with the antiemetic agent or its vehicle except the blank and HWKL groups. Following the administration of cisplatin, body weight, food intake and water intake were observed in every 24 h continuously 168 h.
Hematology and histopathology
The blood was drawn from the retro-orbital sinus 72 h and 144 h after cisplatin injection. The blood was divided into two parts, one was used to observe the hematological factors, including white blood cell (WBC), red blood cell (RBC), platelet (PLT), by Nihon Kohden MZK-6318 blood analyzer (Japan). Another part was centrifuged at 3500 rpm min−1 for 15 min at 4 °C to obtain the serum. Serum blood urea nitrogen (BUN) and creatinine (CREA) were measured by erba XL600 automatic biochemical analyser (Germany). Related reagents were purchased from Shanghai Fosun Long March Medical Science Co., Ltd.
On the last experimental day (168 h), kidney was dissected, rinsed in ice-cold normal saline, and fixed in 10% formalin for at least 24 h. After fixation, all tissues were processed, Paraplast-fixed blocks sectioned (5 μm thickness), and slides stained with haematoxylin and eosin. Representative microphotographs were obtained with a light microscope (Nikon, Japan).
ELISA, MDA, GSH-PX and SOD assays
Serum. The blood was drawn from the retro-orbital sinus 72 h and 144 h after cisplatin injection and then centrifuged at 3500 rpm min−1 for 15 min at 4 °C to obtain the serum.
Tissue homogenate. Tissue sample was immersed in 9-fold volume of PBS/0.9% saline and ground into a powder in ice by Scientz-IID ultrasonic cell disruption system (Ningbo Scientz Biotechnology Co., Ltd, Ningbo, China). The extracts were centrifuged at 3500 rpm min−1 for 15 min at 4 °C to remove insoluble material.The serum and supernatant fractions were analyzed using ELISA kits, MDA, GSH-PX and SOD kits according to the manufacturer's instructions. After the experiment, the absorbance of each sample was measured at 450 nm (ELISA), 532 nm (MDA), 412 nm (GSH-PX) and 550 nm (SOD) respectively with a microplate reader (Thermo Scientific L-117, USA).
Standard curves were prepared using diluted standard solutions to allow calculation of the relative target in the samples. All standards and samples were run in duplicate.
Quantitative real time PCR. Total RNA was isolated from kidney and detected by quantitative real time PCR. Rat Gapdh was used as endogenous control for sample normalization. Results are shown as fold-increases relative to the expression of rat Gapdh. The PCR primer pairs were as follows: rat Gapdh, 5′-GCAAGTTCAACGGCACAGTCA-3′ (forward) and 5′-TGGTGGTGAAGACGCCAGTAG-3′ (reverse); rat TNF-α, 5′-GGGCTCCCTC TCATCAGTTC-3′ (forward) and 5′-GCTTGGTGG TTTGCTACGA C-3′ (reverse); rat IL-1β, 5′-TGAAGCAGCTATGGCAACTG-3′ (forward) and 5′-CTGCCTTCCTG A AGCTCTTG-3′ (reverse). The contents was measured by fold to control.
Immunoblotting assay. The kidney was lysed in pre-cold RIPA lysis buffer and centrifuged at 14
000 rpm for 15 min at 4 °C. The total protein contained in the supernatants was analyzed by relative amount SDS-PAGE and transferred onto NC membranes. After blocking, the membranes were incubated overnight with relative primary antibodies. Next, the membranes were incubated with secondary antibodies and detected with enhanced chemiluminescence detection kit (Nanjing Kengen Biotech Co., Ltd, Nanjing, Jiangsu, China). The intensity of each band was determined by using Gel-Pro32 software (Media Cybernetics, Bethesda, MD, USA).Pro caspase-3, cleaved caspase-3 and p53 primary antibodies were purchased from immunoWay Biotechnology Company (Newark, DE, USA).
Organ indexes and marrow DNA detection
Organ indexes. Rats were sacrificed 168 h after administration of cisplatin. Tissues, including kidney, spleen and thymus, were removed from every animal for calculating organ index to evaluate the conditions of those organs. The calculating formula was as follows.
Organ index = the weight of organ/the body weight × 100% |
Marrow DNA detection. An intact right femur was washed with 20 mL 0.005 mol L−1 CaCl2 solution. Then, the solution was placed at 4 °C in refrigerator for 30 min and centrifuged at 2500 rpm min−1 for 15 min. The supernatant was discarded and the residue was mixed with 10 mL 0.2 mol L−1 HClO4 solution and vortexed 1 min and placed in a boiling water bath for 15 min. The solution was cooled and filtered. The filtrate was measured at 260 nm by ultraviolet spectrophotometer (UV-1200, AOE instrument, Shanghai, China).
Statistical analysis. All values are presented as mean ± SEM. One-way ANOVA analysis is used to compare the values collected in the same time points meanwhile Two-way ANOVA analysis is used to compare the values collected in different time points. Differences were considered statistically significance at p < 0.05.
Results
Chemical analysis of HWKL by UHPLC-Q-TOF-MS/MS
In order to identify the main components of HWKL in a thorough and efficient manner by measuring the ingredients of each herb, the method used in an earlier study21 was used. By searching many recommended databases such as PubMed of the U.S. National Library Medicine and the National Institutes of Health, SciFinder Scholar of the American Chemical Society and Chinese National Knowledge Infrastructure (CNKI) of Tsinghua University, all the compounds reported in the literature involving PR, ZR, ZB, GR, SR, GHR, JF and CR were summarized in a Microsoft Office Excel table to establish an in-house library, containing the name, molecular formula, chemical structure and literatures of each compounds. The table was used to find and match the empirical molecular formulae with those of the published known compounds in the library. The empirical molecular formula of each was short listed by comparing the accurately measured mass value with the exact mass value of putative molecules at a mass accuracy less than 5 ppm.
A total of 37 major components were identified using UHPLC-Q-TOF-MS/MS, of which 11 were confirmed and quantified by using reference compounds.19,22,23 A typical base peak chromatogram (BPC) of an HWKL sample is presented in Fig. 1. The properties and structures of all identified compounds are presented in ESI Table S1 and Fig. S1† respectively. An external standard method for the 11 major compounds was established to control the quality of HWKL by using HPLC in terms of linearity, precision, accuracy, and stability.
