DOI:
10.1039/C7RA01279A
(Paper)
RSC Adv., 2017,
7, 23875-23881
Preliminary characterization and immunostimulatory activity of a novel functional polysaccharide from Astragalus residue fermented by Paecilomyces sinensis
Received
30th January 2017
, Accepted 20th April 2017
First published on 3rd May 2017
Abstract
An Astragalus residue was reutilized through solid-state fermentation by Paecilomyces sinensis, which is a member of Ophiocordyceps sinensis (Berk.) Sacc. In this research, the polysaccharide from the product of solid-state fermentation primarily was studied. First, HPLC, FT-IR, and GC were applied to study the preliminary characterization of a polysaccharide (APP-II) from the solid-state fermentation product Astragalus residue and Paecilomyces sinensis. Results indicated that APP-II is α-pyran polysaccharide with an average molecular weight of 670 kD that is composed of rhamnose, arabinose, xylose, mannose, glucose, and galactose with a molar ratio of 4.0
:
4.2
:
2.0
:
41.4
:
22.5
:
25.9. Second, an immunostimulatory activity of APP-I and APP-II was detected. Results suggested that APP-I and APP-II could promote RAW264.7 cell proliferation. APP-I was used to detect the immunostimulatory activity of APP-II in vivo. The results indicated that APP-I can promote growth of immune organs, stimulate splenocyte proliferation, and enhance NK activity; this indicated that APP-II had immunostimulatory activity in vivo. All the results indicated that APP-II is a novel polysaccharide with immunostimulatory activity.
Introduction
Astragalus (Plant kingdom, Angiospermae, Dicotyledons, Fabales, Leguminosae) has a long history of medicinal use in traditional Chinese medicine. It is commonly used as an agent for immunopotentiation in mixed herbal decoctions to treat common cold, diarrhea, fatigue and anorexia.1 Astragalus polysaccharides (APS) have various important biological activities such as anti-oxidant activity, immunomodulatory activity, anti-tumor activity, anti-diabetes activity, antiviral activity, hepatoprotection activity, and anti-inflammation activity.2–10 In industrial production, most methods used to extract APS involve water extraction and alcohol precipitation. These methods not only leave many other bioactive substances behind, but also produce a large amount of Astragalus residue. Literature review shows that Astragalus residue still contains many nutrients such as crude fiber, crude protein, crude fat, amino acids, lignin, and also includes abundant medicinal components such as alkaloids, terpenoids, and saponinsit.11 Adding Astragalus residue to the diet of broiler chickens can significantly reduce the apparent metabolic rate of crude fat, which gradually decreased with increasing amounts of residue.12
Ophiocordyceps sinensis (Berk.) Sacc. (i.e. Cordyceps sinensis) are entomo-parasitic fungi and have been used as valuable Chinese traditional medicines.13,14 Ophiocordyceps sinensis (Berk.) Sacc. belong to the fungi kingdom, Pezizomycotina, Sordariomycetes, Hypocreales, Ophiocordycipitaceae.13 Studies have suggested that Ophiocordyceps sinensis (Berk.) Sacc. have many functions with bodies, such as activities of immunomodulatory, anti-fibrotic, anti-oxidation, anti-tumorigenic, hypolipidemic, anti-inflammation, hypotensive and vasorelaxant.15–23 It also can regulate insulin sensitivity and decrease plasma cholesterol levels.24,25
When taking account of the values of Astragalus residue and Paecilomyces sinensis, we included the reutilization of Astragalus residue by biotransformation from our previously study. The Astragalus residue was reutilized through solid-state fermentation by Paecilomyces sinensis, which is a member of Ophiocordyceps sinensis (Berk.) Sacc. and the fermentation conditions were accordant with our previous study.26 The product of the solid-state fermentation of Astragalus residue and Paecilomyces sinensis was named “AP”. The content of soluble sugar in AP was 9.39%, which is 58.08% higher than in the Astragalus residue.27 So in this research, the polysaccharide from AP was mainly studied; first, a preliminary characterization of the polysaccharide was described and then immunostimulatory activity of polysaccharide was detected both in vivo and in vitro.
