DOI:
10.1039/C7RA02238G
(Paper)
RSC Adv., 2017,
7, 26921-26929
Synthesis and evaluation of novel 12-aryl berberine analogues with hypoxia-inducible factor-1 inhibitory activity†
Received
23rd February 2017
, Accepted 9th May 2017
First published on 23rd May 2017
Abstract
Eighteen novel 12-aryl berberine derivatives were synthesized and evaluated for their inhibitory effects on hypoxia-inducible factor (HIF-) 1 transcription which is a potential target for the development of anticancer agents. As a result, seven 12-phenyl berberine analogues (3a–3f, 3k) showed more potent inhibitory effect on hypoxia-induced HIF-1 transcriptional activity than the parent compound berberine (1). Notably, the 12-biphenyl berberine (3e) exhibited the strongest HIF-1 suppressing activity among all the berberine analogues with an IC50 value of 0.74 μM. The introduction of a biphenyl substituent to berberine resulted in a 5.4 fold enhancement of potency over the parent berberine (1). Structure–activity relationship analysis revealed that the phenyl substituent is a preferential pharmacophore for HIF-1 inhibitory activity of 12-aryl berberine analogues over heteroaromatic ring substituents of pyridyl, thienyl and furyl. The phenyls containing hydrophobic moieties are beneficial to increase the HIF-1 suppressing effect compared to those bearing hydrophilic ones. In addition, the p-fluoro phenyl is also a favorable pharmacophore over the m- and o-fluoro phenyls. Western blot assay revealed that berberine analogues (3a–3f, 3k) exhibited a stronger suppressive effect on hypoxia-induced expression of HIF-1α protein than berberine in both T47D and MCF-7 cells. It was also found that berberine derivatives (3a–3f, 3k) possessing more potent HIF-1 inhibitory activity generally showed a greater toxic effect on MCF-7 cancer cells. These findings may guide the rational design of berberine-based HIF-1 inhibitors for the development of anticancer drugs. This is also the first report on berberine and its derivatives' inhibitory effect on HIF-1 transcriptional activity.
Introduction
Hypoxia of solid tumors originates from the aberrant cell proliferation rate from an imbalance between oxygen supply and consumption.1,2 Hypoxia-inducible factor 1 (HIF-1) is a principal transcription factor by which tumor cells adapt to the low oxygen microenvironment. HIF-1 plays an essential role in expression of genes involving angiogenesis, cell proliferation, survival, invasion and metastasis of solid tumors and energy metabolism.3 HIF-1 is a heterodimer composed of an oxygen-regulated subunit HIF-1α and a constitutively expressed subunit HIF-1β.4 HIF-1α protein is rapidly degraded under normal conditions but stabilized under hypoxia conditions. The stabilized HIF-1α is accumulated in cytosol and further translocated to the nucleus where it dimerizes with HIF-1β and then binds to hypoxia response elements (HREs) together with coactivators to activate target genes, including vascular endothelial growth factor (VEGF), glucose transporter-1 (GLUT1), hexokinases etc. HIF-1α is detected at increased levels in many types of human tumors, and overexpression of HIF-1α correlates with poor prognosis and resistance to treatment for cancer patients.5 Therefore, HIF-1 has been regarded as a potential target for the development of anticancer agents.6
Natural products play an important role in drug discovery. A large number of inhibitors of HIF-1 activation have been identified from medicinal plants and microorganisms,7–15 such as cardiac glycosides,10,11 geldanamycin,12 chaetocin,13 mannasantin14 etc. Berberine (1), a major bioactive isoquinoline alkaloid from traditional Chinese medicines including Rhizoma Coptidis (“Huang-Lian” in Chinese) and Cortex Phellodendri (“Huang-Bai” in Chinese), was recently discovered to inhibit HIF-1α protein expression in various carcinoma cells and enhance radiosensitivity of cancer cells.16–19 As a hotspot compound, berberine shows a wide spectrum of pharmacological effects20–27 including antihyperglycemic,21 hypolipidemic,22,23 anticancer,24 anti-inflammatory,25 neuroprotective26 and antimicrobial27 activities. This alkaloid was used as an OTC drug for the treatment of diarrhea in China for decades. As an efficacious and safe drug, berberine becomes a fascinating leading compound for structural modification for the development of new drug. Numerous novel berberine derivatives have been designed, synthesized and screened for medicinal application.28–37 However these derivatives mainly focus on positions of C-8,36 C-9
29–34 and C-13
35 of berberine by appending various types of substituents including aliphatic chains and heterocycles. There was few reports on aryl directly substituted berberine analogues or derivation of berberine at C-12 position. So far, only bromination has been performed at C-12 position of berberine.38,39 Therefore, it's desirable to study the aryl substituted effects at the C-12 position of berberine. To promote our study on berberine,40–45 a series of novel 12-aryl berberine analogues were designed, synthesized and evaluated for their inhibitory effect on HIF-1 transcriptional activity by using a T47D cell-based co-transfected firefly and Renilla luciferase reporter assay. Inhibition of HIF-1α expression by active berberine analogues was subsequently assessed by western blot assay in both T47D and MCF-7 cells under hypoxic condition. The cytotoxic effects of active berberine analogues were also evaluated on MCF-7 breast cancer cells.
Results and discussion
Chemistry
A series of 12-aryl berberine derivatives were prepared via Suzuki cross coupling reaction of 12-bromoberberine (2) with various arylboronic acids or heteroarylboronic acids (Scheme 1). 12-Bromoberberine (2) was firstly synthesized by bromination of berberine chloride with elemental bromine in dioxane according to the published method.38 Subsequently, 12-bromoberberine (2) was reacted with various arylboronic acids under the catalysis of palladium at room temperature to produce target 12-aryl berberine derivatives (3a–3r) by using Suzuki cross coupling reaction. A wide variety of arylboronic acids, from the electron-rich to electron-deficient nature, were successfully applied to the coupling reaction to afford four groups of 12-aryl berberine analogues, including hydrophobic group bearing phenyl berberines (3b–3f), hydrophilic group bearing phenyl berberines (3g–3j), fluoro substituted phenyl berberines (3k–3n) and heteroaromatic ring substituted berberines (3o–3r).
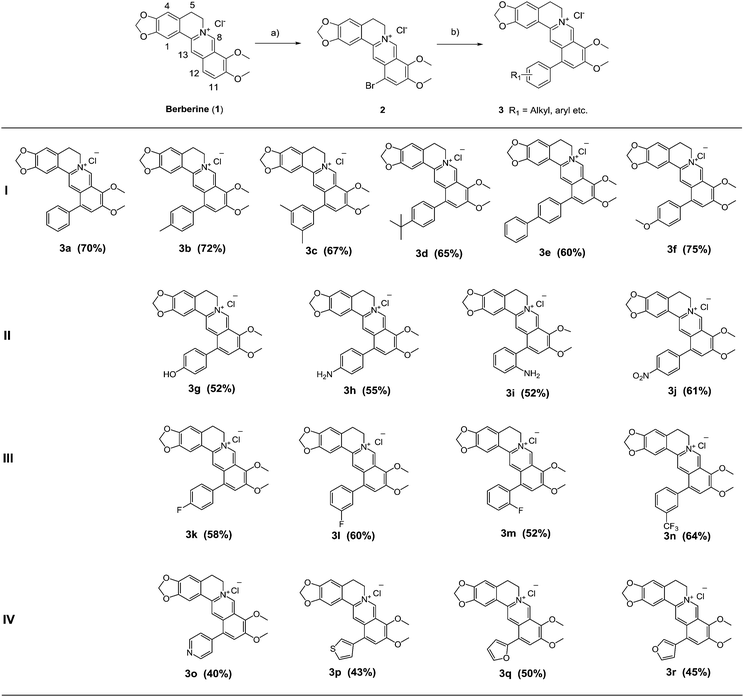 |
| Scheme 1 Synthesis of 12-aryl berberine analogues. Reagents and conditions: (a) Br2/dioxane; (b) RB(OH)2/Pd(OAc)2/Na2CO3/EtOH. (I) Hydrophobic group bearing phenyl berberines; (II) hydrophilic group bearing phenyl berberines; (III) fluoro substituted phenyl berberines; (IV) heteroaromatic ring substituted berberines. All are isolated yields. | |
Biological activity
Compounds that inhibit HIF-1 transcriptional activity show great potential in anticancer therapy since HIF-1 plays an important role in many key aspects of cancer biology. A T47D (human ductal breast epithelial tumor) cell-based reporter assay was utilized to evaluate the inhibitory effects of 12-aryl berberine analogues (3a–3r), 2 and berberine on HIF-1 transcriptional activity. Briefly, T47D cells were transiently co-transfected with the hypoxia response element (HRE) luciferase and Renilla plasmids which contain reporter genes for hypoxia-inducible expression of firefly luciferase and constitutive expression of Renilla luciferase, respectively.10,11 The HRE contains essential binding sites for HIF-1, which mediates increased transcription in cells that are exposed to hypoxia. We could screen for compounds that specifically inhibit hypoxia-induced firefly luciferase activity driven by HIF-1 from the decreased ratio of firefly/Renilla luciferase activity under normoxia and hypoxia conditions. Digoxin, a well-studied HIF-1 inhibitor,10 was used as a positive control. The HIF-1 inhibitory effects of all berberine derivatives were firstly evaluated at a single concentration of 10 μM. As illustrated in Fig. 1, the relative HIF-1 luciferase activity of the transfected T47D cells cultured under normoxic conditions (20% O2) and under hypoxic conditions (2% O2) for 20 h were 1.8% and 100%, respectively, displaying the ratio of firely/Renilla luciferase activity in hypoxic condition was 55.5-fold (100/1.8) higher than that in normoxic condition. Digoxin decreased HIF-1 luciferase activity from 100% in the blank control group to 57.0% under hypoxic condition (2% O2) at the drug concentration of 100 nM, showing its suppressive effect on hypoxia-induced HIF-1 transcriptional activity. In the same way, berberine also significantly (p < 0.001) reduced the hypoxia-induced HIF-1 transcriptional activity to 20.2% (Fig. 1) at the tested concentration of 10 μM. Similarly, fifteen berberine derivatives (2, 3a–3i, 3k–3n, 3p) also showed inhibitory effects on hypoxia-induced HIF-1 transcriptional activity in various potency (Fig. 1). Compounds 3a–3f and 3k showed stronger HIF-1 inhibitory effects than their parent compound, berberine. The analogues 3h, 3m and 3n displayed a comparable HIF-1 suppressing activity to berberine, while 3g, 3j, 3l, 3p and 2 possessed weaker HIF-1 inhibiting activity than the parent berberine. However, compounds 3j, 3o, 3q and 3r didn't demonstrate HIF-1 inhibitory activity at the tested concentration.