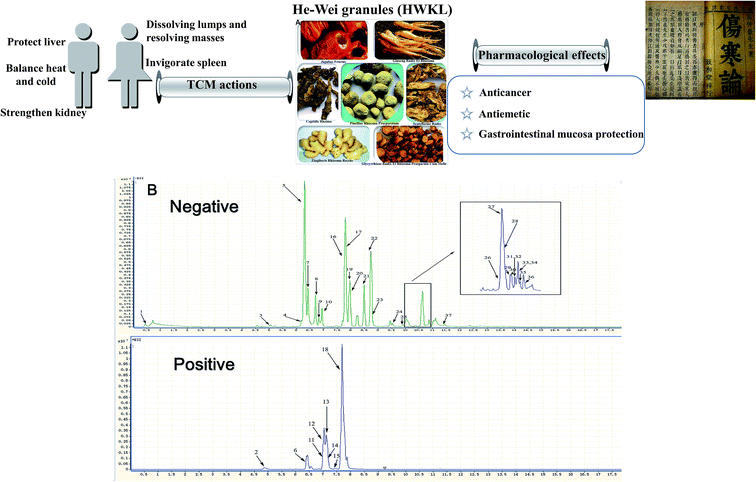 |
| Fig. 1 Herbs involved in HWKL and traditional Chinese medicinal uses and pharmacological effects in clinical situations (A). Representative base peak chromatogram of HWKL analyzed by UHPLC-Q-TOF-MS/MS (B). (1*: sucrose, 2: magnoflorine, 3: liquiritigenin 7-O-glucoside-4′-O-apiosyl-O-glucoside, 4*: liquiritin, 5*: isoliquiritin, 6: berberrubine, 7*: scutellarin, 8: isomer of chrysin-6-C-arabinoside-8-C-glucoside, 9: chrysin-6-C-arabinoside-8-C-glucoside, 10: acteoside, 11: coptisine, 12: epiberberine, 13: columbamine, 14: jatrorrhizine, 15: palmatine, 16*: baicalin, 17: isomer of baicalin, 18*: berberine, 19*: ginsenoside Rg1, 20*: ginsenoside Re, 21: wogonoside, 22: oroxylin A 7-O-glucuronide, 23: 22-β-acetoxyl licorice saponin G2, 24: 22-β-acetoxylglycyrrhizic acid, 25: 20S-ginsenoside Rg2/20R-ginsenoside Rg2, 26*: ginsenoside Rb1, 27: pinellic acid, 28: ginsenoside Rc, 29: ginsenoside Rb2, 30: ginsenoside Rb3, 31: licorice saponin G2, 32: licorice saponin H2, 33: licorice saponin J2, 34: licorice saponin K2, 35: ginsenoside Rd, 36*: wogonin, 37*: 6-gingerol. * stands for being confirmed and quantified by relative reference compounds.) | |
HWKL combats cisplatin-induced renal injury
After cisplatin administration, food and water consumptions and body weight were observed to be sharply reduced in the cisplatin group (ESI Tables S2, S3 and S4†). After cisplatin injection, anorexia and body weight loss were observed because of the severely reduced food intake in the cisplatin group in comparison with the blank group. Renal damage induced by cisplatin administration was usually accompanied by a reduction in water consumption while the biomarker BUN and Scr levels increased. The water consumption of the cisplatin group was greatly reduced in all groups after cisplatin administration which suggests that nephrotoxicity was induced by cisplatin (ESI Tables S2, S3 and S4†). The kidney index, BUN and Scr levels of the cisplatin group were observed to increase significantly at 72 h and 144 h after cisplatin administration which confirmed the kidney injury developed after cisplatin injection (Fig. 2A and B). Chemotherapy-induced renal damage is usually accompanied by hematological changes. Red blood cell (RBC), red blood cell specific volume (HCT) and hemoglobin (HGB) levels were all found to be sharply reduced in the cisplatin group (Fig. 2C, D and E).
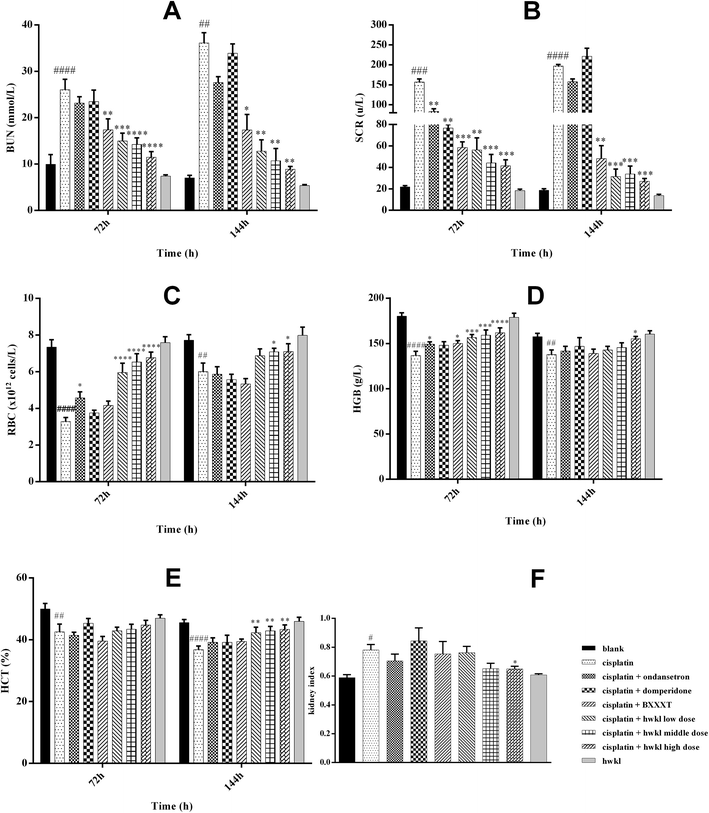 |
| Fig. 2 Effect of HWKL on serum biochemical indicators of kidney (A and B), hematological parameters (C–E) and kidney index (F) in cisplatin-treated rats. Values were represented as mean ± SEM. Serum used to detect BUN and Scr level was collected in 72 h (n = 12) and 144 h (n = 6) after cisplatin administration. Blood used to test RBC (C), HGB (D) and HCT (E) was collected in 72 h (n = 12) and 144 h (n = 6) after cisplatin administration. The orbital blood was added with 15% EDTA-2Na as anticoagulant. Kidney were collected in 168 h (n = 6) after cisplatin administration. The data were analyzed for significant differences using ANOVA by SPSS 19.0. #p < 0.05, ##p < 0.01, ###p < 0.001, ####p < 0.0001 versus blank group. *p < 0.05, **p < 0.01, ***p < 0.001, ****p < 0.0001 versus cisplatin group. | |
HWKL can significantly counter the adverse effects induced by cisplatin administration (Fig. 2 and ESI Tables S2, S3 and S4†). As we observed in our study, food and water consumption increased sharply in the HWKL treatment groups in comparison with the cisplatin group. Drinking water helps accelerate the excretion of cisplatin. Food and water consumption and body weight in the ondansetron and domperidone groups were reduced significantly indicating that ondansetron and domperidone could not significantly help to maintain normal conditions in comparison with the cisplatin group. However, BXXXT and HWKL could contribute to reducing cisplatin-induced side effects but renal injury led to an increase in kidney index, BUN and Scr levels. In addition, renal injury was usually accompanied by hematological changes involving RBC, HGB and HCT. The cisplatin-induced rises in kidney index, BUN level and Scr level were significantly suppressed by HWKL treatment and cisplatin-mediated inhibitions of RBC, HGB and HCT were also reduced by HWKL treatment. Compared with the blank group, the food and water consumption and body weight in the HWKL group were found not to be significantly different which suggested that HWKL alone did not influence body conditions. No hematological and serum biochemical changes were detected in the HWKL group in comparison with the blank group indicating that HWKL alone did not cause nephrotoxicity.
