DOI:
10.1039/C7RA02525D
(Paper)
RSC Adv., 2017,
7, 19780-19786
Compositional engineering of the pi-conjugated small molecular VOPcPhO
:
Alq3 complex to boost humidity sensing†
Received
1st March 2017
, Accepted 26th March 2017
First published on 4th April 2017
Abstract
This study exhibits a solution-processed organic semiconductor humidity sensor based on vanadyl 2,9,16,23-tetraphenoxy-29H,31H-phthalocyanine (VOPcPhO), tris-(8-hydroxy-quinoline)aluminum (Alq3), and their composites. Compositional engineering of the VOPcPhO
:
Alq3 complex was performed to develop a sensitive humidity sensor with a linear response. Thin films of VOPcPhO, Alq3, and composites were spin-coated over pre-deposited aluminum (Al) electrodes, whereas the other electrodes were deposited through a thermal evaporation technique. Both capacitive and resistive responses were measured as a function of different relative humidity levels. Morphological and structural properties of the organic thin films were characterized by atomic force microscopy (AFM), field emission scanning electron microscopy (FESEM), and Fourier transform infrared spectroscopy (FTIR). Compared to the VOPcPhO and Alq3 stand-alone sensors, the VOPcPhO
:
Alq3 composite-based sensor demonstrated superior performance with significantly improved sensing parameters, highlighting unique advantages of the low-molecular composite-based thin film organic humidity sensors.
Introduction
Over the last couple of decades, researchers in the field of sensors have been attracted to the field of organic based sensing devices due to their low production cost, simple and easy fabricating technologies, and their multi-functionality over a single substrate. Organic materials are very sensitive towards moisture and are good applicants to fabricate sensors for humidity measurements.1–4 In order to employ a humidity sensor in a wide range of applications, it must possess superior sensitivity, slight hysteresis, short response time, adequate durability, and a low manufacturing cost.5,6 However, in the current scenario, researchers are struggling to introduce correct material combinations which possess outstanding properties of superior sensitivity and stability, while preserving a low manufacturing cost, a moderately simple processing method, and eco-friendly techniques.4,7–9 Even though researchers have managed to introduce various simple fabricating processes, which can be coupled with physical and chemical flexibility from polymer organic-based materials, mass production of these types of sensing devices seems promising only once their other limitations have been overcome.
Humidity is a universal phenomenon because of the general presence of moisture contents in air. Control or measurement of humidity is becoming more important, not only for human well-being but also for various industrial applications such as meteorology, process control techniques, agriculture, and medical equipment. Based on requirements or applications, various kinds of humidity sensors are used and they can be classified based on their sensing mechanisms. Humidity sensors based on inorganic materials10,11 are costly, and require high power for operation, whereas organic material-based humidity sensors have advantages of low cost, light weight, flexibility, and simple technology.
Even though organic materials are highly responsive to humidity, many of them are easily dispersed in water12,13 which makes them impractical in highly humid conditions. Among organic materials, the phthalocyanines group has hydrophobic properties and is insoluble in water and hence, is considered as a good candidate for humidity meters.14 Phthalocyanines are macro-cyclic and hetero-cyclic compounds which exhibit a ring structure containing nitrogen atoms in addition to carbon as a part of the ring. Most phthalocyanines are considered as p-type semiconductors15 and they possess sufficiently good charge carrier mobility16 and significant conductivity for utilization in potential applications with organic electronic devices. Among the phthalocyanines, VOPcPhO is available as a green colored dye that is insoluble in water. It exhibits very good chemical and environmental stability and has been extensively studied for various types of sensors17–21 whereas Alq3 is a pi-conjugated small molecular material.22 In pi-conjugated materials, single and double or single and triple bonds alternate throughout the backbone of a molecule. The second and third bonds of a double or triple bond are pi-bonds.23 The gap between LUMO and HOMO is typically in the 1.5–3 eV range, i.e. the materials are organic semiconductors.23 Even though Alq3 is mostly utilized as an exciton blocking layer and a light emitting layer in photovoltaic applications, it can be utilized to obtain a high-quality thin film with high electron mobility by a simple spin-coating method.24
In this work, the compositional engineering of the pi-conjugated small molecular VOPcPhO
:
Alq3 complex was performed to develop a sensitive humidity sensor with a linear response. The bulk heterojunction of the two materials was expected to have the characteristics of the both components and is assumed to be more linear in response towards humidity than VOPcPhO and more sensitive than Alq3 alone. The aim of this research is to boost the humidity sensing potential of VOPcPhO by making its complex with Alq3 to develop a cheap and reliable sensing element for assessing humidity in the surrounding environment.