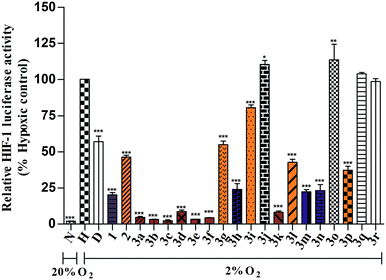 |
| Fig. 1 Relative HIF-1 luciferase activity (% hypoxic control) of berberine and its derivatives (10 μM). N means normoxic condition (20% O2), H represents hypoxia condition (2% O2), D stands for digoxin (100 nM). ***p < 0.001, **p < 0.01 or *p < 0.05 for comparison between berberine analogue-treated group and the blank control group. | |
Based on the above screening result, berberine and nine active 12-aryl berberine derivatives (3a–3f, 3k–3m) were selected for further evaluation of their dose-dependent response for suppressing hypoxia-induced HIF-1 transcriptional activity. As a result (Table 1), all tested berberine derivatives exhibited inhibitory effect on hypoxia-induced HIF-1 transcriptional activity with IC50 values ranged from 0.74 to 11.82 μM, and berberine showed an IC50 value of 4.78 μM. The analogues 3a–3f, 3k showed a 2.2–6.4 fold more potent inhibitory effects on HIF-1 transcriptional activity than the parent berberine, 3m exhibited comparable potency to berberine, while 3l still displayed weaker HIF-1 suppressing activity than berberine. The cell viability, as measured by the control Renilla luciferase activity, was more than 85% in the groups treated by berberine derivatives at the concentration around their IC50 values (Table S1†), indicating that all tested compounds effectively inhibited HIF-1 transcription without significant cytotoxicity at the concentrations at which they were active.46
Table 1 HIF-1 inhibitory activity in T47D cell and anti-proliferative effect on MCF-7 cell of active berberine analoguesa
Compounds |
HIF-1 inhibitory activity (IC50, μM) |
Cytotoxicity on MCF-7 cell (IC50, μM) |
***p < 0.001 for comparison between groups treated by compounds and group treated by 1. |
1 |
4.78 ± 0.73 |
26.57 ± 1.75 |
3a |
1.51 ± 0.09*** |
5.58 ± 2.10*** |
3b |
1.39 ± 0.07*** |
4.51 ± 0.84*** |
3c |
1.26 ± 0.29*** |
2.74 ± 0.06*** |
3d |
2.12 ± 0.36*** |
3.59 ± 0.69*** |
3e |
0.74 ± 0.06*** |
0.98 ± 0.07*** |
3f |
1.35 ± 0.14*** |
9.17 ± 2.59*** |
3k |
2.07 ± 0.17*** |
8.45 ± 0.22*** |
3l |
11.82 ± 1.65*** |
20.97 ± 5.28 |
3m |
5.40 ± 1.36 |
17.05 ± 4.03*** |
The structure–activity relationship (SAR) of these synthesized 12-aryl berberine analogues was analyzed and summarized as follows on the basis of the above results. Firstly, a phenyl substituent at C-12 of berberine contributes to the inhibitory effect on HIF-1 transcriptional activity, compared to heteroaromatic rings including pyridyl, thienyl and furyl substituents at C-12 of berberine. As illustrated in Fig. 2A, 3a containing a 12-phenyl substituent remarkably suppressed HIF-1 transcriptional activity with an IC50 value of 1.51 μM. In sharp contrast, 3o, 3q and 3r with 12-heteroaromatic ring substituents did not show any suppressing effect on HIF-1 function at the tested concentration of 10 μM and the inhibitory effect of 3p containing thienyl ring was much weaker than that of 3a. Thus, the phenyl substituent is a preferential pharmacophore over the substituents of pyridyl, thienyl and furyl for the HIF-1 inhibiting activity of 12-aryl berberine analogues. Secondly, 12-phenyls containing hydrophobic groups are beneficial to enhance HIF-1 suppressing effect, compared to those with hydrophilic ones (Fig. 2B). The analogues 3b–3f which respectively bear hydrophobic p-methyl, 3,5-dimethyl, p-methoxyl, p-phenyl and p-tert-butyl moieties on their 12-phenyl substituent, exhibited inhibitory effect on HIF-1 transcriptional activity with IC50 values ranged from 0.74 to 2.12 μM. On the contrary, 3g, 3i and 3j with hydrophilic p-hydroxyl, o-amino groups, and p-nitryl on their 12-phenyl substituent did not display inhibition of HIF-1 function at the tested concentration of 10 μM. Notably, 12-biphenyl substituted berberine (3e) demonstrated the most potent HIF-1 inhibitory effect among all 12-phenyl berberine analogues with an IC50 value of 0.74 μM. The introduction of the biphenyl substituent to berberine leads to an increase of a 5.5 fold potency over that of the parent berberine. Finally, the p-fluoro phenyl berberine (3k) showed stronger inhibitory effect on HIF-1 transcriptional activity than those with m- and o-fluoro phenyl berberines 3l and 3m, respectively (Fig. 2C). The IC50 value of 3k was 2.07 μM, which is a 5.7 and 2.6 fold more potent than that of 3l and 3m, respectively. Collectively, the phenyls with hydrophobic and p-fluoro groups are favorable pharmacophores for enhancing HIF-1 inhibitory activity of 12-aryl berberine derivatives. Additionally, we also performed a calculation of log
P (clog
P) using ChemDraw Ultra 12.0 to determine lipophilicity of berberine and selected derivatives (3a–3c, 3e and 3f).47,48 A modest inverse linear correlation of log IC50 values and clog
P was evident (r2 = 0.9133), suggesting that hydrophobicity of these 12-aryl berberine derivatives plays a significant role in their HIF-1 inhibitory activity (Fig. S1†).