HWKL improves cisplatin-induced renal morphologic damage
It is known that cisplatin is a potent redox cycler that generates toxic reactive oxygen species and, hence, causes oxidative injury to a variety of cells and tissues.5,24 The pathophysiology of cisplatin-induced renal injury can be classified into 4 types of toxicity: tubular toxicity, vascular damage, glomerular injury, and interstitial injury. We examined the tubular damage caused by cisplatin and the potential effect of HWKL by staining kidney specimens with hematoxylin eosin. As shown in A of Fig. 3, the rats in the blank and HWKL groups exhibited normal renal tissue architecture while tubular damage, glomerular injury and interstitial injury were observed in the cisplatin group after cisplatin administration. In the cisplatin + ondansetron, cisplatin + domperidone and cisplatin + BXXXT groups, tubular damage and glomerular injury were clearly apparent. Cisplatin administration produced clear signs of tubular damage, including tubular degeneration, swelling, vacuole formation and necrosis, which were markedly reduced by treatment with HWKL. Little tubular damage was found in HWKL low-dose group and very little tubular damage was found in HWKL middle-dose and high-dose group. To compare the tubular damage caused by cisplatin injection in each treatment group in a quantitative manner, the extent of injury was scored as the percentage of cortical tubular necrosis. As shown in B of Fig. 3, the degree of injury was observed to be significantly increased by cisplatin (4.467 ± 0.1994) compared with the blank group whereas the degree of injury was found to be sharply reduced in the three different HWKL groups suggesting that HWKL could be used to prevent cisplatin-induced tubular injury.
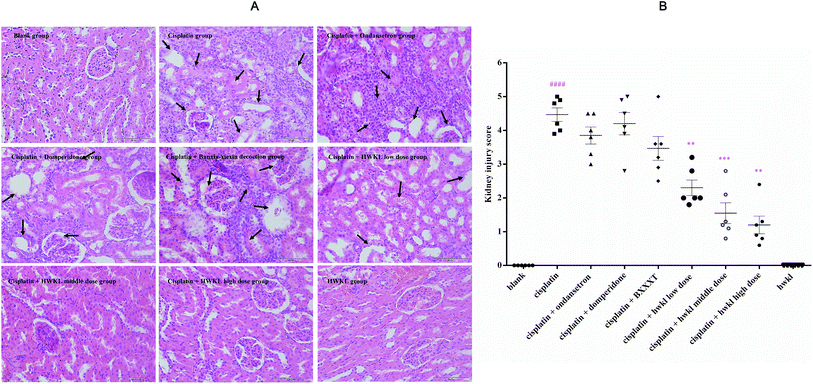 |
| Fig. 3 Effect of HWKL on organ protection of kidney in cisplatin-treated rats by histopathological diagnosis (A). Effect of HWKL on organ protection of kidney in cisplatin-treated rats by histopathological diagnosis based on tubular injury scoring (B). Tissues obtained from treated rats were stained with hematoxylin and eosin. Tissues used in this experiment were collected in 168 h (n = 4). Damaged areas of the tissue sections are marked with black arrows (400× magnification). Tissues of cisplatin + HWKL middle dose group and cisplatin + HWKL high dose group were not observed obvious injury under microscope at 400× magnification. The severity of tubular injury in kidney was quantified by observing stained tissue samples under a light microscope. Tubular injury was scored using the quantitative evaluation method namely tubular damage in HE-stained kidney sections was evaluated and scored based on the percentage of cortical tubular necrosis: 0 = no damage, 1 = 0–10%, 2 = 11–25%, 3 = 26–45%, 4 = 46–75%, 5 = more than 75%. Slides were scored in a blinded manner. Mean scores were calculated by counting 10 different fields for each group. Values were represented as mean ± SEM. The data were analyzed for significant differences using ANOVA by SPSS 19.0. #p < 0.05, ##p < 0.01, ###p < 0.001, ####p < 0.0001 versus blank group. *p < 0.05, **p < 0.01, ***p < 0.001, ****p < 0.0001 versus cisplatin group. | |
HWKL treatment prevents oxidative stress in the kidney of cisplatin-treated rats
It has been reported that cisplatin-induced renal damage is usually related to oxidative stress12,24,25 and GSH-Px, SOD and MDA are considered to be the major parameters associated with in oxidative stress. In order to examine the possible involvement of oxidative stress in the pathogenesis of cisplatin-induced renal injury, relative markers such as renal GSH-Px, SOD and MDA were examined. As demonstrated in Fig. 4, the GSH-Px active level and renal SOD were found to be lower while renal MDA was detected to be higher in the cisplatin group in comparison with the blank group confirming that there was oxidative damage. After HWKL administration, the GSH-Px active level and renal SOD were observed to be up-regulated and renal MDA was found to be down-regulated indicating that HWKL protected organs from being damaged by cisplatin injection through reducing the oxidation level. Also, BXXXT had some effect on resisting oxidative damage caused by cisplatin while ondansetron and domperidone had very little effect.
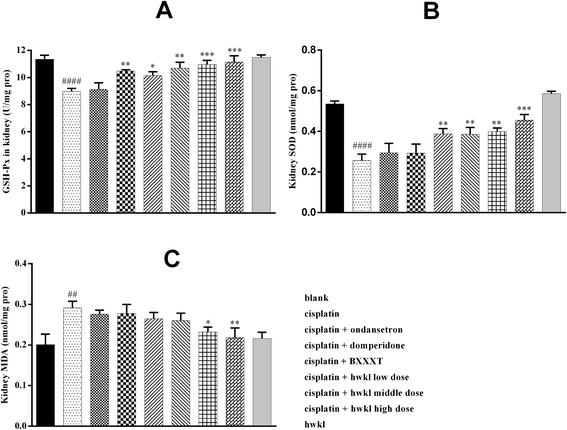 |
| Fig. 4 Effect of HWKL on the GSH-Px (A), SOD (B) and MDA (C) level of kidney in cisplatin-treated rats. Kidney used to detect GSH-Px, SOD and MDA were collected in 168 h after cisplatin administration (n = 6). Values were represented as mean ± SEM. The data were analyzed for significant differences using ANOVA by SPSS 19.0. #p < 0.05, ##p < 0.01, ###p < 0.001, ####p < 0.0001 versus blank group. *p < 0.05, **p < 0.01, ***p < 0.001, ****p < 0.0001 versus cisplatin group. | |
HWKL attenuates cisplatin-induced renal inflammation
Regarding cisplatin-induced nephrotoxicity, it is known that inflammatory factors may contribute to the induction and pathological progression in addition to oxidative factors.10,12 IL-1β, TNF-α and COX-2, as inflammatory cytokines, are considered to play an important role in the induction and pathological progression of cisplatin-related renal damage.11 It has been shown that hydroxyl free radicals produced by cisplatin play an important role in TNF-α synthesis via the P38 signal transduction pathway and activation of COX-2/mPGES-1 signal transduction may be involved in the renal damage caused by cisplatin.12 Furthermore, pro-inflammatory factor IL-1β is known to be increased in cisplatin-induced nephrotoxicity.10 In our study, the IL-1β, TNF-α and COX-2 levels of the cisplatin group in the kidney were found to be higher in comparison with those in the blank group which confirmed that cisplatin-induced renal injury was related to inflammation. Treatment with HWKL can significantly reduce the levels of these three inflammatory cytokines showing that HWKL can protect the kidney from damage by cisplatin through reducing the IL-1β, TNF-α and COX-2 levels. In addition, the levels of IL-1β mRNA and TNF-α mRNA in the kidney of the cisplatin group were significantly higher than in the blank group indicating that the inflammation was related to cisplatin-induced renal injury. HWKL treatment successfully reduced the levels of IL-1β mRNA and TNF-α mRNA in the kidney suggesting that HWKL can protect the kidney from damage by cisplatin by regulating inflammation. The results of IL-1β mRNA and TNF-α mRNA also confirmed the conclusion from a gene level (Fig. 5).