Materials and methods
Materials and chemicals
BALB/c mice (6–8 weeks old and weighing 18–22 g) were obtained from Fukang Biotechnology Co. Ltd (Beijing, China). All mice were specific pathogen free (SPF) animals and their certificate number is SCXK (Beijing) 2009-0004.
RAW264.7 macrophages were purchased from ATCC (American Type Tissue Culture collection).
RPMI-1640 medium and fetal calf serums (FCS) were purchased from HyClone (Logan, UT, USA). 3-(4,5-Dimethylthiazol-2-yl)-2,5-diphenyl-tetrazolium bromide (MTT), concanavalin A (ConA) and lipopolysaccharide (LPS) were purchased from Sigma Chemical Co. (St. Louis, MO, USA). All solvents and reagents were obtained from commercial sources and were analytical reagent (AR) grade.
Live subject statement
Animal welfare and experimental procedures were carried out in accordance with the relevant laws and the Guide for the Care and Use of Laboratory Animals (Ministry of Science and Technology of China, 2006), and were approved by the animal ethics committee of Tianjin University of Science and Technology. No experiments involved human subjects.
Extraction and purification of polysaccharides
AP was mixed with distilled water (1
:
15 ratio of raw material to water, w/v) in a round-bottom flask at 80 °C for 2 h. Then, the suspension was centrifuged with a centrifugal machine (Sorvall ST16R, Thermo, USA) and the insoluble residue was handled twice as described above. The supernatant was evaporated with a rotary evaporator (Sy-2000, Shanghai Ya Rong, China) at 60 °C and lyophilized (SA-15, ShenzhenYujia, China) to give an aqueous extract. The content of soluble sugar was determined by using a phenol–sulfuric acid method.28 The aqueous extract of AP was dissolved in distilled water and that solution was treated by precipitation with 4 times volume of 80% (v/v) ethanol, deproteinized with the Sevag method, ultra-filtrated (ultra-filtrate tubes with a molecular cut off weight of 10 kDa), and lyophilization (SA-15, ShenzhenYujia, China) to give the crude polysaccharide.29,30 This crude polysaccharide of AP was named “APP-I”. Then it was purified by Sephadex G-100 eluting with deionized water at a flow rate of 0.4 mL min−1 and 2 min per tube, monitored by the phenol–sulfuric acid method. Polysaccharides with greater molecular weights should elute first. Therefore, the first fraction was collected and lyophilized as pure polysaccharide. The pure polysaccharide was named “APP-II”. Total sugar content of APP-II was measured by a phenol–sulfuric acid method and its purity was determined using a UV spectral-scanning method.
Molecular weight distribution analysis of APP-I and APP-II
Molecular weight (Mw) distribution of the polysaccharide was determined using a HPGPC (Agilent-1200, USA) equipped with a TSK-GEL G4000 PWXL column (7.8 mm × 300 mm, column temperature 30 °C) and Refractive Index Detector (RID, detecting temperature 35 °C). The sample solution (10 μL) was injected and run with deionized water at 0.6 mL min−1 as the mobile phase. A standard curve was established using T-series Dextran as the standards (T-10, T-40, T-70, T-500 and T-2000).31
FT-IR analysis of APP-II
IR spectra analysis was performed by grinding a mixture of 1 mg polysaccharide with 150 mg of dried KBr, and pressing it into a 1 mm pellet. Spectra were recorded in the absorbance mode in the region of 4000–400 cm−1 on a FT-IR spectrophotometer (VECTOR-22, PerkinElmer Corp., USA).32
Monosaccharide composition analysis of APP-II
5.0 mg of dried sample was hydrolyzed with 1 mL of 2 M TFA in an oil bath at 110 °C for 3 h and the soluble fraction was evaporated to dryness under a stream of nitrogen. After TFA was removed, the dried sample was acetylated with Ac2O–pyridine (1
:
1, v/v) at 90 °C for 1 h.33 The acetylated sample was completely dissolved in dichloromethane for further GC (GC2010, Shimadzu, Japan) analysis. D-glucose, D-xylose, D-galactose, L-rhamnose, D-mannose, and D-arabinose standards were also derived for standards.