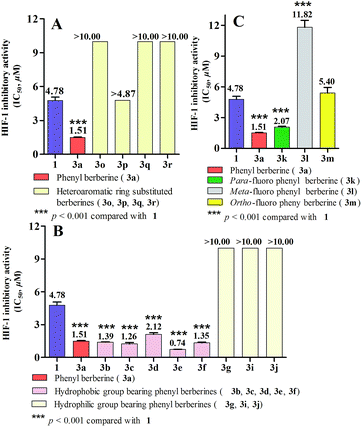 |
| Fig. 2 HIF-1 inhibitory activity (IC50) of 12-aryl berberine derivatives. (A) Comparison of effect of phenyl and heteroaromatic rings substituents on HIF-1 function; (B) comparison of effect of hydrophobic and hydrophilic groups of phenyl berberines on HIF-1 function; (C) comparison of effect of fluoro substituted position of phenyl berberines on HIF-1 function. | |
The inhibitory effects of berberine and its analogues 3a–3f, 3k on the expression of HIF-1α protein were also evaluated in both T47D and MCF-7 cells under hypoxic condition by using western blot analysis. As the results, 3a–3f and 3k showed more potent suppressive activities on hypoxia-induced expression of HIF-1α protein than the parent berberine at a single concentration of 10 μM (Fig. 3A). Dose-dependent responses were also observed for berberine and 3e that exposure of T47D and MCF-7 cells to berberine (2.0–50.0 μM, Fig. 3B) and 3e (0.5–10.0 μM, Fig. 3C) for 24 h inhibited hypoxia-induced expression of HIF-1α protein. Obviously, 3e exhibited much stronger inhibitory effect on the expression of HIF-1α protein than berberine. All these results coincided with those of inhibitory effects on hypoxia-induced HIF-1 transcriptional activity.
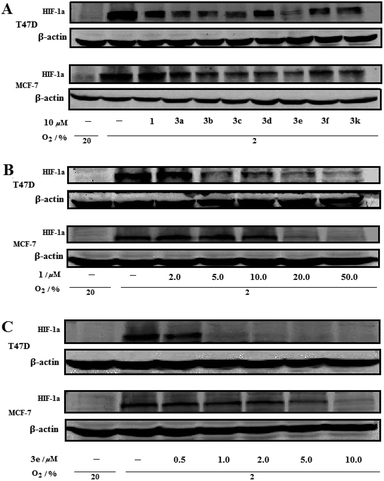 |
| Fig. 3 Inhibition of hypoxia-induced expression of HIF-1α protein in T47D and MCF-7 cells by berberine (1) and 12-aryl berberine derivatives. (A) T47D and MCF-7 cells were exposed to vehicle, 10 μM of berberine (1), 3a–3f and 3k for 24 h under hypoxic (2% O2) or normoxic conditions (20% O2) and cell lysates were subjected to immunoblot assays for HIF-1α and β-actin; (B) T47D and MCF-7 cells were exposed to vehicle or the indicated concentration of berberine (1) for 24 h under hypoxic (2% O2) or normoxic conditions (20% O2); (C) T47D and MCF-7 cells were exposed to vehicle or the indicated concentration of berberine analogue 3e for 24 h under hypoxic (2% O2) or normoxic conditions (20% O2). | |
The cytotoxic effects of compounds 3a–3f, 3k–3m and berberine were evaluated on human breast cancer cell (MCF-7). As the result (Table 1), berberine exhibited cytotoxicity against MCF-7 cell with an IC50 value of 26.57 μM. Compound 3e, the most potent HIF-1 inhibitor, showed the strongest cytotoxic effect among all tested compounds with an IC50 value of 0.98 μM, which was a 27.1 fold potency of that of berberine. Compounds 3a–3d, 3f and 3k with stronger HIF-1 inhibitory activity significantly (p < 0.001) inhibited MCF-7 cell growth with IC50 values ranged from 2.74 to 9.17 μM, which were a 2.8–9.6 fold more toxic than that of berberine. However, 3l and 3m with weaker HIF-1 suppressing activity exhibited higher IC50 value (20.97 and 17.05 μM, respectively) than the other tested berberine analogues.
Conclusions
Eighteen novel derivatives of 12-aryl substituted berberine were successfully synthesized by Suzuki cross coupling reaction and evaluated for their inhibitory effect on HIF-1 transcriptional activity. Seven berberine analogues (3a–3f, 3k) showed more potent (p < 0.001) HIF-1 suppressing effects than the parent berberine (1). Analysis of SAR suggested that phenyls containing hydrophobic moieties are preferential pharmacophores for the HIF-1 inhibitory activity of 12-aryl berberine analogues. Among all the berberine analogues, the 12-biphenyl berberine (3e) exhibited the most potent HIF-1 suppressing activity with a 5.4 fold enhancement of potency over the parent berberine. Berberine analogues 3a–3f and 3k demonstrated more potent inhibitory effects on hypoxia-induced expression of HIF-1α protein in both T47D and MCF-7 cells than the parent berberine. In general, berberine analogues (3a–3f, 3k) with more potent HIF-1 inhibitory activity also exhibited more toxic effect on MCF-7 cancer cells. This study provided a series of promising berberine-based HIF-1 inhibitors. Additionally, it was the first report on berberine and its derivatives' inhibitory activity on HIF-1 transcriptional activity.
Methods
General
The 1H, 13C NMR experiments were measured on a Bruker Ascend® 600 NMR spectrometer (600 MHz for 1H and 150 MHz for 13C) with the solvent signal as internal reference. High resolution mass spectra (HRMS) were performed on an Agilent 6230 electrospray ionization (ESI) time-of-flight (TOF) mass spectrometer. UHPLC-DAD analyses were carried out on an Agilent 1290 Infinity LC system using an ACQUITY UPLC® BEH C18 column (1.7 mm, 100 × 2.1 mm, Waters®), and all synthetic compounds were more than 95% in purity. Melting points are uncorrected and were measured on a MPA100 Optimelt Point Apparatus. Column chromatography was performed with Davisil silica gel (particle size 40–63 micron). Analytical thin layer chromatography (TLC) was performed on Merck silica gel 60-F254 plates. Berberine hydrochloride and aromatic boronic acids were purchased from Acros. All other chemicals were purchased from 9dingchem. Unless otherwise specified, all fine chemicals were used as received.
Synthesis of 12-bromoberberine chloride (2)
Berberine chloride (0.37 g, 1.0 mmol) was suspended in dioxane (20 mL). A solution of Br2 (0.5 mL, 9.7 mmol) in dioxane (10 mL) was added into the above berberine solution dropwise at room temperature. The reaction mixture was then stirred at room temperature for 4 days. The precipitate was filtered off and successively washed with 10% Na2S2O3 solution (20 mL × 2), 10% NaHCO3 solution (20 mL × 2), and 20% KBr solution (20 mL × 2). Then, the precipitate was completely dissolved in AgCl hot methanol solution. After filtration of the above solution, the methanol solution was evaporated and the residue was purified by silica gel chromatography to afford 2 (0.31 g, 69% yield). Its HRMS, 1H-, 13C-NMR and melt point data are in good agreement with those of 12-bromoberberine chloride in the literature.38
General procedure for Suzuki cross coupling reaction
A mixture of 12-bromoberberine (2, 10.0 mg, 0.022 mmol), boronic acid (1.5 equiv.), palladium acetate (0.45 mg, 0.002 mmol) and sodium carbonate (4.7 mg, 0.044 mmol) was stirred in anhydrous ethanol (2 mL) at room temperature overnight. The reaction mixture was quenched with water and extracted with dichloromethane. The dichloromethane extract was washed with brine, and then dried over MgSO4. Then, the solvent was evaporated and the residue was purified by chromatography on silica gel to give yellowish solid of berberine derivatives. Finally, the yellowish solid was converted into chloride derivatives (3a–3r) of berberine either by AgCl in hot methanol or using column chromatography of ion-exchange Amberlite® IRA-400 (chloride form).
9,10-Dimethoxy-12-phenyl-5,6-dihydro-[1,3]dioxolo[4,5-g]isoquinolino[3,2-a]isoquinolin-7-ium chloride (3a). Purification by silica gel column chromatography (95
:
5 CHCl3/MeOH) afforded 6.9 mg (70%) of 3a as yellow solid. TLC Rf = 0.28 (95
:
5 CHCl3/MeOH); mp 206–208 °C; 1H NMR (600 MHz, CDCl3): δ 10.61 (s, 1H), 8.11 (s, 1H), 7.73 (s, 1H), 7.58–7.61 (m, 3H), 7.46–7.48 (m, 2H), 7.01 (s, 1H), 6.81 (s, 1H), 6.05 (s, 2H), 5.33 (t, J = 6.0 Hz, 2H), 4.42 (s, 3H), 4.08 (s, 3H), 3.32 (t, J = 6.0 Hz, 2H); 13C NMR (150 MHz, CDCl3): δ 150.6, 150.3, 148.2, 147.7, 145.4, 137.8, 136.9, 135.9, 131.5, 130.8, 129.72, 129.3, 129.0, 126.5, 122.6, 120.4, 117.7, 108.8, 105.0, 102.1, 63.4, 57.1, 56.0, 27.8. HRMS (ESI): m/z for C26H22NO4 [M − Cl]+ calcd 412.1543, found 412.1569.