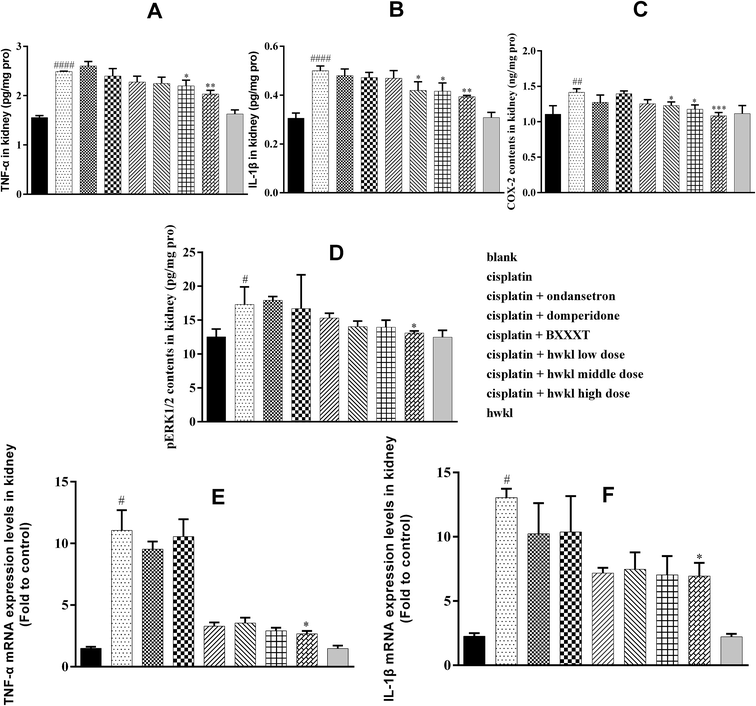 |
| Fig. 5 Effect of HWKL on the inflammatory cytokines, TNF-α level (A), IL-1β level (B), TNF-α mRNA level (E), IL-1β mRNA level (F) and COX-2 level (C) in cisplatin-treated rats. Effect of HWKL on pERK1/2 (D) in cisplatin-treated rats. Kidney used in this part was collected in 168 h after cisplatin administration (n = 6). The contents of TNF-α, IL-1β, pEKR1/2 and COX-2 were detected by using ELISA kits while the contents of TNF-α mRNA and IL-1β mRNA were detected by using qRT-PCR. The contents of TNF-α mRNA and IL-1β mRNA were measured by fold to control. Values were represented as mean ± SEM. The data were analyzed for significant differences using ANOVA by SPSS 19.0. #p < 0.05, ##p < 0.01, ###p < 0.001, ####p < 0.0001 versus blank group. *p < 0.05, **p < 0.01, ***p < 0.001, ****p < 0.0001 versus cisplatin group. | |
Cisplatin administration can increase the content of pERK1/2 in the kidney by activating the MAPK signal pathway. According to (D) of Fig. 5, the pERK1/2 level in the cisplatin group increased sharply after cisplatin injection in comparison with that in the blank group while there was no corresponding rise in the HWKL group showing that HWKL alone did not induce it. After HWKL treatment, the level of pERK1/2 was significantly reduced in the high dose HWKL group suggesting that HWKL could inhibit the unwanted increase in pERK1/2 caused by cisplatin. Unfortunately, ondansetron and domperidone did not have any significant effect on inhibiting the level of pERK1/2 while BXXXT appeared to control the level of pERK1/2 although this effect was not statistically significant.
HWKL reduces cisplatin-induced renal apoptosis
Apoptosis of renal tubular cells contributes to cisplatin-mediated acute kidney injury.11 In other words, cisplatin-mediated renal tubular epithelial cell apoptosis is one of the reasons for cisplatin-induced nephrotoxicity. Cisplatin-induced renal tubular epithelial cell apoptosis may take place through two routes: one is an endogenous pathway mediated by mitochondria, while the other is an exogenous pathway mediated by the death receptor. p53 is considered to play an important role in both routes. As a performer, caspase-3 plays an important role in apoptosis and pro caspase-3 is the source of caspase-3. The protein expression of pro caspase-3 in the cisplatin group was reduced after cisplatin administration while the protein expressions of cleaved caspase-3 and p53 increased in comparison with the corresponding protein levels in the blank group indicating that cisplatin-mediated apoptosis was present in the kidney (Fig. 6). However, treatment with high-dose HWKL significantly inhibited the cisplatin-induced increases in cleaved caspase-3 and p53 protein levels (Fig. 6). The role of p53 in cisplatin-induced nephrotoxicity was further supported by the results indicating that p53 activation in renal tubular cells was related to apoptosis. Unfortunately, ondansetron and domperidone had no effect on regulating these apoptotic factors.
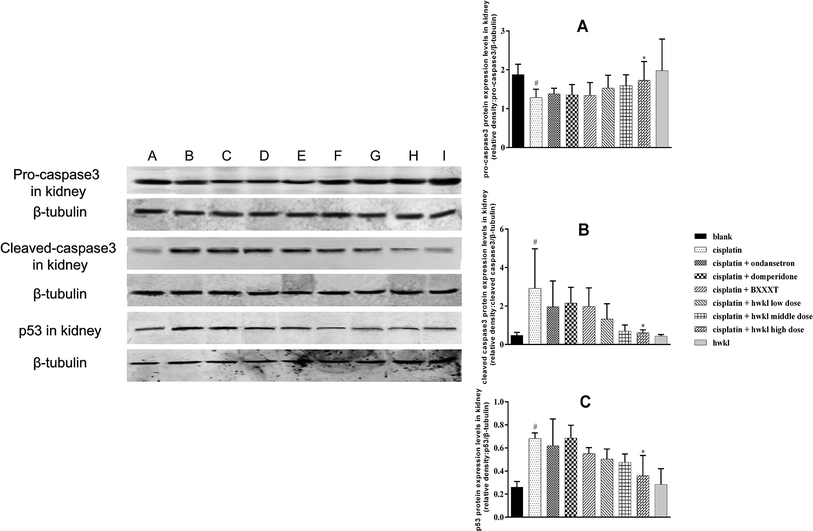 |
| Fig. 6 Effect of HWKL on reducing apoptosis of kidney in cisplatin-treated rats. Kidney used to detect pro caspase-3 (A), cleaved caspase-3 (B) and p53 (C) was collected in 168 h after cisplatin administration (n = 3). The contents of pro caspase-3, cleaved caspase-3 and p53 in kidney were detected by using western blotting. The alphabet A to I represents those nine groups respectively, namely blank group, cisplatin group, cisplatin + ondansetron group, cisplatin + domperidone group, cisplatin + BXXXT group (1.38 g kg−1), cisplatin + HWKL low dose group (1.18 g kg−1), cisplatin + HWKL middle dose group (2.36 g kg−1), cisplatin + HWKL high dose group (4.725 g kg−1), HWKL group (2.36 g kg−1). The contents of pro caspase-3, cleaved caspase-3 and p53 were measured by fold to control. Values were represented as mean ± SEM. The data were analyzed for significant differences using ANOVA by SPSS 19.0. #p < 0.05, ##p < 0.01, ###p < 0.001, ####p < 0.0001 versus blank group. *p < 0.05, **p < 0.01, ***p < 0.001, ****p < 0.0001 versus cisplatin group. | |
HWKL reduces cisplatin-induced myelosuppression
Chemotherapeutic drugs are usually cytotoxic which results in the killing cancer of cells although, unfortunately, the immune system is destroyed at the same time. Cisplatin, as a dose-dependent chemotherapeutic drug, has been reported to induce myelosuppression accompanied by a reduction in white blood cell (WBC) and platelet (PLT) levels. It was found that the WBC, PLT and lymphocyte levels were sharply reduced in the cisplatin group after cisplatin administration which indicated that myelosuppression was present (Fig. 7A–C) and the results of bone marrow DNA relative levels confirmed this (Fig. 7D). Immune dysfunction is often be related to immune organic changes and the spleen and thymus play a crucial role in the immune system. The spleen and thymus indexes of the cisplatin group were found to be significantly reduced after cisplatin administration (Fig. 7E and F). Cisplatin produced a marked reduction in the levels of WBC, PLT, lymphocytes, bone marrow DNA and spleen and thymus indexes were increased by HWKL treatment while ondansetron and domperidone had no effect on improving the levels of these biomarkers. Also, the levels of these biomarkers in the HWKL group were unchanged in comparison with the blank group suggesting that HWKL alone did not induce myelosuppression.