Experimental work
Tris-(8-hydroxyquinoline)aluminum (Alq3) and vanadyl 2,9,16,23-tetraphenoxy-29H,31H-phthalocyanine (VOPcPhO) were purchased from Sigma-Aldrich (USA). Fig. 1a and b shows their molecular structures, respectively. Surface type humidity sensing devices were fabricated from glass slides which were cleaned with acetone for 10 min followed by cleaning with ethanol for another 10 min in an ultrasonic cleaner. Afterwards, the slides were washed with distilled water and dried with a N2 blower. Then the substrates were processed in an oxygen plasma etcher for 5 min under a pressure of 1 mbar followed by an additional 5 min plasma cleaning in 10−3 mbar pressure inside a vacuum thermal evaporator. Later, 100 nm thick aluminum electrodes were deposited with equal inter-electrode gaps of 40 μm, over which the active layers were deposited using a spin coater (Laurell WS-650-23NPP) at 2500 rpm and an acceleration of 500 m s−2. The fabricated surface type sensors' schematic diagram is shown in Fig. 1d. Al/active layer/Al sensors consist of stand-alone films of VOPcPhO and Alq3 plus their composite in various proportions (i.e. 2
:
1, 1
:
1 and 1
:
2). Samples were assessed in the setup of a humidity controlled chamber as shown in Fig. 1c. Thicknesses of thin films were in the range (composite-raw material) of ∼200 nm measured by a KLA-TENCOR P6 Surface Profiler. The devices were annealed at different temperatures, i.e. 75, 100, and 125 °C for 20 min and then cooled down gradually by WiseStir heating. For capacitance-relative humidity and resistance-relative humidity measurements a INSTEK LCR-819 meter was used. A Veeco Dimension 3000 AFM was utilized to measure surface morphology of the organic thin films. Sensors were characterized for their humidity response, w.r.t capacitance, and resistance.
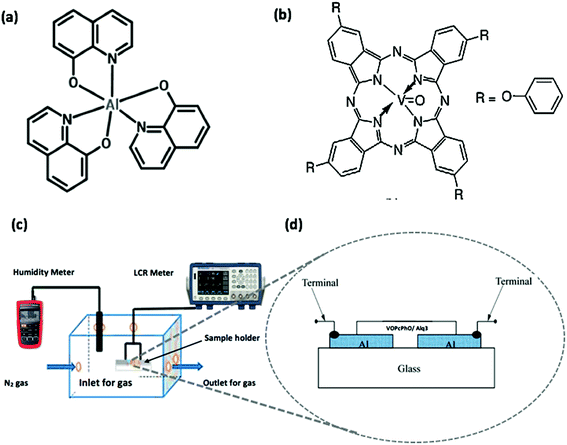 |
| Fig. 1 The molecular structure of (a) tris-(8-hydroxyquinoline)aluminum (Alq3) and (b) vanadyl 2,9,16,23-tetraphenoxy-29H,31H-phthalocyanine (VOPcPhO). (c) Setup of the humidity chamber and (d) schematic diagram of surface type sensors. | |
Results and discussion
A well-organized study was carried out to optimize various parameters, including the composition and annealing temperature of VOPcPhO, Alq3, and VOPcPhO
:
Alq3 composite humidity sensors. Capacitance responses of the fabricated sensors toward changes in relative humidity levels are shown in Fig. 2. The humidity dependent sensitivity of the sensors was investigated with respect to variation in RH% from 15–95% at different annealing temperatures, i.e. 25, 75, 100, and 125 °C to obtain the best optimized device. We observed that the best sensitivity in the case of Alq3 was obtained at 125 °C and at this temperature the sensitivity was ∼0.3125 pF/RH% as shown below in Fig. 2a. The device is more responsive in the range of 35–95% RH. On the other hand, Fig. 2b shows results of the VOPcPhO devices, which were also annealed at different temperatures for the same time, and these samples exhibit sensitivity in the range of 55–95% RH. The VOPcPhO showed higher sensitivity at 25 °C (1.06 pF/RH%) as compared to other annealing temperatures. While the best concentration of VOPcPhO