Immunostimulatory activity of APP-I and APP-II in vitro
The immunostimulatory activities of APP-I and APP-II were performed using RAW264.7 cells. The effects of APP-I and APP-II on proliferation of RAW264.7 cells were determined by the 3-(4,5-dimethylthiazol-2-yl)-2,5-diphenyltetrazolium bromide (MTT) assay. Cells were counted with a hemocytometer using a trypan blue stain assay after recovery. Then the concentration of spleen cells was adjusted to 5 × 104 per mL. An aliquot of 100 μL of RAW264.7 cells was seeded in a 96-well flat bottom microtiter plate and the plate was incubated at 37 °C in an incubator with 5% CO2 for 48 h. Then the plate was centrifuged at 1000 rpm for 10 min and the supernatant was discarded. Next, a RPMI-1640 medium (200 μL) with APP-I or APP-II was added. The concentrations of APP-I and APP-II were 0, 25, 50, 100, 200, and 400 μg mL−1, respectively. The positive control and negative controls were LPS (5 μg mL−1) and RPMI-1640 medium without polysaccharide, respectively. The incubation times were 24 h, 36 h, and 48 h, respectively. After this initial incubation, the cells were incubated with 20 μL MTT solution (5 mg mL−1) in a medium for 4 h at 37 °C. The viable cells convert MTT to formazan, which generates a blue-purple color after being dissolved in 150 μL of dimethyl sulfoxide (DMSO). Absorbance at 570 nm was measured with a microplate reader (Model 680, Bio-Rad, Hercules, CA, USA). All experiments were carried out in triplicate using the equation:
where CPR, ODE, and ODC, respectively represent cell proliferation, absorbance of experimental group, and absorbance of the negative control group.
Immunostimulatory activity of APP-II in vivo
Taking account of the low yield and efficiency of gel chromatography and the large amount of APP-II in APP-I, APP-I was used to study immunostimulatory activity of APP-II in mice with a large dosage of polysaccharide. Fifty-six BALB/c mice weighing 18–22 g were suitably cultured after a week in the animal room and randomly divided into four groups: one control group and three experimental groups. The experimental groups were divided into low dose (200 mg kg−1 bw), medium does (400 mg kg−1 bw) and high dose groups (1000 mg kg−1 bw). In addition to free access to a standard pellet diet and water, the experimental groups were fed every morning through intragastric administration (0.2 mL/10 g·bw) according to their dosage, and the control group was fed normal saline. All the mice were continuously fed for 21 days and all were weighed and recorded after the last day.
Determination of thymus and spleen indices. At 24 h after the last drug administration, the mice were killed using a cervical dislocation method. The spleen and thymus were dissected and weighed and the organ index was calculated as follows:
where OI, mow, and mbw, respectively represent organ index, organ weight (mg), and body weight (g).