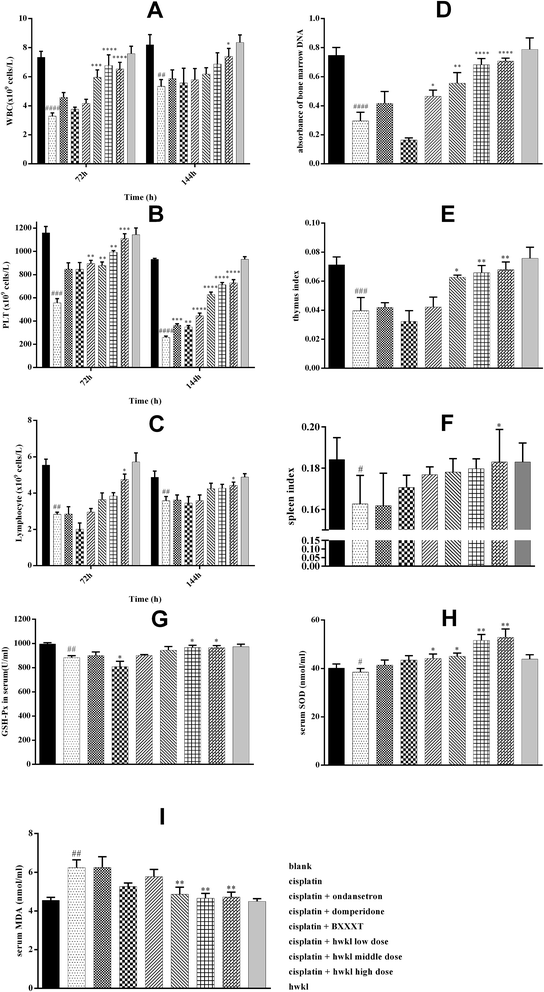 |
| Fig. 7 Effect of HWKL on the immune-related parameters and immune-organ indexes in cisplatin-treated rats. Effect of HWKL on the GSH-Px, SOD and MDA level of serum in cisplatin-treated rats. Values were represented as mean ± SEM. Blood used to test WBC (A), PLT (B) and lymphocyte (C) was collected in 72 h (n = 12) and 144 h (n = 6) after cisplatin administration. The orbital blood was added with 15% EDTA-2Na as anticoagulant. A whole stick of thighbone used to test bone marrow DNA (D) was collected in 168 h (n = 6) after cisplatin administration. The absorbance was used to quantify the relative amount of bone marrow DNA. Thymus (E) and spleen (F) were collected in 168 h (n = 6) after cisplatin administration. Serum used to test GSH-Px (G), SOD (H) and MDA (I) was collected in 144 h (n = 6) after cisplatin administration. The data were analyzed for significant differences using ANOVA by SPSS 19.0. #p < 0.05, ##p < 0.01, ###p < 0.001, ####p < 0.0001 versus blank group. *p < 0.05, **p < 0.01, ***p < 0.001, ****p < 0.0001 versus cisplatin group. | |
Cisplatin-induced oxidative stress is considered to be one of the main causes of myelosuppression.13,26 As demonstrated in (G, H and I) of Fig. 7, the GSH-Px active level and serum SOD were lower while serum MDA was higher in the cisplatin group in comparison with the blank group proving that there was oxidative damage. After HWKL administration, the GSH-Px active level and serum SOD were observed to be up-regulated and serum MDA was detected to be down-regulated indicating that HWKL could ameliorate cisplatin-induced myelosuppression by reducing the oxidation level. In addition, BXXXT had some effect on combatting oxidative damage caused by cisplatin while ondansetron and domperidone had very little effect.
Discussion
Cisplatin is widely used alone or combination to treat a variety of soft tissue neoplasms such as ovarian, testicular, cervical, head and neck, lung and bladder cancer. In order to obtain cure, the dose of cisplatin has been increased in recent treatment protocols because cisplatin is a dose-dependent chemotherapeutic agent.5,10,12,27 High-dose cisplatin causes severe toxicity including emesis, nephrotoxicity and myelosuppression which inhibits its clinical use and causes too much suffering for cancer patients. Nephrotoxicity and myelosuppression also cause severe suffering and reduce patient quality of life. In addition, severe cisplatin-induced nephrotoxicity and myelosuppression reduce the therapeutic effect.
Although many methods of renal protection and other protective approaches are currently being evaluated, the protective effects of such agents are limited, leading to a rationale for developing combined strategies.11 For example, regarding cisplatin-induced nephrotoxicity, hydration therapy is the only option to be used in clinical situations which do not meet the need effectively. TCM has been used for many years to improve life quality by effectively reducing the cisplatin-induced side effects including nephrotoxicity and myelosuppression.2,6,11,13,18,20,24,28–37 There have been no reports of the effect of HWKL on cisplatin-induced nephrotoxicity and myelosuppression despite there being many studies on BXXXT. The herbs involved in HWKL are considered to help improve stomach, kidney and spleen functions due to its properties. In the present study, we report for the first time that systemic administration of HWKL, a product obtained using modern techniques and based on a modified ancient TCM formula according to clinical indications, sharply reduces cisplatin-induced emesis, renal damage, gastrointestinal injury and myelosuppression.