:
Alq3 was found as 1
:
2 (V1
:
A2), it showed a more augmented response at 75 °C as shown in Fig. 2c. It is important to note that the Alq3 exhibits a more linear response than the VOPcPhO, whereas the VOPcPhO exhibits more sensitivity at higher humidity levels as compared to the Alq3. Hence, to retain greater sensitivity at higher RH levels, the ratio V1
:
A2 was selected. Both these properties are very important for a good sensor. The bulk heterojunction of both materials is expected to consist of both characteristics and is assumed to be more linear than VOPcPhO and more sensitive than Alq3 alone. Thus, V1
:
A2 was selected on the basis of results presented in the ESI file (Fig. S1†). The V1
:
A2 based devices were also annealed at different temperatures and their most sensitive response was found to be 0.32 pF/% at 75 °C. The most responsive behavior of the device is seen from 40–95% RH. A ratio of VOPcPhO
:
Alq3 at 1
:
2 was more humidity sensitive than the rest of the samples. Hence, the optimum concentration was selected as 1
:
2. Fig. S3† shows the best selected capacitance vs. humidity sensing behavior for Alq3 VOPcPhO and composite.
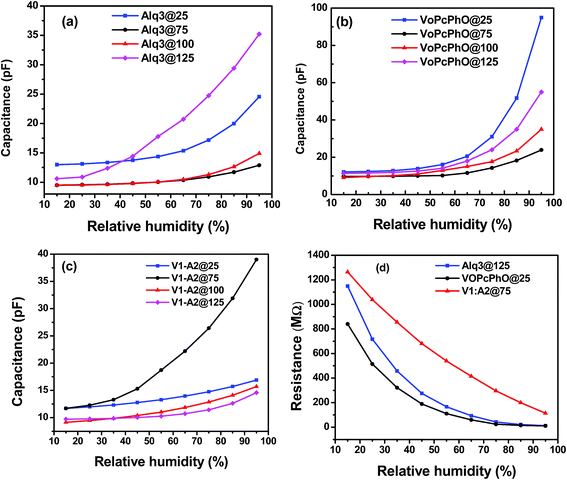 |
| Fig. 2 Capacitance vs. humidity sensing behavior of (a) Alq3, (b) VOPcPhO, (c) composite (VOPcPhO : Alq3) at various ambient temperatures, and (d) resistance response of the best selected sensors i.e. composite (V1 : A2)@75 °C, Alq3@125 °C, and VOPcPhO@25 °C. The capacitance measurement was recorded at 100 Hz frequency and the frequency–capacitance response is presented in Fig. S2 in a ESI file.† Normalized capacitance to temperature response of the desired composite at (a) 25 °C and (b) 75 °C is shown in Fig. S4.† | |
Many factors, including porosity of the sensing films, dielectric constant, the material's dynamic sensing area, and polarizability, significantly participate in capacitance/resistance variation in response to ambient relative humidity levels. Relative permittivity of organic-based materials with small molecular sizes lies between 4–8;25 on the other hand, water permittivity is ∼80.4 The dielectric constant of water remarkably increases capacitance of a sensor because of a large difference between the relative permittivity of water and active thin film of respective materials and their composites. Fig. 2d shows the relationship between resistances vs. relative humidity levels for the composites (V1
:
A2)@75 °C, Alq3@125 °C, and VOPcPhO@25 °C based humidity sensors. The sensitivity of composite (V1
:
A2), Alq3, and VOPcPhO was found to be 14.5, 12.81, and 9.68, respectively. It was observed that the resistance responses of VOPcPhO and Alq3 were more obvious in the range of 15–60% RH; after that they seem to tend to be saturated values. However, in the case of V1
:
A2, the resistive device was sensitive over the whole measured range.