9,10-Dimethoxy-12-(p-tolyl)-5,6-dihydro-[1,3]dioxolo[4,5-g]isoquinolino[3,2-a]isoquinolin-7-ium chloride (3b). Purification by silica gel column chromatography (95
:
5 CHCl3/MeOH) afforded 7.3 mg (72%) of 3b as yellow solid. TLC Rf = 0.28 (95
:
5 CHCl3/MeOH); mp 205–207 °C; 1H NMR (600 MHz, CDCl3): δ 10.57 (s, 1H), 8.13 (s, 1H), 7.71 (s, 1H), 7.40 (d, J = 7.8 Hz, 2H), 7.35 (d, J = 7.8 Hz, 2H), 7.27 (s, 1H), 7.03 (s, 1H), 6.06 (s, 2H), 5.33 (t, J = 6.0 Hz, 2H), 4.39 (s, 3H), 4.08 (s, 3H), 3.32 (t, J = 6.0 Hz, 2H), 2.52 (s, 3H); 13C NMR (150 MHz, CDCl3): δ 150.5, 150.3, 148.2, 147.5, 145.2, 139.0, 137.7, 136.0, 133.9, 131.5, 130.8, 130.0, 129.6, 126.4, 122.6, 120.4, 117.9, 108.8, 105.0, 102.1, 63.3, 57.1, 56.0, 27.6, 21.3. HRMS (ESI): m/z for C27H24NO4 [M − Cl]+ calcd 426.1700, found 426.1726.
12-(3,5-Dimethylphenyl)-9,10-dimethoxy-5,6-dihydro-[1,3]dioxolo[4,5-g]isoquinolino[3,2-a]isoquinolin-7-ium chloride (3c). Purification by silica gel column chromatography (95
:
5 CHCl3/MeOH) afforded 7.0 mg (67%) of 3c as yellow solid. TLC Rf = 0.28 (95
:
5 CHCl3/MeOH); mp 203–204 °C; 1H NMR (600 MHz, CDCl3): δ 10.58 (s, 1H), 8.11 (s, 1H), 7.70 (s, 1H), 7.20 (s, 1H), 7.05 (s, 2H), 7.02 (s, 1H), 6.82 (s, 1H), 6.05 (s, 2H), 5.33 (t, J = 6.0 Hz, 2H), 4.41 (s, 3H), 4.08 (s, 3H), 3.32 (t, J = 6.0 Hz, 2H), 2.45 (s, 6H); 13C NMR (150 MHz, CDCl3): δ 150.5, 150.3, 148.2, 147.5, 145.2, 139.0, 137.6, 136.8, 136.3, 131.5, 130.8, 130.6, 127.5, 126.3, 122.5, 120.5, 117.9, 108.8, 105.0, 102.1, 63.3, 57.1, 56.0, 27.6, 21.4. HRMS (ESI): m/z for C28H26NO4 [M − Cl]+ calcd 440.1856, found 440.1875.
12-(4-(tert-Butyl)phenyl)-9,10-dimethoxy-5,6-dihydro-[1,3]dioxolo[4,5-g]isoquinolino[3,2-a]isoquinolin-7-ium chloride (3d). Purification by silica gel column chromatography (95
:
5 CHCl3/MeOH) afforded 5.3 mg (63%) of 3d as yellow solid. TLC Rf = 0.29 (95
:
5 CHCl3/MeOH); mp 207–209 °C; 1H NMR (600 MHz, CDCl3): δ 10.59 (s, 1H), 8.19 (s, 1H), 7.72 (s, 1H), 7.61 (d, J = 8.4 Hz, 2H), 7.41 (d, J = 8.4 Hz, 2H), 7.07 (s, 1H), 6.82 (s, 1H), 6.06 (s, 2H), 5.32 (t, J = 6.0 Hz, 2H), 4.40 (s, 3H), 4.07 (s, 3H), 3.31 (t, J = 6.0 Hz, 2H), 1.44 (s, 9H); 13C NMR (150 MHz, CDCl3): δ 152.2, 150.5, 150.3, 148.2, 147.6, 145.2, 137.7, 135.9, 134.0, 131.4, 130.9, 129.4, 126.6, 126.3, 122.6, 120.5, 117.9, 108.8, 105.1, 102.1, 63.3, 57.1, 56.0, 34.9, 31.4, 27.6. HRMS (ESI): m/z for C30H30NO4 [M − Cl]+ calcd 468.2169, found 468.2180.
12-([1,1′-Biphenyl]-4-yl)-9,10-dimethoxy-5,6-dihydro-[1,3]dioxolo[4,5-g]isoquinolino[3,2-a]isoquinolin-7-ium chloride (3e). Purification by silica gel column chromatography (95
:
5 CHCl3/MeOH) afforded 6.9 mg (60%) of 3e as yellow solid. TLC Rf = 0.27 (95
:
5 CHCl3/MeOH); mp 208–210 °C; 1H NMR (600 MHz, CDCl3): δ 10.64 (s, 1H), 8.19 (s, 1H), 7.83 (d, J = 7.8 Hz, 2H), 7.78 (s, 1H), 7.72 (d, J = 7.8 Hz, 2H), 7.52–7.56 (m, 4H), 7.43 (t, J = 7.8 Hz, 1H), 7.06 (s, 1H), 6.82 (s, 1H), 6.04 (s, 2H), 5.34 (t, J = 6.0 Hz, 2H), 4.41 (s, 3H), 4.10 (s, 3H), 3.31 (t, J = 6.0 Hz, 2H); 13C NMR (150 MHz, CDCl3): δ 150.6, 150.3, 148.3, 147.8, 145.5, 141.9, 140.0, 137.8, 135.7, 135.5, 131.4, 130.9, 130.2, 129.1, 128.0, 128.0, 127.2, 126.5, 122.7, 120.4, 117.7, 108.8, 105.0, 102.1, 63.3, 57.1, 55.9, 27.6. HRMS (ESI): m/z for C32H26NO4 [M − Cl]+ calcd 488.1856, found 488.1868.
9,10-Dimethoxy-12-(4-methoxyphenyl)-5,6-dihydro-[1,3]dioxolo[4,5-g]isoquinolino[3,2-a]isoquinolin-7-ium chloride (3f). Purification by silica gel column chromatography (95
:
5 CHCl3/MeOH) afforded 7.9 mg (75%) of 3f as yellow solid. TLC Rf = 0.27 (95
:
5 CHCl3/MeOH); mp 194–196 °C; 1H NMR (600 MHz, CDCl3): δ 10.58 (s, 1H), 8.12 (s, 1H), 7.70 (s, 1H), 7.38 (d, J = 8.4 Hz, 2H), 7.12 (d, J = 8.4 Hz, 2H), 7.04 (s, 1H), 6.82 (s, 1H), 6.06 (s, 2H), 5.32 (t, J = 6.0 Hz, 2H), 4.39 (s, 3H), 4.08 (s, 3H), 3.95 (s, 3H), 3.31 (t, J = 6.0 Hz, 2H); 13C NMR (150 MHz, CDCl3): δ 160.1, 150.6, 150.3, 148.2, 147.5, 145.1, 137.6, 135.8, 131.7, 130.9, 130.8, 129.0, 126.4, 122.6, 120.4, 117.9, 114.7, 108.8, 105.0, 102.1, 63.3, 57.9, 55.9, 55.5, 27.6. HRMS (ESI): m/z for C27H24NO5 [M − Cl]+ calcd 442.1649, found 442.1664.