Increased levels of BUN and Scr have been reported in both acute and chronic cisplatin-induced renal toxicity. As a pair of sensitive biomarkers in kidney injury, BUN and Scr levels are used to indicate renal injury. Water intake can be inhibited by severe nephrotoxicity and, as a result, water balance might be adversely affected and, as a results, normal balance can be disrupted due to sharply reduced food intake and body weight. In addition, cisplatin-induced inflammation causes hematological changes accompanied by reduced RBC, HCT and HGB levels which may be related to oxidative stress due to the results of GSH-Px, SOD and MDA in serum. During the last decade, oxidative stress has been confirmed as an important factor involved in cisplatin-induced renal damage. A variety of increased reactive oxygen species (ROS) can be produced during cisplatin treatment of cultured renal tubular cells, kidney slices and in in vivo studies in animals. An imbalance in ROS production is reflected in oxidative stress. Moreover, oxidative stress shows the ability of a biological system to readily counteract the effect of a pro-oxidant.38–43 It has been reported that cisplatin can alter the redox balance in cells, leading to ROS production. According to previous reports, ROS play a vital role in the activation of several important signaling pathways during cisplatin nephrotoxicity, for example, p38 and p53 activation signaling pathways.44 All in all, ROS may be one of the early signals that are at least partially responsible for the activation of various signaling pathways, culminating in renal injury and death following cisplatin-induced kidney damage. Therefore, reducing oxidative stress helps inhibit cisplatin-induced nephrotoxicity. Flavonoids in SR and GHR, such as baicalin (16 in Fig. 1) and scutellarin (7 in Fig. 1), are effective antioxidants. Also, the effect of inhibiting oxidative stress of alkaloids in CR, such as berberine (18 in Fig. 1) and coptisine (11 in Fig. 1), has been confirmed45,46 and these conclusions were also supported by the results of our study (Fig. 4).
On the basis of previous studies, ROS generated by cisplatin may activate the nuclear factor kappa B (NF-κB) pathway and transcription of genes responsible for inflammation. In addition, there is increasing evidence which indicates that cisplatin induces a wide variety of inflammatory cytokines and chemokines including translocation of the redox-sensitive transcription factor NF-κB from the cytosol to the nucleus.47–50 As a result, the expression of several proinflammatory genes, including IL-1β, TNF-α and COX-2, was triggered. In the inflammatory response triggered by cisplatin, TNF-α appears to be a key upstream regulator. TNF-α induces a variety of cellular reposes ranging from inflammation to cell death through engaging the cell surface receptors TNFR1 and TNFR2 acting as a pleiotropic cytokine. According to subsequent reports, it was found that TNF-α is critical to the induction of proinflammatory factors and recruitment of inflammatory cells. Overall, TNF-α has an important effect in producing the proinflammatory response during cisplatin-induced nephrotoxicity and the ensuing kidney injury and acute renal failure. Unlike TNF-α, the action of IL-1β in cisplatin-induced kidney injury is not fully understood. However, as we know, IL-1β is not constitutively expressed and its secretion is largely restricted to circulating monocytes. As a consequence, IL-1β mainly contributes to systemic inflammation by initiating acute phase response proteins in the liver such as C-reactive protein, by activating endothelial cells, triggering fever, causing neutrophil mobilization from the bone marrow (leukocytosis), and activating all classes of leukocytes and renal cells. Our results have provided additional evidence to prove that IL-1β may play a role in cisplatin-induced renal injury.47–50 However, further studies are needed to confirm the significant role of IL-1β in cisplatin-induced nephrotoxicity. At present, COX-2 has been confirmed to play a vital role in cisplatin-induced kidney damage. In addition, activation of MAPK, another important inflammatory signaling pathway, contributes to an increase in the content of TNF-α resulting in aggravation of cisplatin-induced nephrotoxicity. Also, cisplatin can up-regulate pERK1/2 leading to severe kidney injury. In summary, it could have a marked beneficial effect on cisplatin-induced kidney damage by reducing the increase of cisplatin-mediated inflammatory cytokines and inhibiting the action of pERK1/2 in the kidney.47–50
Cisplatin-induced apoptosis has been confirmed to play an important mode in nephrotoxicity. In the mitochondrial pathway, oxidative stressors initiate the pathway resulting in caspase 9 activation then, caspase 9 activates the principal executioner caspase, namely caspase 3, in renal tubule apoptosis. In addition, p53 may also induce apoptosis by directly activating proapoptotic Bcl-2 family proteins, leading to mitochondrial injury and the release of apoptogenic factors. In addition, TNF-α has an important effect in mediating cisplatin-induced kidney damage. It induces apoptosis and produces ROS in the kidney. Engagement of cell surface receptors with extracellular TNF-α can activate caspase 8 that can also activate caspase 3 resulting in apoptosis in an extrinsic pathway. Moreover, it has also been reported that p53 has a vital role in the extrinsic pathway by controlling fatty acid synthase (Fas).24,42,48 Accordingly, the mitochondrial pathway and the extrinsic pathway do not work alone. In summary, HWKL inhibits cisplatin-induced nephrotoxicity via these two pathways.
After cisplatin administration, immune organs, such as the spleen and thymus, are damaged according to the spleen index and thymus index results. Consequently, humoral and cellular immunity may be disrupted. Also, hematological changes including WBC, PLT and lymphocytes together with the sharply reduced bone marrow DNA confirm this conclusion. It has been reported that cisplatin-induced oxidative stress plays an important role in producing myelosuppression.51–55 Therefore, reducing the severity of cisplatin-induced oxidative stress might have an effect on inhibiting myelosuppression. We found that flavonoids and alkaloids in HWKL can reduce cisplatin-induced oxidative stress and apart from these, ginsenosides in GR (such as 19, 20, 26 in Fig. 1) are regarded as excellent antioxidants and immunopotentiators. In addition, the polysaccharides in the seven herbs involved in HWKL are considered to be effective in improving immune function.56–62 In summary, HWKL reduces cisplatin-induced myelosuppression by reducing oxidative stress and improving immune function.
In our previous study, pica and anorexia were also found in the groups treated with cisplatin (7 mg kg−1). In order to find whether reduced gastrointestinal side effects may be helpful in kidney recovery because of increased food and water intake, ondansetron and domperidone were chosen as positive drugs due to their actions in reducing cisplatin-induced side effects including vomiting and gastrointestinal dysfunction in our study.27 We hypothesized they may have an effect on cisplatin-induced nephrotoxicity and myelosuppression due to the fact that they can improve cisplatin-induced adverse effects to some extent. Unfortunately, the results obtained in our study did not show that ondansetron and domperidone had a significant effect in reducing cisplatin-induced renal damage and myelosuppression. The relationship between gastrointestinal disorders and renal damage was not clearly due to the negative effects on kidney of ondansetron and domperidone. So, further studies are needed to examine whether they have an effect on cisplatin-induced kidney injury and myelosuppression.
Conclusion
In summary, the results described above demonstrate that multiple pathways are involved in cisplatin-induced nephrotoxicity and myelosuppression. It is necessary for the production of a therapeutic effect by a drug or TCM formulation that there is simultaneous inhibition of the various signaling pathways involved in cisplatin-induced nephrotoxicity and myelosuppression. Although many studies have been performed to evaluate methods intended to reduce cisplatin-induced toxicity, the reported protective effects of those procedures have been incomplete and so it is necessary to seek alternative methods with greater efficacy. In our research, we showed for the first time that HWKL exhibits significant protective effects against cisplatin-induced nephrotoxicity and myelosuppression. We also found basic evidence for possible mechanism involved in the protective effects of HWKL. However, further future studies are needed to identify the exact mechanisms and active compounds responsible for the protective effect of HWKL.