Surface morphology of thin films of Alq3, VOPcPhO, and their composites was studied using atomic force microscopy (AFM) and field emission scanning electron microscope (FESEM). Fig. 3 shows the AFM and FESEM micrographs of VOPcPhO annealed at@25 °C (Fig. 3a and b), Alq3 annealed at@125 °C (Fig. 3c and d), and composite VOPcPhO
:
Alq3 annealed at@75 °C (Fig. 3e and f), respectively. Micrographs of the composite films (Fig. 3e and f) display a rough surface with uniform distribution of the textured appearance. The image of Fig. 3e indicates that the Alq3
:
VOPcPhO composite thin film, when annealed at 75 °C, results in much rougher surface with a sharp peak like structures. The rms roughness measured for VOPcPhO and Alq3 thin films were 0.832 nm and 0.597 nm, respectively. The composite film V1
:
A2 has rms roughness of 0.937 nm which exhibits a slightly rougher surface than the stand-alone films. The annealed composite thin film provides more absorption sites for moisture content to be adsorbed. Sensitivity of the humidity sensors depends upon the amount of water absorbed corresponding to the increased surface area. It can be inferred that the greater roughness with uniform distribution leads to larger surface area exposure and more moisture adsorption as well.26 Sensitivity (S) of the humidity sensors can be assessed by using the following equations:27 S(R) = ΔR/ΔRH and S(C) = ΔC/ΔRH, where ΔR, ΔC and ΔRH are increments in resistance, capacitance, and change in the relative humidity, respectively. A summary of the devices is described in Table 1.
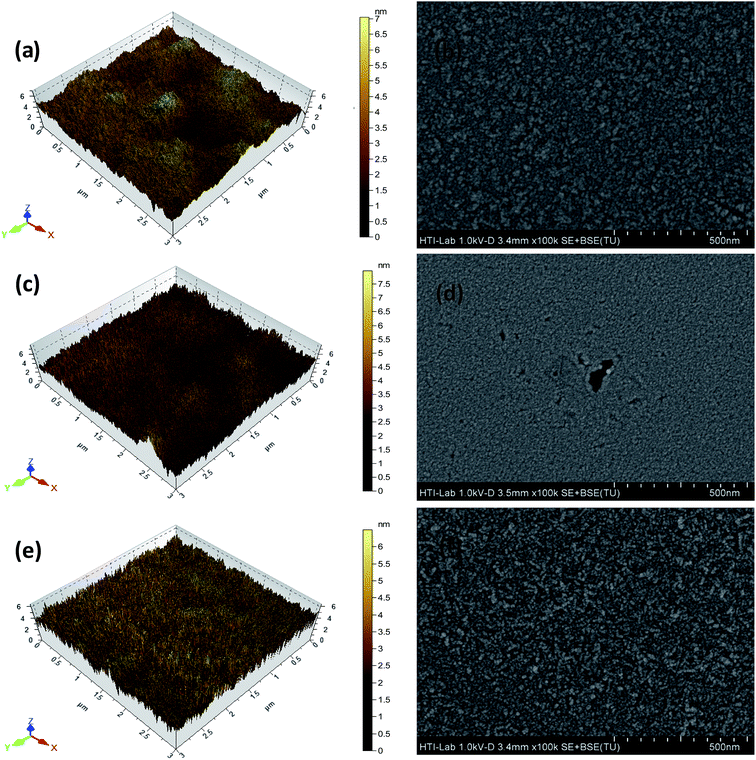 |
| Fig. 3 AFM images of (a) VOPcPhO@25, (c) Alq3@125, (e)V1 : A2@75, and FESEM (b) VOPcPhO@25, (d) Alq3@125, and (f) V1 : A2@75. | |
Table 1 Summary of the properties of thin film sensors
S. no |
Thin film |
Annealing temperature (°C) |
Sensitivity SSC (pF/%) |
Sensitivity SSR (MΩ/%) |
τres (s) |
τrec (s) |
Surface roughness (nm) |
1 |
VOPcPhO |
25 |
1.06 |
9.68 |
13 |
8 |
0.83 |
2 |
Alq3 |
125 |
0.3125 |
12.81 |
6 |
4 |
0.57 |
3 |
V1 : A2 |
75 |
0.325 |
14.5 |
4 |
3 |
0.937 |