12-(4-Hydroxyphenyl)-9,10-dimethoxy-5,6-dihydro-[1,3]dioxolo[4,5-g]isoquinolino[3,2-a]isoquinolin-7-ium chloride (3g). Purification by silica gel column chromatography (gradient elution from 95
:
5 to 90
:
10 CHCl3/MeOH) afforded 5.3 mg (52%) of 3g as yellow solid. TLC Rf = 0.24 (95
:
5 CHCl3/MeOH); mp 198–200 °C; 1H NMR (600 MHz, CDCl3): δ 10.19 (s, 1H), 8.05 (s, 1H), 7.59 (s, 1H), 7.05 (d, J = 8.4 Hz, 2H), 6.91 (d, J = 8.4 Hz, 2H), 6.88 (s, 1H), 6.80 (s, 1H), 6.04 (s, 2H), 5.17 (t, J = 6.0 Hz, 2H), 4.38 (s, 3H), 4.05 (s, 3H), 3.34 (t, J = 6.0 Hz, 2H); 13C NMR (150 MHz, CDCl3): δ 157.8, 150.6, 150.2, 148.3, 146.7, 144.4, 137.4, 136.2, 131.4, 130.5, 130.3, 127.4, 126.2, 122.5, 120.4, 118.2, 116.4, 108.6, 104.9, 102.1, 63.0, 57.1, 56.2, 27.5. HRMS (ESI): m/z for C26H22NO5 [M − Cl]+ calcd 428.1492, found 428.1528.
12-(4-Aminophenyl)-9,10-dimethoxy-5,6-dihydro-[1,3]dioxolo[4,5-g]isoquinolino[3,2-a]isoquinolin-7-ium chloride (3h). Purification by silica gel column chromatography (gradient elution from 95
:
5 to 90
:
10 CHCl3/MeOH) afforded 5.6 mg (55%) of 3h as yellow solid. TLC Rf = 0.21 (95
:
5 CHCl3/MeOH); mp 178–180 °C; 1H NMR (600 MHz, CDCl3): δ 10.52 (s, 1H), 8.19 (s, 1H), 7.68 (s, 1H), 7.24 (d, J = 8.4 Hz, 2H), 7.06 (s, 1H), 6.87 (d, J = 8.4 Hz, 2H), 6.81 (s, 1H), 6.06 (s, 2H), 5.31 (t, J = 6.0 Hz, 2H), 4.38 (s, 3H), 4.07 (s, 3H), 3.31 (t, J = 6.0 Hz, 2H); 13C NMR (150 MHz, CDCl3): δ 150.49, 150.42, 148.23, 147.26, 147.21, 144.67, 137.44, 136.43, 131.69, 130.76, 130.67, 126.47, 126.07, 122.64, 120.50, 118.13, 115.43, 108.73, 104.97, 102.08, 63.28, 57.03, 55.94, 27.60. HRMS (ESI): m/z for C26H23N2O4 [M − Cl]+ calcd 427.1652, found 427.1658.
12-(2-Aminophenyl)-9,10-dimethoxy-5,6-dihydro-[1,3]dioxolo[4,5-g]isoquinolino[3,2-a]isoquinolin-7-ium chloride (3i). Purification by silica gel column chromatography (gradient elution from 95
:
5 to 90
:
10 CHCl3/MeOH) afforded 5.3 mg (52%) of 3i as yellow solid. TLC Rf = 0.22 (95
:
5 CHCl3/MeOH); mp 182–184 °C; 1H NMR (600 MHz, CDCl3): δ 10.50 (s, 1H), 7.91 (s, 1H), 7.78 (s, 1H), 7.35–7.37 (m, 1H), 7.12 (dd, J = 7.8, 1.2 Hz, 1H), 7.06 (s, 1H), 6.93–6.95 (m, 1H), 6.90 (dd, J = 7.8, 0.6 Hz, 1H), 6.80 (s, 1H), 6.04 (s, 2H), 5.32–5.37 (m, 1H), 5.20–5.24 (m, 1H), 4.41 (s, 3H), 4.07 (s, 3H), 3.33–3.38 (m, 1H), 3.24–3.29 (m, 1H); 13C NMR (150 MHz, CDCl3): δ 150.6, 150.6, 148.2, 147.4, 145.5, 144.3, 137.8, 133.0, 131.5, 131.2, 130.6, 130.5, 127.6, 122.7, 121.3, 120.5, 118.8, 118.5, 116.1, 108.6, 105.3, 102.1, 63.2, 57.1, 56.1, 27.5. HRMS (ESI): m/z for C26H23N2O4 [M − Cl]+ calcd 427.1652, found 427.1663.
9,10-Dimethoxy-12-(4-nitrophenyl)-5,6-dihydro-[1,3]dioxolo[4,5-g]isoquinolino[3,2-a]isoquinolin-7-ium chloride (3j). Purification by silica gel column chromatography (95
:
5 CHCl3/MeOH) afforded 5.6 mg (61%) of 3j as yellow solid. TLC Rf = 0.28 (95
:
5 CHCl3/MeOH); mp 190–192 °C; 1H NMR (600 MHz, CDCl3): δ 10.65 (s, 1H), 8.48 (d, J = 8.4 Hz, 2H), 7.94 (s, 1H), 7.73 (s, 1H), 7.68 (d, J = 8.4 Hz, 2H), 6.99 (s, 1H), 6.82 (s, 1H), 6.06 (s, 2H), 5.31 (t, J = 6.0 Hz, 2H), 4.46 (s, 3H), 4.10 (s, 3H), 3.34 (t, J = 6.0 Hz, 2H); 13C NMR (150 MHz, CDCl3): δ 150.9, 150.1, 148.4, 148.2, 148.2, 146.7, 143.4, 138.4, 132.8, 131.0, 130.8, 126.7, 124.6, 122.5, 120.0, 116.6, 108.8, 104.9, 102.2, 63.4, 57.3, 56.1, 27.5. HRMS (ESI): m/z for C26H21N2O6 [M − Cl]+ calcd 457.1394, found 457.1412.
12-(4-Fluorophenyl)-9,10-dimethoxy-5,6-dihydro-[1,3]dioxolo[4,5-g]isoquinolino[3,2-a]isoquinolin-7-ium chloride (3k). Purification by silica gel column chromatography (95
:
5 CHCl3/MeOH) afforded 5.9 mg (58%) of 3k as yellow solid. TLC Rf = 0.28 (95
:
5 CHCl3/MeOH); mp 196–198 °C; 1H NMR (600 MHz, CDCl3): δ 10.59 (s, 1H), 8.02 (s, 1H), 7.70 (s, 1H), 7.45 (d, J = 5.4 Hz, 2H), 7.44 (d, J = 5.4 Hz, 2H), 7.01 (s, 1H), 6.82 (s, 1H), 6.06 (s, 2H), 5.32 (t, J = 6.0 Hz, 2H), 4.42 (s, 3H), 4.08 (s, 3H), 3.33 (t, J = 6.0 Hz, 2H); 13C NMR (150 MHz, CDCl3): δ 162.3 (d, J = 250.49 Hz), 150.7, 150.2, 148.3, 147.7, 145.6, 137.9, 134.7, 132.8 (d, J = 3.02 Hz), 131.4 (d, J = 7.55 Hz), 130.9, 126.6, 122.5, 120.3, 117.4, 116.4 (d, J = 22.64 Hz), 108.8, 104.9, 102.2, 63.3, 57.2, 56.0, 27.5. HRMS (ESI): m/z for C26H21FNO4 [M − Cl]+ calcd 430.1449, found 430.1477.
12-(3-Fluorophenyl)-9,10-dimethoxy-5,6-dihydro-[1,3]dioxolo[4,5-g]isoquinolino[3,2-a]isoquinolin-7-ium chloride (3l). Purification by silica gel column chromatography (95
:
5 CHCl3/MeOH) afforded 6.1 mg (60%) of 3l as yellow solid. TLC Rf = 0.28 (95
:
5 CHCl3/MeOH); mp 197–199 °C; 1H NMR (600 MHz, CDCl3): δ 10.62 (s, 1H), 8.06 (s, 1H), 7.72 (s, 1H), 7.57–7.59 (m, 1H), 7.25–7.29 (m, 2H), 7.18–7.20 (m, 1H), 7.02 (s, 1H), 6.82 (s, 1H), 6.06 (s, 2H), 5.33 (t, J = 6.0 Hz, 2H), 4.42 (s, 3H), 4.08 (s, 3H), 3.33 (t, J = 6.0 Hz, 2H); 13C NMR (150 MHz, CDCl3): δ 162.2 (d, J = 248.68 Hz), 150.7, 150.2, 148.3, 147.8, 145.9, 138.9 (d, J = 20.98 Hz) 138.0, 134.2 (d, J = 3.02 Hz), 131.3, 131.0 (d, J = 7.55 Hz), 130.9, 126.6, 125.5 (d, J = 2.72 Hz), 122.5, 120.2, 117.3, 116.8 (d, J = 22.03 Hz), 116.0 (d, J = 20.98 Hz), 108.8, 105.0, 102.1, 63.4, 57.2, 56.0, 27.5. HRMS (ESI): m/z for C26H21FNO4 [M − Cl]+ calcd 430.1449, found 430.1462.