Assay of splenocyte proliferation. Splenocyte proliferation was assessed using the MTT assay. A small portion of the spleen was used to prepare splenocytes; these were prepared from the spleens, strained with a stainless steel mesh (200 μm), and suspended in Hank's solution. The red cells were removed with an ACR buffer (8 g NH4Cl, 1 g Na2EDTA, 0.1 g KH2PO4, pH = 7.4). Then the single cell suspensions were washed three times with Hank's solution and suspended in a RPMI-1640 medium. Spleen cells were counted with a hemocytometer using a trypan blue stain assay. The concentration of spleen cells was adjusted to 2 × 107 per mL. Aliquots of 100 μL of splenocytes were seeded into 9 wells of a 96-well flat bottom microtiter plate. Then, ConA (Solarbio Co., Beijing, China) and LPS (Solarbio Co., Beijing, China) (final concentration 5 μg mL−1) aliquots were added, giving a final volume of 200 μL. After incubation at 37 °C in an incubator with 5% CO2 for 48 h, 20 μL of MTT solution (5 mg mL−1) was added to each well and incubated for another 4 h. The plate was centrifuged at 1000 rpm for 10 min and the supernatant was discarded. Then, 150 μL of DMSO was added to each well. The plate was shocked gently to dissolve formazan crystals. Optical density was measured at 570 nm wavelength on a microplate reader (1510, Thermo Fisher Scientific) using the equation:
where SI, ODT, and ODC, respectively represent stimulation index, optical density value of a test well, and optical density value of a control well.34
Assay of natural killer cell activity. Activity of natural killer (NK) cells was measured as previously described with some modifications. Briefly, H22 cells (purchased from Shanghai Institute of Cell Biology, Chinese Academy of Sciences, Shanghai, China) were cultured in BALB/c peritoneal for 2 weeks and used as target cells. Then, they were seeded in 96-well plates at a density of 1 × 106 cells per well in RPMI-1640 medium with 100 μL per well. After 24 h, splenocytes, used as the effector cells, were added at 2 × 107 cells per well to give effector/target cells a ratio of 20
:
1. After incubation for 4 h (37 °C, 5% CO2), each well plate was pulsed with 20 μL MTT (5 mg mL−1). The plate was further incubated for 4 h and DMSO (150 μL per well) was then added and gently shocked to dissolve formazan crystals. Optical density was measured using a microplate reader at 570 nm wavelength and NK cell activity was calculated using the following equation:
where NKA, ODT, ODS, and ODE, respectively represent NK activity, optical density value of target cells control, optical density value of test samples, and optical density value of effector cells control.35
Statistical analysis
Quantitative data were expressed as means ± SD. All statistical analyses were carried out using an ANOVA test by SPSS 8.0 (IBM Co., NY, USA).
Results and discussion
Extraction and purification of polysaccharides
The AP was extracted with distilled water at 80 °C and the yield of aqueous extract was 15.53%. The content of soluble sugar in aqueous extract was 75.43%. In this study, polysaccharide APP-I samples, derived from AP, were obtained with ethanol precipitation, deproteination, and ultrafiltration. The yield of APP-I from aqueous extract of AP was 65.32%. Weight-average molecular weight (Mw) distribution of APP-I was investigated and is shown in Fig. 1a. The first peaks (60.15%) of APP-I represent polysaccharides with high molecular weight and content. The APP-I was purified by gel-filtration chromatography on a Sephadex G-100 column, eluted with deionized water. Purity of APP-II was determined using a UV spectral scanning method. APP-II had no absorption peak at 260 nm and 280 nm, which proves that there are no nucleic acids, peptides, or proteins in them. Sugar content of APP-II was measured using a phenol sulfuric acid method and was 89.21%.
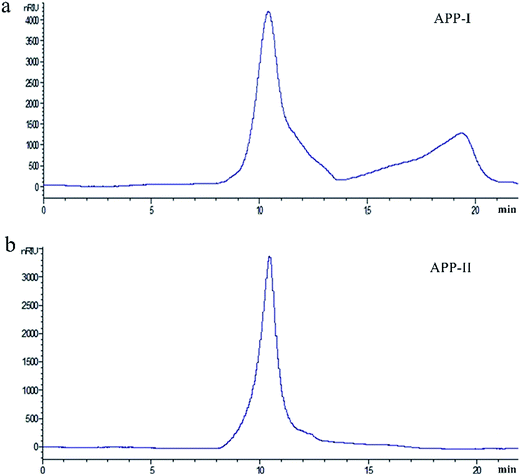 |
| Fig. 1 The weight-average molecular weight (Mw) distribution of APP-I (a) and APP-II (b). | |
Molecular weight distribution analysis. The molecular weight (Mw) of APP-II was measured by HPGPC. Fig. 1b shows that APP-II was all pure polysaccharides. The standard curve equation used was as follows: y = 0.0047x3 − 0.1804x2 + 1.9098x + 0.2378, R2 = 0.9969. The retention time of APP-II is 10.55 min and the molecular weight (Mw) of APP-II was calculated to be about 670 kDa.