The chemical bonding structures of Alq3, VOPcPhO, and their blend thin films were investigated by FTIR and the results are shown in Fig. 4. In line (a), C
C aromatic stretching vibrations are observed in the range of 1601–1497 cm−1. The absorption bands at 1385 cm−1 to 1114 cm−1 represent the resonance of aromatic amine (C–N–C).28 The band at 918 cm−1 is due to an Al–N stretching vibration. Moreover, out-of-plane quinoline CH wagging vibration can be seen at 744 cm−1. The weaker band at 555 cm−1 originates from the stretching vibration of Al–O.29 Line b in Fig. 4 represents the characteristic spectrum of VOPcPhO. The peak at 1057 cm−1 shows the presence of a single V–O stretching motion which was already reported by Miller and Cousins.30 This peak has been assigned to shorter V–O bonds. However, the peak at 895 cm−1 also shows the presence of V–O bonds but this band is due to the characteristic of longer V–O bonds.31 Moreover, the band at 746 cm−1 corresponds to out-of-plane C–H bending modes and this peak is a characteristic of almost all phthalocyanines.32 The FTIR of the blend of Alq3 and VOPcPhO is presented in Fig. 4 (line c).
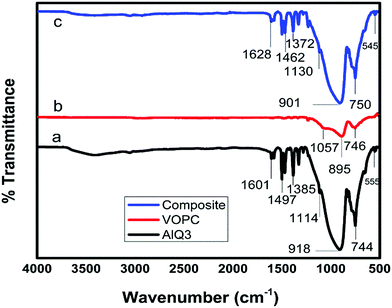 |
| Fig. 4 FTIR spectra of (a) Alq3, (b) VOPcPhO, and (c) composite thin film of Alq3 and VOPcPhO. | |
Response times τres and recovery times τrec for Alq3, VOPcPhO, and their composite (V1
:
A2) are given in Fig. 5a–c; they are 6 s, 13 s, and 4 s and 4 s, 8 s, and 3 s, respectively. The response and recovery time of the composite is better than others. The response time and recovery time of the sensors were measured as follows: initially the sensors were placed in a sealed chamber with 20% RH and then they were exposed to an environment with 70% RH level, thereby enabling them to adsorb water vapor and hence increasing their capacitance. The recovery time of a sensor was achieved by first exposing it to a humidity level of 70% RH and then immediately back to 20% RH. Fig. 5d–f show the hysteresis of Alq3, VOPcPhO, and their composites at the ratio V1
:
A2 at 100 Hz frequency. The up and down arrows show the increase and decrease in % RH, respectively.