12-(2-Fluorophenyl)-9,10-dimethoxy-5,6-dihydro-[1,3]dioxolo[4,5-g]isoquinolino[3,2-a]isoquinolin-7-ium chloride (3m). Purification by silica gel column chromatography (95
:
5 CHCl3/MeOH) afforded 5.3 mg (52%) of 3m as yellow solid. TLC Rf = 0.28 (95
:
5 CHCl3/MeOH); mp 194–197 °C; 1H NMR (600 MHz, CDCl3): δ 10.64 (s, 1H), 7.87 (d, J = 2.4 Hz, 1H), 7.75 (s, 1H), 7.57–7.61 (m, 1H), 7.39–7.44 (m, 2H), 7.33–7.56 (m, 1H), 7.03 (s, 1H), 6.82 (s, 1H), 6.06 (s, 2H), 5.49–5.53 (m, 1H), 5.16–5.19 (m, 1H), 4.43 (s, 3H), 4.08 (s, 3H), 3.40–3.42 (m, 1H), 3.24–3.28 (m, 1H); 13C NMR (150 MHz, CDCl3): δ 158.8 (d, J = 248.68 Hz), 150.7, 150.2, 148.2, 147.7, 146.1, 138.0, 132.1 (d, J = 2.11 Hz), 131.7, 131.3 (d, J = 7.70 Hz), 131.0, 129.2, 127.7, 125.2 (d, J = 3.32 Hz), 124.1 (d, J = 15.39 Hz), 122.5, 120.3, 117.6 (d, J = 2.26 Hz), 116.4 (d, J = 22.03 Hz), 108.8, 105.0, 102.1, 63.3, 57.2, 56.0, 27.6. HRMS (ESI): m/z for C26H21FNO4 [M − Cl]+ calcd 430.1449, found 430.1436.
9,10-Dimethoxy-12-(3-(trifluoromethyl)phenyl)-5,6-dihydro-[1,3]dioxolo[4,5-g]isoquinolino[3,2-a]isoquinolin-7-ium chloride (3n). Purification by silica gel column chromatography (95
:
5 CHCl3/MeOH) afforded 7.3 mg (64%) of 3n as yellow solid. TLC Rf = 0.29 (95
:
5 CHCl3/MeOH); mp 176–178 °C; 1H NMR (600 MHz, CDCl3): δ 10.65 (s, 1H), 7.98 (s, 1H), 7.85 (d, J = 7.2 Hz, 1H), 7.75–7.77 (m, 1H), 7.75 (s, 1H), 7.73 (s, 1H), 7.68 (d, J = 7.2 Hz, 1H), 6.98 (s, 1H), 6.82 (s, 1H), 6.06 (s, 2H), 5.31 (t, J = 6.0 Hz, 2H), 4.44 (s, 3H), 4.10 (s, 3H), 3.33 (t, J = 6.0 Hz, 2H); 13C NMR (150 MHz, CDCl3): δ 150.8, 150.2, 148.4, 148.0, 146.2, 138.2, 137.7, 133.8, 133.1, 132.2 (q, J = 32.90 Hz) 131.3, 130.9, 129.9, 126.7, 126.7 (q, J = 4.53 Hz), 126.5 (q, J = 272.83 Hz), 125.8 (q, J = 3.32 Hz), 122.5, 120.2, 117.0, 108.8, 104.9, 102.2, 63.4, 57.2, 56.0, 27.5. HRMS (ESI): m/z for C27H21F3NO4 [M − Cl]+ calcd 480.1417, found 480.1430.
9,10-Dimethoxy-12-(pyridin-4-yl)-5,6-dihydro-[1,3]dioxolo[4,5-g]isoquinolino[3,2-a]isoquinolin-7-ium chloride (3o). Purification by silica gel column chromatography (gradient elution from 95
:
5 to 90
:
10 CHCl3/MeOH) afforded 4.0 mg (40%) of 3o as yellow solid. TLC Rf = 0.20 (95
:
5 CHCl3/MeOH); mp 200–202 °C; 1H NMR (600 MHz, CDCl3): δ 10.60 (s, 1H), 8.88 (d, J = 6.0 Hz, 2H), 8.02 (s, 1H), 7.72 (s, 1H), 7.43 (d, J = 6.0 Hz, 2H), 7.01 (s, 1H), 6.82 (s, 1H), 6.07 (s, 2H), 5.29 (t, J = 6.0 Hz, 2H), 4.44 (s, 3H), 4.10 (s, 3H), 3.33 (t, J = 6.0 Hz, 2H); 13C NMR (150 MHz, CDCl3): δ 150.9, 150.8, 150.2, 148.4, 148.2, 146.6, 144.8, 138.4, 132.4, 131.0, 130.8, 126.5, 124.5, 122.5, 120.1, 116.6, 108.8, 104.9, 102.2, 63.4, 57.3, 56.1, 27.5. HRMS (ESI): m/z for C25H21N2O4 [M − Cl]+ calcd 413.1496, found 413.1499.
9,10-Dimethoxy-12-(thiophen-3-yl)-5,6-dihydro-[1,3]dioxolo[4,5-g]isoquinolino[3,2-a]isoquinolin-7-ium chloride (3p). Purification by silica gel column chromatography (95
:
5 CHCl3/MeOH) afforded 4.3 mg (43%) of 3p as yellow solid. TLC Rf = 0.27 (95
:
5 CHCl3/MeOH); mp 198–200 °C; 1H NMR (600 MHz, CDCl3): δ 10.58 (s, 1H), 8.23 (s, 1H), 7.76 (s, 1H), 7.62 (dd, J = 4.8, 3.0 Hz, 1H), 7.49 (d, J = 3.0 Hz, 1H), 7.29 (d, J = 4.8 Hz, 1H), 7.09 (s, 1H), 6.82 (s, 1H), 6.07 (s, 2H), 5.32 (t, J = 6.0 Hz, 2H), 4.30 (s, 3H), 4.08 (s, 3H), 3.33 (t, J = 6.0 Hz, 2H); 13C NMR (150 MHz, CDCl3): δ 150.6, 150.3, 148.3, 147.6, 145.5, 137.9, 131.7, 130.9, 130.9, 130.7, 128.6, 127.6, 126.3, 125.1, 122.6, 120.4, 117.6, 108.8, 105.0, 102.1, 63.3, 57.1, 56.0, 27.6. HRMS (ESI): m/z for C24H20NO4S [M − Cl]+ calcd 418.1108, found 418.1133.
12-(Furan-2-yl)-9,10-dimethoxy-5,6-dihydro-[1,3]dioxolo[4,5-g]isoquinolino[3,2-a]isoquinolin-7-ium chloride (3q). Purification by silica gel column chromatography (95
:
5 CHCl3/MeOH) afforded 4.8 mg (50%) of 3q as yellow solid. TLC Rf = 0.27 (95
:
5 CHCl3/MeOH); mp 191–193 °C; 1H NMR (600 MHz, CDCl3): δ 10.63 (s, 1H), 8.72 (s, 1H), 7.96 (s, 1H), 7.73 (d, J = 1.8 Hz, 1H), 7.28 (s, 1H), 6.85 (s, 1H), 6.82 (d, J = 3.6 Hz, 1H), 6.69 (dd, J = 3.6, 1.8 Hz, 1H), 6.10 (s, 2H), 5.34 (t, J = 6.0 Hz, 2H), 4.41 (s, 3H), 4.11 (s, 3H), 3.33 (t, J = 6.0 Hz, 2H); 13C NMR (150 MHz, CDCl3): δ 150.7, 150.3, 150.2, 148.4, 147.7, 146.0, 144.0, 138.0, 130.9, 129.9, 124.4, 124.0, 122.7, 120.4, 117.6, 112.3, 110.6, 108.8, 105.0, 102.2, 63.4, 57.1, 55.9, 27.6. HRMS (ESI): m/z for C24H20NO5 [M − Cl]+ calcd 402.1336, found 402.1343.