FT-IR analysis. To study the primary structure of polysaccharides, APP-II was analyzed with FT-IR. Fig. 2 shows the infra-red spectrum of APP-II. The bands at 3385, 2930, and 1411 cm−1 are attributed to –OH stretching, C–H stretching of –CH2, and C–H bending vibrations, respectively. The band at 1639 cm−1 is attributed to C
O stretching vibration.36 These four bands are characteristic absorption bands of polysaccharides. The bands at 1152 cm−1, 1079 cm−1, and 1025 cm−1 indicate the pyran configurations of polysaccharides. The band at 857 cm−1 shows the α-type glycosidic linkages.37 Thus, APP-II is α-pyran polysaccharide.
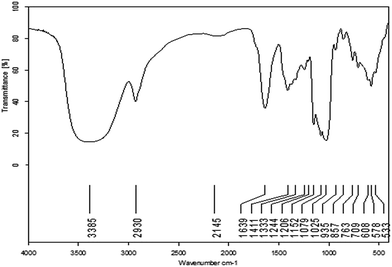 |
| Fig. 2 FT-IR spectra of APP-II. | |
Monosaccharide composition analysis. The gas chromatogram (GC) for monosaccharide composition analysis is shown as Fig. 3. GC analysis indicated that the APP-II is composed of rhamnose, arabinose, xylose, mannose, glucose, and galactose with a molar ratio of 4.0
:
4.2
:
2.0
:
41.4
:
22.5
:
25.9. It also shows that the large proportions of APP-II are mannose, glucose, and galactose.
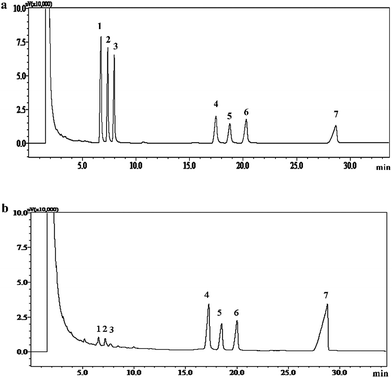 |
| Fig. 3 Gas chromatogram of standard monosaccharides; (a) APP-II, (b) peaks: (1) rhamnose, (2) arabinose, (3) xylose, (4) mannose, (5) glucose, (6) galactose, and (7) inositol. | |
In a previous study, Zhu et al. studied the structure of a polysaccharide from Astragalus (APS).38 Results showed that the average molecular weight of APS was 693 kDa, and the APS was α-pyranose. Zhu et al. also studied a polysaccharide from Paecilomyces sinensis (CPS).39 Those results indicated that CPS was a kind of glycopeptide with an average molecular weight of 200 kDa and represented an α-pyran type. CPS-II mainly contained xylose, mannose, glucose, and galactose with a molar ratio of 3.7
:
12.2
:
33
:
48. In this study, APP-II consisted of α-pyran polysaccharides with an average molecular weight of 670 kDa and is composed of rhamnose, arabinose, xylose, mannose, glucose, and galactose, with a molar ratio of 4.0
:
4.2
:
2.0
:
41.4
:
22.5
:
25.9. All the results indicated that the APP-II is a novel polysaccharide which is different from the polysaccharide of Astragalus and Paecilomyces sinensis.
Immunostimulatory activity of APP-I and APP-II in vitro
The effects of APP-I and APP-II on the proliferation of RAW264.7 cells is shown in Fig. 4; both APP-I and APP-II increased cell proliferation in concentrations from 25–400 μg mL−1 in a dose-dependent manner. These results suggested that APP-I and APP-II both could promote RAW264.7 cell proliferation and indicated that APP-I and APP-II had immunostimulatory activity in vitro. From previous results, the APP-II content in APP-I is 60.15% which directly indicates that the immunostimulatory activity of APP-I is caused by the APP-II in it.