Conflicts of interest
All the authors of this manuscript state that they have no conflicts of interest.
Abbreviations
BXXXT | Banxiaxiexin Tang |
HWKL | He-Wei granules |
PR | Pinelliae Rhizoma Praeparatum |
ZR | Zingiberis rhizoma |
GR | Ginseng Radix et Rhizoma |
SR | Scutellariae radix |
CR | Coptidis rhizoma |
GHR | Glycyrrhizae Radix et Rhizoma Praeparata Cum Melle |
JF | Jujubae fructus |
ZB | Zingiberis Rhizoma Recens |
TNF-α | Tumor necrosis alpha |
IL-1β | Interleukin-1 beta |
COX-2 | Cyclooxygenase-2 |
ERK | Extracellular signal-regulated kinase |
ELISA | Enzyme linked immunosorbent assay |
MDA | Malonaldehyde |
SOD | Superoxide dismutase |
GSH-PX | Glutathione peroxidase |
RBC | Red blood cell |
HCT | Red blood cell specific volume |
HGB | Hemoglobin |
WBC | White blood cell |
PLT | Platelet |
References
- G. J. Dugbartey, L. J. Peppone and I. A. de Graaf, Toxicology, 2016, 371, 58–66 CrossRef CAS PubMed.
- A. Basu, A. Bhattacharjee, A. Samanta and S. Bhattacharya, Drug Chem. Toxicol., 2016, 1–9, DOI:10.1080/01480545.2016.1237522.
- M. Sooriyaarachchi, W. M. White, A. Narendran and J. Gailer, Metallomics, 2014, 6, 532–541 RSC.
- M. Kudo, Y. Yamamoto, Y. Koga, T. Hamaguchi, T. Akimoto, M. Yasunaga and Y. Matsumura, Mol. Clin. Oncol., 2016, 5, 817–822 Search PubMed.
- A. W. Prestayko, J. C. D' Aoust, B. F. Issell and S. T. Crooke, Cancer Treat. Rev., 1979, 6, 17–39 CrossRef CAS PubMed.
- Y. Topcu-Tarladacalisir, M. Sapmaz-Metin and T. Karaca, Renal Failure, 2016, 38, 1741–1748 CrossRef CAS PubMed.
- W. Lam, Sci. Transl. Med., 2010, 2, 45–59 Search PubMed.
- F. Qi, BioSci. Trends, 2010, 4, 297–307 Search PubMed.
- F. Qi, L. Zhao, A. Zhou, B. Zhang, A. Li, Z. Wang and J. Han, BioSci. Trends, 2015, 9, 16–34 CrossRef PubMed.
- N. Pabla and Z. Dong, Kidney Int., 2008, 73, 994–1007 CrossRef CAS PubMed.
- Z. Y. Teng, X. L. Cheng, X. T. Cai, Y. Yang, X. Y. Sun, J. D. Xu, W. G. Lu, J. Chen, C. P. Hu, Q. Zhou, X. N. Wang, S. L. Li and P. Cao, Sci. Rep., 2015, 5, 15592 CrossRef CAS PubMed.
- X. Yao, K. Panichpisal, N. Kurtzman and K. Nugent, Am. J. Med. Sci., 2007, 334, 115–124 CrossRef PubMed.
- M. Zenitani, T. Nojiri, T. Kimura, H. Hosoda, K. Miura, J. Hino, K. Nakahata, S. Uehara, M. Miyazato, T. Oue, H. Okuyama and K. Kangawa, Cancer Chemother. Pharmacol., 2017, 79, 363–368 CrossRef CAS PubMed.
- H. Lu, S. Ma, Y. Guo, J. Cai, X. Wang and W. Ye, Chin. Arch. Tradit. Chin. Med., 2009, 27, 1082–1084 Search PubMed.
- H. X. Yu and D. S. Wang, Chin. Arch. Tradit. Chin. Med., 2009, 27, 1537–1540 Search PubMed.
- R. Xue, Y. Cao, N. Han, X. Lin, Z. Liu and J. Yin, J. Ethnopharmacol., 2011, 137, 1156–1160 CrossRef PubMed.
- Y. Li, B. Zhang, Y. Lai and Z. Chen, Chin. J. Exp. Tradit. Med. Formulae, 2014, 20, 180–184 Search PubMed.
- G. Chen, Y. Yang, M. Liu, Z. Teng, J. Ye, Y. Xu, X. Cai, X. Cheng, J. Yang, C. Hu, M. Wang and P. Cao, J. Ethnopharmacol., 2015, 166, 149–156 CrossRef PubMed.
- L. L. Yan, J. W. Shi, J. F. Wang and Y. Shi, Acta Pharmacol. Sin., 2013, 48, 526–531 CAS.
- L. Q. Zhao, S. S. Zhang, Z. F. Wang and A. Wiebrecht, DT. Ztschr. F. Akupunktur., 2013, 56, 30–31 CrossRef.
- X. Liu, X. L. Wang, L. Wu, H. Li, K. M. Qin, H. Cai, K. Pei, T. Liu and B. C. Cai, J. Ethnopharmacol., 2014, 154, 606–612 CrossRef PubMed.
- W. Zhang, M. W. Saif, G. E. Dutschman, X. Li, W. Lam, S. Bussom, Z. Jiang, M. Ye, E. Chu and Y.-C. Cheng, J. Chromatogr. A, 2010, 1217, 5785–5793 CrossRef CAS PubMed.
- L. Y. Du, D. W. Qian, E. X. Shang, P. Liu, S. Jiang, J. M. Guo, S. L. Su, J. A. Duan, J. Xu and M. Zhao, J. Ethnopharmacol., 2015, 169, 156–162 CrossRef CAS PubMed.
- R. Khan, A. Q. Khan, W. Qamar, A. Lateef, M. Tahir, M. U. Rehman, F. Ali and S. Sultana, Toxicol. Appl. Pharmacol., 2012, 258, 315–329 CrossRef CAS PubMed.
- S. Sultana, K. Verma and R. Khan, J. Pharm. Pharmacol., 2012, 64, 872–881 CrossRef CAS PubMed.
- A. I. ElKady and W. S. Ramadan, Biomed. Pap., 2016, 160, 363–371 Search PubMed.
- K. Yamamoto, M. Nakai, K. Nohara and A. Yamatodani, Eur. J. Pharmacol., 2007, 554, 34–39 CrossRef CAS PubMed.
- H. H. Arab, W. R. Mohamed, B. M. Barakat and S. A. Arafa el, Chem.–Biol. Interact., 2016, 258, 205–213 CrossRef CAS PubMed.
- C. S. Chao, C. S. Tsai, Y. P. Chang, J. M. Chen, H. K. Chin and S. C. Yang, Int. Immunopharmacol., 2016, 40, 517–523 CrossRef CAS PubMed.
- Z. Farooqui, F. Ahmed, S. Rizwan, F. Shahid, A. A. Khan and F. Khan, Biomed. Pharmacother., 2017, 85, 7–15 CrossRef CAS PubMed.