12-(Furan-3-yl)-9,10-dimethoxy-5,6-dihydro-[1,3]dioxolo[4,5-g]isoquinolino[3,2-a]isoquinolin-7-ium chloride (3r). Purification by silica gel column chromatography (95
:
5 CHCl3/MeOH) afforded 4.3 mg (45%) of 3r as yellow solid. TLC Rf = 0.26 (95
:
5 CHCl3/MeOH); mp 192–194 °C; 1H NMR (600 MHz, CDCl3): δ 10.57 (s, 1H), 8.29 (s, 1H), 7.73 (s, 2H), 7.71 (s, 1H), 7.15 (s, 1H), 6.83 (s, 1H), 6.69 (s, 1H), 6.09 (s, 2H), 5.33 (t, J = 6.0 Hz, 2H), 4.40 (s, 3H), 4.09 (s, 3H), 3.33 (t, J = 6.0 Hz, 2H); 13C NMR (150 MHz, CDCl3): δ 150.7, 150.4, 148.4, 147.6, 145.5, 144.4, 140.9, 137.9, 131.8, 130.9, 126.7, 126.3, 122.7, 121.9, 120.3, 117.4, 111.6, 108.8, 104.9, 102.2, 63.3, 57.1, 56.0, 27.6. HRMS (ESI): m/z for C24H20NO5 [M − Cl]+ calcd 402.1336, found 402.1372.
Reporter assay for inhibiting HIF-1 transcriptional activity11
Berberine and its analogues were dissolved in DMSO to 10 mM as stock solution and then diluted in DMEM media to appropriate concentration. T47D cells (American Type Culture Collection) were cultured in DMEM medium (Invitrogen), supplemented with 10% (v/v) fetal bovine serum (FBS) (Invitrogen), 100 U mL−1 penicillin, and 100 μg mL−1 streptomycin (Invitrogen) in a humidified atmosphere (5% CO2 and 95% air) at 37 °C. Cells (5 × 106 cells) were co-transfected with the HRE-luciferase (Addgene) and Renilla plasmids using Lipofectamine 2000 (Invitrogen) according to the manufacturer's protocol. The transfected cells were seeded in 96-well plates with a density of 3 × 104 per well and cultured in DMEM with 10% FBS overnight. After addition of berberine analogues with different concentrations (0.3–50 μM), the cells were incubated for 1 h, and then were exposed to the hypoxic (2% O2/5% CO2/93% N2) or normoxic (5% CO2/95% air) conditions at 37 °C for 20 h. Then the cells were finally lysed, and luciferase activities of both HRE and Renilla were measured by Dual-Luciferase® reporter assay (Promega) kit according to manufacturer's instructions by using a multimode reader (Infinite 200 PRO, Tecan). HIF-1 transcriptional activity was shown by the ratio of firefly/Renilla luciferase activity. IC50 values were determined from the dose-response curves using the Prsim software. The data were repeated by three independent experiments.
Western blot assay
T47D or MCF-7 cells, at a density of 1 × 106 cells per well, were seeded in 6-well plates and cultured for 24 hours to allow attachment. Various concentrations of berberine and its derivatives were added to the cells which were incubated under hypoxic condition (2% O2, 5% CO2, and 93% N2) for 24 hours. Meanwhile, vehicle treatment was also performed as control under both hypoxic and normoxic conditions in parallel. The supernatant was removed and each well of cells was lysed with RIPA buffer (Cell Signaling Technology) containing protease inhibitor cocktail (Roche). Cell lysates were then harvested into 0.5 mL microtube and boiled at 99 °C for 10 minutes. Subsequently, the total proteins of lysates were separated by 8% SDS polyacrylamide gel electrophoresis. After separation, the proteins in the gel were transferred onto nitrocellulose membranes (Pall), and the transferred membranes were blocked with 5% nonfat dry milk in 1 × TBST for 1 hour at room temperature. After being washed three times in 1 × TBST, these parts of membranes containing target HIF-1α and reference β-actin proteins were divided apart according to the protein markers, and incubated respectively in corresponding primary mouse anti-human HIF-1α antibody (BD Biosciences) at a dilution of 1
:
200 (v/v) and mouse anti-human β-actin antibody (Santa Cruz Biotechnology) at a dilution of 1
:
8000 (v/v). After overnight incubation at 4 °C, the primary antibodies were washed away with 1 × TBST, and IRDye-conjugated goat anti-mouse secondary antibodies (LI-COR Biosciences) were added at a ratio of 1
:
5000 (v/v) for HIF-1α and 1
:
20
000 (v/v) for β-actin for 1 hour incubation at room temperature. After three washes with 1 × TBST, the membranes were scanned with an Odyssey infrared fluorescent scanner (LI-COR Biosciences). Finally, the amounts of proteins were characterized according to the optical densities of the bands. The amount of β-actin was served as a loading control.
Cytotoxic assay on MCF-7 breast cancer cell
MCF-7 cell line (American Type Culture Collection) was cultured in DMEM medium (Invitrogen) supplemented with 10% (v/v) heat-inactivated fetal bovine serum (FBS), penicillin (100 U mL−1) and streptomycin (10 μg mL−1) in a humidified atmosphere of 5% CO2/95% air at 37 °C. Cells were seeded in 96-well microplates at a density of 5 × 103 cells per well (100 μL), and cultured at 37 °C under 5% CO2/95% air for 24 h. Then, the cells were treated with fresh medium containing berberine analogues with appropriate concentrations (0.3–50 μM) and further cultured for 72 h. After that, the supernatant in each well was removed and the cell was gently washed by phosphate buffered saline (PBS) to avoid the possible effect of tested compounds on the following MTT assay. A volume of 100 μL medium containing 0.5 mg mL−1 MTT was added into each well and further cultured for 4 h at 37 °C. Then, the supernatant was discarded and the formed formazan crystals were dissolved in DMSO (100 μL). Cell viability was determined by measuring the absorbance of each well at 570 nm using a microplate reader (Infinite 200 PRO, Tecan). The IC50 values (concentration that inhibits 50% of cell growth) of tested compounds were calculated using the GraphPad Prism 6 software. All assays were conducted in triplicate in three independent experiments. Data was expressed as mean ± SD (n = 3).
Acknowledgements
This research was financially supported by the Macao Science and Technology Development Fund, MSAR (056/2013/A2 to Dr Li-Ping Bai). The content is solely the responsibility of the authors. The funders had no role in study design, data collection and analysis, decision to publish, or preparation of the manuscript.
Notes and references
- A. L. Harris, Nat. Rev. Cancer, 2002, 2, 38–47 CrossRef CAS PubMed.
- M. C. Brahimi-Horn, J. Chiche and J. Pouyssegur, J. Mol. Med., 2007, 85, 1301–1307 CrossRef PubMed.
- P. Carmeliet, Y. Dor, J.-M. Herbert, D. Fukumura, K. Brusselmans, M. Dewerchin, M. Neeman, F. Bono, R. Abramovitch, P. Maxwell, C. J. Koch, P. Ratcliffe, L. Moons, R. K. Jain, D. Collen and E. Keshet, Nature, 1998, 394, 485–490 CrossRef CAS PubMed.
- G. L. Wang and G. L. Semenza, J. Biol. Chem., 1995, 270, 1230–1237 CrossRef CAS PubMed.
- A. Giaccia, B. G. Siim and R. S. Johnson, Nat. Rev. Drug Discovery, 2003, 2, 803–811 CrossRef CAS PubMed.
- R. Sheng, S. Li, G. Lin, S. Shangguan, Y. Gu, N. Qiu, J. Cao, Q. He, B. Yang and Y. Hu, RSC Adv., 2015, 5, 81817–81830 RSC.
- D. G. Nagle and Y. D. Zhou, Curr. Drug Targets, 2006, 7, 355–369 CrossRef CAS PubMed.
- H. S. Ban, Y. Uto and H. Nakamura, Expert Opin. Ther. Pat., 2011, 21, 131–146 CrossRef CAS PubMed.
- H. S. Ban, Y. Uto, M. Won and H. Nakamura, Expert Opin. Ther. Pat., 2016, 26, 309–322 CrossRef CAS PubMed.
- H. Zhang, D. Z. Qian, Y. S. Tan, K. Lee, P. Gao, Y. R. Ren, S. Rey, H. Hammers, D. Chang, R. Pili, C. V. Dang, J. O. Liu and G. L. Semenza, Proc. Natl. Acad. Sci. U. S. A., 2008, 105, 19579–19586 CrossRef CAS PubMed.
- S. Parhira, G.-Y. Zhu, R.-W. Jiang, L. Liu, L.-P. Bai and Z.-H. Jiang, Sci. Rep., 2014, 4, srep04748 Search PubMed.
- N. J. Mabjeesh, D. E. Post, M. T. Willard, B. Kaur, E. G. Van Meir, J. W. Simons and H. Zhong, Cancer Res., 2002, 62, 2478–2482 CAS.