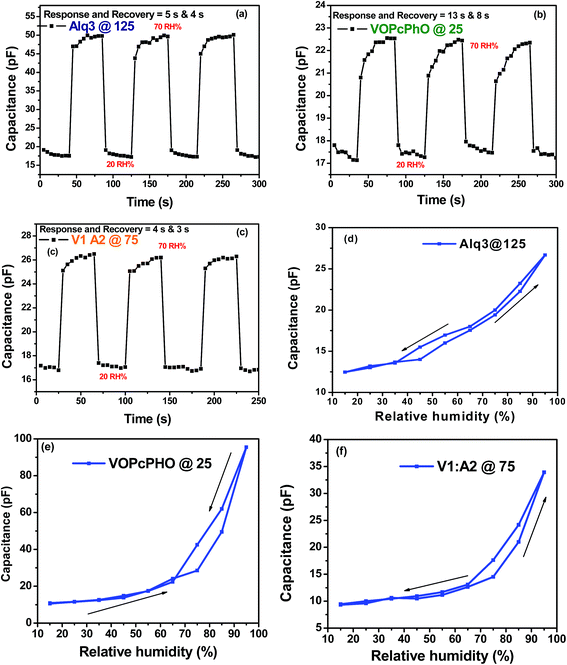 |
| Fig. 5 Transient response of capacitance to time of (a) VOPcPhO@room temperature, (b) Alq3@125 °C, and (c) VOPcPhO : Alq3 (1 : 2)@75 °C. Hysteresis behavior of capacitance–RH of (d) Alq3, (e) VOPcPhO and (f) composite (VOPcPhO : Alq3) at room temperature. | |
Conclusion
In this work, the effect of a humid environment on a surface type humidity sensor based on VOPcPhO, Alq3, and their composite thin film prepared by spin coating was investigated. Compared to VOPcPhO and Alq3 stand-alone sensors, the VOPcPhO
:
Alq3 composite-based sensor demonstrated superior performance with significantly improved sensing parameters, highlighting unique advantages of the low-molecular composite-based thin film organic humidity sensor. This research highlights the potential of a low-molecular weight complex to develop a cheap and reliable sensing element for assessing humidity in the surrounding environment. Our composite sensor exhibits a superior performance as compared to stand-alone films.
Acknowledgements
The authors are thankful to Capital University of Science and Technology Islamabad, University of Malaya, Malaysia, Ghulam Ishaq Khan Institute of Engineering Sciences and Technology and Center for Advanced Materials (CAM), Qatar University for their exclusive support during this research work.
References
- Z. M. Rittersma, Sens. Actuators, A, 2002, 96, 196 CrossRef CAS.
- F. Gutman and L. E. Lyons, Organic semiconductors, Part A, ed. E. Robert, Krieger Publishing Company, Malabar, Florida, 1981 Search PubMed.
- F. Gutman, H. Keyzer and L. E. Lyons, Organic semiconductors, Part B, ed. E. Robert, Krieger Publishing Company, Malabar, Florida, 1983 Search PubMed.
- M. I. Azmer, Q. Zafar, Z. Ahmad and K. Sulaiman, RSC Adv., 2016, 6, 35387–35393 RSC.
- K.-J. Park and M.-S. Gong, Sens. Actuators, B, 2017, 246, 53–60 CrossRef CAS.
- M. Eryürek, Z. Tasdemir, Y. Karadag, S. Anand, N. Kilinc, B. Alaca and A. Kiraz, Sens. Actuators, B, 2016, 1115–1120 Search PubMed.
- Z. Zhuang, Y. Li, D. Qi, C. Zhao and H. Na, Sens. Actuators, B, 2017, 242, 801–809 CrossRef CAS.
- C. Kelb, M. Körner, O. Prucker, J. Rühe, E. Reithmeier and B. Roth, Procedia Technology, 2016, 26, 530–536 CrossRef.
- D. Toloman, A. Popa, M. Stan, C. Socaci, A. Biris, G. Katona, F. Tudorache, I. Petrila and F. Iacomi, Appl. Surf. Sci., 2017, 410–417 CrossRef CAS.
- H. Zhao, T. Zhang, R. Qi, J. Dai, S. Liu, T. Fei and G. Lu, Sens. Actuators, B, 2016 DOI:10.1016/j.snb.2016.11.104.
- M. T. S. Chani, K. S. Karimov, F. A. Khalid, K. Raza, M. U. Farooq and Q. Zafar, Phys. E, 2012, 45, 77–81 CrossRef CAS.
- Y. Sakai, Y. Sadaoka and M. Matsuguchi, Sens. Actuators, B, 1996, 35–36, 85–90 CrossRef.