- S. M. Hassan, M. M. Khalaf, S. A. Sadek and A. M. Abo-Youssef, Pharm. Biol., 2017, 55, 766–774 CrossRef CAS PubMed.
- X. He, C. Li, Z. Wei, J. Wang, J. Kou, W. Liu, M. Shi, Z. Yang and Y. Fu, Eur. J. Pharmacol., 2016, 789, 215–221 CrossRef CAS PubMed.
- V. A. K., R. M. Madhana, E. R. Kasala, P. K. Samudrala, M. Lahkar and R. Gogoi, J. Biochem. Mol. Toxicol., 2016, 30, 571–579 CrossRef PubMed.
- K. Kaltalioglu and S. Coskun-Cevher, Renal Failure, 2016, 38, 1291–1299 CrossRef CAS PubMed.
- W. Li, M. H. Yan, Y. Liu, Z. Liu, Z. Wang, C. Chen, J. Zhang and Y. S. Sun, Nutrients, 2016, 8, 566 CrossRef PubMed.
- R. Rezaee, A. A. Momtazi, A. Monemi and A. Sahebkar, Pharmacol. Res., 2017, 117, 218–227 CrossRef CAS PubMed.
- M. R. Sepand, M. H. Ghahremani, K. Razavi-Azarkhiavi, M. Aghsami, J. Rajabi, H. Keshavarz-Bahaghighat and M. Soodi, J. Pharm. Pharmacol., 2016, 68, 1222–1232 CrossRef CAS PubMed.
- M. A. Darwish, A. M. Abo-Youssef, M. M. Khalaf, A. A. Abo-Saif, I. G. Saleh and T. M. Abdelghany, J. Biochem. Mol. Toxicol., 2017, 31, 1–9 CrossRef CAS PubMed.
- A. H. Eid, N. F. Abdelkader, O. M. Abd El-Raouf, H. M. Fawzy and E. S. El-Denshary, Arch. Pharmacal Res., 2016, 39, 1693–1702 CrossRef CAS PubMed.
- L. Gao, W. F. Wu, L. Dong, G. L. Ren, H. D. Li, Q. Yang, X. F. Li, T. Xu, Z. Li, B. M. Wu, T. T. Ma, C. Huang, Y. Huang, L. Zhang, X. Lv, J. Li and X. M. Meng, Front. Pharmacol., 2016, 7, 479 Search PubMed.
- K. Hosohata, Int. J. Mol. Sci., 2016, 17, 1826 CrossRef PubMed.
- A. Leekha, B. S. Gurjar, A. Tyagi, M. A. Rizvi and A. K. Verma, J. Cancer Res. Clin. Oncol., 2016, 142, 2503–2514 CrossRef CAS PubMed.
- M. Yan, C. Tang, Z. Ma, S. Huang and Z. Dong, Toxicol. Appl. Pharmacol., 2016, 313, 104–108 CrossRef CAS PubMed.
- G. Ramesh and W. B. Reeves, Am. J. Physiol. Ren. Physiol., 2005, 289, F166–F174 CrossRef CAS PubMed.
- R. Domitrovic, O. Cvijanovic, E. Pernjak-Pugel, M. Skoda, L. Mikelic and Z. Crncevic-Orlic, Food Chem. Toxicol., 2013, 62, 397–406 CrossRef CAS PubMed.
- J. Song, T. Lu, L. Xie and X. D. Liu, Chin. J. Exp. Tradit. Med. Formulae, 2010, 4, 118–122 Search PubMed.
- H. J. Anders, J. Am. Soc. Nephrol., 2016, 27, 2564–2575 Search PubMed.
- I. Potocnjak and R. Domitrovic, Food Chem. Toxicol., 2016, 98, 251–261 CrossRef CAS PubMed.
- G. S. Saribas, D. Erdogan, G. Goktas, S. N. Akyol, I. M. Hirfanoglu, S. G. Gurgen, N. Coskun and C. Ozogul, J. Obstet. Gynaecol., 2016, 36, 1086–1092 CrossRef CAS PubMed.
- E. Vacchelli, F. Aranda, F. Obrist, A. Eggermont, J. Galon, I. Cremer, L. Zitvogel, G. Kroemer and L. Galluzzi, OncoImmunology, 2014, 3, e29030 CrossRef PubMed.
- S. Cao, S. Wang, H. Ma, S. Tang, C. Sun, J. Dai, C. Wang, Y. Shu, L. Xu, R. Yin, X. Song, H. Chen, B. Han, Q. Li, J. Wu, C. Bai, J. Chen, G. Jin, Z. Hu, D. Lu and H. Shen, Pharmacogenomics J., 2016, 16, 41–46 CrossRef CAS PubMed.
- de Carvalho Maroni, A. C. de Oliveira Silveira, E. A. Leite, M. M. Melo, A. F. de Carvalho Ribeiro, G. D. Cassali, C. M. de Souza, E. M. Souza-Fagundes, I. R. Caldas, M. S. Araujo, O. A. Martins-Filho, M. C. de Oliveira and A. Teixeira-Carvalho, Exp. Biol. Med., 2012, 237, 973–984 CrossRef PubMed.
- J. Guo, B. Wang, R. X. Rong and Z. R. Cao, Food Sci., 2015, 36, 200–204 CAS.
- O. F. Khabour, K. H. Alzoubi, D. S. Mfady, M. Alasseiri and T. F. Hasheesh, Int. J. Clin. Exp. Med., 2014, 7, 982–988 Search PubMed.
- S. Sinha, M. Jothiramajayam, M. Ghosh, A. Jana, U. Chatterji and A. Mukherjee, Food Chem. Toxicol., 2015, 81, 120–128 CrossRef CAS PubMed.
- H. J. Guo and D. G. Wei, J. Anhui Agric. Sci., 2010, 38, 19341–19345 CAS.
- J. Hu, M. Z. Ao, Y. M. Cui, J. Cao, W. Li and L. J. Yu, Nat. Prod. Res. Dev., 2008, 20, 911–913 Search PubMed.
- Y. Li, W. Wang, D. K. Yin and X. D. Gao, J. Anhui Univ. Chin. Med., 2015, 34, 66–69 CAS.
- M. J. Liu, F. Wang, Y. Zhang, L. Wang, S. M. Zhang and Y. Liang, J. Chin. Inst. Food Sci. Technol., 2016, 16, 52–58 Search PubMed.
- L. H. Ma and W. D. Qin, Sci. Technol. Food Ind., 2010, 31, 120–124 CAS.
- M. J. T. Remila, Y. Y. Cong, A. B. L. Z. Palida, Y. K. F. Mirensha and W. H. Zhao, Chin. Arch. Tradit. Chin. Med., 2016, 34, 1647–1649 Search PubMed.
- C. Wang, Y. M. Wang, F. Guo, K. R. Zhang and A. P. Chi, J. Northwest Univ., 2015, 45, 73–78 Search PubMed.
Footnote |
† Electronic supplementary information (ESI) available. See DOI: 10.1039/c7ra02830j |
|
This journal is © The Royal Society of Chemistry 2017 |
Click here to see how this site uses Cookies. View our privacy policy here.