- A. L. Kung, S. D. Zabludoff, D. S. France, S. J. Freedman, E. A. Tanner, A. Vieira, S. Cornell-Kennon, J. Lee, B. Wang, J. Wang, K. Memmert, H.-U. Naegeli, F. Petersen, M. J. Eck, K. W. Bair, A. W. Wood and D. M. Livingston, Cancer Cell, 2004, 6, 33–43 CrossRef CAS PubMed.
- T. W. Hodges, C. F. Hossain, Y. P. Kim, Y. D. Zhou and D. G. Nagle, J. Nat. Prod., 2004, 67, 767–771 CrossRef CAS PubMed.
- C.-Y. Chen, G.-Y. Zhu, J.-R. Wang and Z.-H. Jiang, RSC Adv., 2016, 6, 79958–79967 RSC.
- S. Lin, S. C. Tsai, C. C. Lee, B. W. Wang, J. Y. Liou and K. G. Shyu, Mol. Pharmacol., 2004, 66, 612–619 CAS.
- L. Fu, W. Chen, W. Guo, J. Wang, Y. Tian, D. Shi, X. Zhang, H. Qiu, X. Xiao, T. Kang, W. Huang, S. Wang and W. Deng, PLoS One, 2013, 8, e69240 CAS.
- C. Zhang, X. Yang, Q. Zhang, B. Yang, L. Xu, Q. Qin, H. Zhu, J. Liu, J. Cai, G. Tao, J. Ma, X. Ge, S. Zhang, H. Cheng and X. Sun, Acta Oto-Laryngol., 2014, 134, 185–192 CrossRef CAS PubMed.
- Q. Zhang, C. Zhang, X. Yang, B. Yang, J. Wang, Y. Kang, Z. Wang, D. Li, G. Huang, Z. Ma, X. Sun, J. Cai, G. Tao, S. Dai, W. Mao and J. Ma, Diagn. Pathol., 2014, 9, 98 CrossRef PubMed.
- A. Kumar, E. Ekavali, K. Chopra, M. Mukherjee, R. Pottabathini and D. K. Dhull, Eur. J. Pharmacol., 2015, 761, 288–297 CrossRef CAS PubMed.
- M. Tillhon, L. M. Guaman Ortiz, P. Lombardi and A. I. Scovassi, Biochem. Pharmacol., 2012, 84, 1260–1267 CrossRef CAS PubMed.
- A. Pirillo and A. L. Catapano, Atherosclerosis, 2015, 243, 449–461 CrossRef CAS PubMed.
- Y. Hu, E. A. Ehli, J. Kittelsrud, P. J. Ronan, K. Munger, T. Downey, K. Bohlen, L. Callahan, V. Munson, M. Jahnke, L. L. Marshall, K. Nelson, P. Huizenga, R. Hansen, T. J. Soundy and G. E. Davies, Phytomedicine, 2012, 19, 861–867 CrossRef CAS PubMed.
- N. Wang, H. Y. Tan, L. Li, M. F. Yuen and Y. Feng, J. Ethnopharmacol., 2015, 176, 35–48 CrossRef CAS PubMed.
- C.-L. Kuo, C.-W. Chi and T.-Y. Liu, Cancer Lett., 2004, 203, 127–137 CrossRef CAS PubMed.
- E. N. Simões Pires, R. L. Frozza, J. B. Hoppe, B. d. M. Menezes and C. G. Salbego, Brain Res., 2014, 1557, 26–33 CrossRef PubMed.
- K. Karaosmanoglu, N. A. Sayar, I. A. Kurnaz and B. S. Akbulut, OMICS, 2014, 18, 42–53 CrossRef CAS PubMed.
- Z. J. Huang, Y. Zeng, P. Lan, P. H. Sun and W. M. Chen, Mini-Rev. Med. Chem., 2011, 11, 1122–1129 CrossRef CAS PubMed.
- S. Q. Wen, P. Jeyakkumar, S. R. Avula, L. Zhang and C. H. Zhou, Bioorg. Med. Chem. Lett., 2016, 26, 2768–2773 CrossRef CAS PubMed.
- P. Jeyakkumar, L. Zhang, S. R. Avula and C. H. Zhou, Eur. J. Med. Chem., 2016, 122, 205–215 CrossRef CAS PubMed.
- S. Zhang, X. Wang, W. Yin, Z. Liu, M. Zhou, D. Xiao, Y. Liu and D. Peng, Bioorg. Med. Chem. Lett., 2016, 26, 4799–4803 CrossRef CAS PubMed.
- X. Jin, L. Yan, H.-j. Li, R.-L. Wang, Z.-L. Hu, Y.-Y. Jiang, Y.-B. Cao, T.-H. Yan and Q.-Y. Sun, Adv. Anticancer Agents Med. Chem., 2015, 15, 89–98 CrossRef CAS.
- T. Su, S. Xie, H. Wei, J. Yan, L. Huang and X. Li, Bioorg. Med. Chem., 2013, 21, 5830–5840 CrossRef CAS PubMed.
- L. Zhang, J.-J. Chang, S.-L. Zhang, G. L. V. Damu, R.-X. Geng and C.-H. Zhou, Bioorg. Med. Chem., 2013, 21, 4158–4169 CrossRef CAS PubMed.
- D. Bhowmik, F. Buzzetti, G. Fiorillo, F. Orzi, T. M. Syeda, P. Lombardi and G. S. Kumar, MedChemComm, 2014, 5, 226–231 RSC.
- Z. Cheng, A. F. Chen, F. Wu, L. Sheng, H. K. Zhang, M. Gu, Y. Y. Li, L. N. Zhang, L. H. Hu, J. Y. Li and J. Li, Bioorg. Med. Chem., 2010, 18, 5915–5924 CrossRef CAS PubMed.
- M. Tera, T. Hirokawa, S. Okabe, K. Sugahara, H. Seimiya and K. Shimamoto, Chem.–Eur. J., 2015, 21, 14519–14528 CrossRef CAS PubMed.
- K. Iwasa, D.-U. Lee, S.-I. Kang and W. Wiegrebe, J. Nat. Prod., 1998, 61, 1150–1153 CrossRef CAS PubMed.
- I. V. Nechepurenko, U. A. Boyarskikh, N. I. Komarova, M. P. Polovinka, M. L. Filipenko, G. I. Lifshits, N. F. Salakhutdinov and G. A. Tolstikov, Dokl. Chem., 2011, 439, 204–208 CrossRef CAS.
- W.-H. Chen, Y. Qin, Z. Cai, C.-L. Chan, G.-A. Luo and Z.-H. Jiang, Bioorg. Med. Chem., 2005, 13, 1859–1866 CrossRef CAS PubMed.
- J.-Y. Pang, Y. Qin, W.-H. Chen, G.-A. Luo and Z.-H. Jiang, Bioorg. Med. Chem., 2005, 13, 5835–5840 CrossRef CAS PubMed.
- W. H. Chen, J. Y. Pang, Y. Qin, Q. Peng, Z. Cai and Z. H. Jiang, Bioorg. Med. Chem. Lett., 2005, 15, 2689–2692 CrossRef CAS PubMed.
- Y. Qin, J. Y. Pang, W. H. Chen, Z. Cai and Z. H. Jiang, Bioorg. Med. Chem., 2006, 14, 25–32 CrossRef CAS PubMed.
- Y.-H. Long, L.-P. Bai, Y. Qin, J.-Y. Pang, W.-H. Chen, Z. Cai, Z.-L. Xu and Z.-H. Jiang, Bioorg. Med. Chem., 2006, 14, 4670–4676 CrossRef CAS PubMed.
- Y. Qin, J.-Y. Pang, W.-H. Chen, Z.-Z. Zhao, L. Liu and Z.-H. Jiang, Chem. Biodiversity, 2007, 4, 481–487 CAS.
- S. Parhira, G.-Y. Zhu, M. Chen, L.-P. Bai and Z.-H. Jiang, J. Ethnopharmacol., 2016, 194, 930–936 CrossRef CAS PubMed.
- M. Ishikawa and Y. Hashimoto, J. Med. Chem., 2011, 54, 1539–1554 CrossRef CAS PubMed.
- A. L. Wolfe, K. K. Duncan, J. P. Lajiness, K. Zhu, A. S. Duerfeldt and D. L. Boger, J. Med. Chem., 2013, 56, 6845–6857 CrossRef CAS PubMed.
Footnotes |
† Electronic supplementary information (ESI) available. See DOI: 10.1039/c7ra02238g |
‡ Authors contribute equally. |
|
This journal is © The Royal Society of Chemistry 2017 |