- K. S. Karimov, I. Q. Khan, T. A. Khan and P. H. Draper, Environ. Monit. Assess., 2008, 141, 323–328 CrossRef CAS PubMed.
- Z. Ahmad, M. H. Sayyad, M. Yaseen, K. C. Aw, M. M. Tahir and M. Ali, Sens. Actuators, B, 2011, 155, 81–85 CrossRef CAS.
- Y. Pan, X. Liao, Y. Wu, L. Chen, Y. Zhao, Y. Shen, F. Li, S. Shen and D. Huang, Thin Solid Films, 1998, 324, 209–213 CrossRef.
- H. B. Wang, D. Song, J. L. Yang, B. Yu, Y. H. Geng and D. H. Yan, Appl. Phys. Lett., 2007, 90, 253510–253512 CrossRef.
- M. I. Azmer, Z. Ahmad, K. Sulaiman, F. Touati, T. M. Bawazeer and M. S. Alsoufi, Appl. Surf. Sci., 2017, 399, 426–431 CrossRef CAS.
- H. A. Malik, F. Aziz, M. Asif, E. Raza, M. A. Najeeb, Z. Ahmad, W. Swelm, Q. Zafar, F. Touati and A. H. Kamboh, J. Lumin., 2016, 180, 209–213 CrossRef CAS.
- M. I. Azmer, Z. Ahmad, K. Sulaiman and F. Touati, Mater. Lett., 2016, 164, 605–608 CrossRef.
- M. I. Azmer, Q. Zafar, Z. Ahmad, K. Sulaiman and K. S. Karimov, Synth. Met., 2014, 191, 120–125 CrossRef CAS.
- F. Aziz, M. H. Sayyad, K. Sulaiman, B. Majlis, K. S. Karimov, Z. Ahmad and G. Sugandi, Meas. Sci. Technol., 2011, 23, 014001 CrossRef.
- Z. Ahmad, M. H. Suhail, I. I. Muhammad, W. K. Al-Rawi, K. Sulaiman, Q. Zafar, A. S. Hamzah and Z. Shaameri, Chin. Phys. B, 2013, 22, 100701 CrossRef.
- J. Shinar, Organic Light-Emitting Devices, Springer-Verlag, NY, 2004 Search PubMed.
- C. Zhang, P. Zhang, X. Xu, Y. Dang, X. Chen and B. Kang, Mater. Lett., 2016, 164, 591–594 CrossRef CAS.
- K. Barua, Introduction to condensed matter physics, Alpha Science International Limited, 2007 Search PubMed.
- Z. Chen and C. Lu, Sens. Lett., 2005, 3, 274–295 CrossRef CAS.
- A. Croft, R. Davison and M. Hargreaves, Engineering Mathematics: A Modern Foundation for Electrical, Electronic, and Control Engineers, Addison-Wesley Longman Publishing Co., Inc., 1992 Search PubMed.
- S. Kim, S. Ryu, J. Choi, S. Kang, S. Park, S. Im, C. Whang and D. Choi, Thin Solid Films, 2001, 398, 78–81 CrossRef.
- Y. Sakurai, Y. Hosoi, H. Ishii, Y. Ouchi, G. Salvan, A. Kobitski, T. Kampen, D. Zahn and K. Seki, J. Appl. Phys., 2004, 96, 5534–5542 CrossRef CAS.
- F. A. Miller and L. R. Cousins, J. Chem. Phys., 1957, 26, 329–331 CrossRef CAS.
- L. D. Frederickson Jr and D. Hausen, Anal. Chem., 1963, 35, 818–827 CrossRef.
- V. Kolotovska, M. Friedrich, D. Zahn and G. Salvan, J. Cryst. Growth, 2006, 291, 166–174 CrossRef CAS.
Footnote |
† Electronic supplementary information (ESI) available. See DOI: 10.1039/c7ra02525d |
|
This journal is © The Royal Society of Chemistry 2017 |
Click here to see how this site uses Cookies. View our privacy policy here.