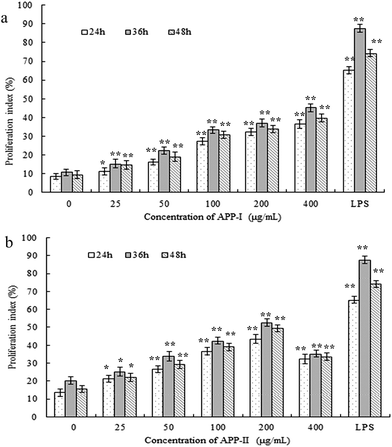 |
| Fig. 4 APP-I (a) and APP-II (b) increased the cell proliferation index of RAW264.7 cells. (*p < 0.05, **p < 0.01 vs. the control group). | |
Immunostimulatory activity of APP-II in vivo
Taking account of the low yield and efficiency of gel chromatography and the large dosage used in the animal experiments, APP-I (i.e., crude polysaccharides of AP) was used to study immunostimulatory activity in vivo.
Thymus and spleen indices. The thymus and spleen are the main immune organs of body. The thymus is the primary immune organ and has a relationship to cellular immunity. The spleen is a secondary immune organ and provides a place for immunocompetent cells to conduct further proliferation and differentiation. Thus, the thymus and spleen have close relations to humoral immunity and cellular immunity.Effects of APP-I on the thymus and spleen of mice were examined using immunological experiments and results are shown in Fig. 5a (thymic index) and Fig. 5b (splenetic index). Compared with the control group, all experimental groups had extremely significant differences in their thymic and splenetic indexes. With increasing dose, both the thymic and splenetic indexes improved which indicates that APP-I exerted significant promotion with both the thymic and splenetic indexes.
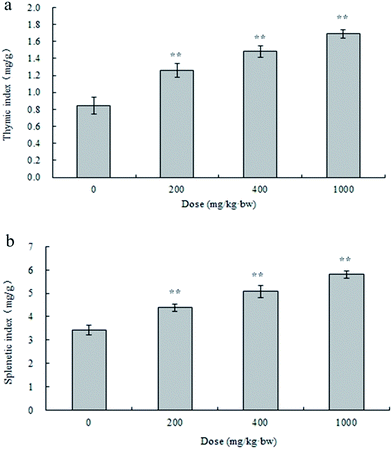 |
| Fig. 5 Effect of APP-I on thymic index (a), splenetic index (b) of mice. (*p < 0.05, **p < 0.01 vs. the control group). | |
Splenocyte proliferation. The effect of APP-I on proliferation of splenocyte also was examined. As a mitogen, concanavalin A (ConA) and lipopolysaccharide (LPS) can act on related receptors of T, B cell membrane, and activate cells to promote their proliferation. The stimulation indexes (SI) of ConA and LPS are shown in Fig. 6a and b, respectively. The experimental groups had extremely significant differences on stimulation indexes (SI) of ConA and LPS, compared with the control group. APP-I had a significant promotion for enhancing proliferation of splenocytes.
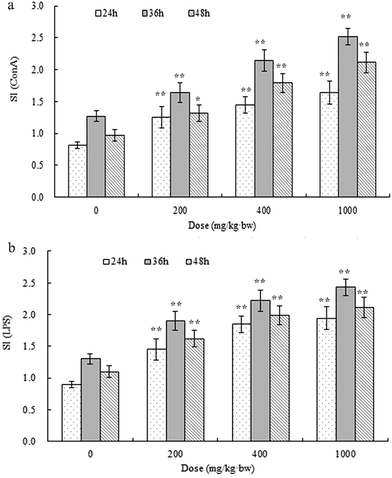 |
| Fig. 6 Effect of APP-I on splenocyte proliferation ((a) ConA stimulated, (b) LPS stimulated) of mice. (*p < 0.05, **p < 0.01 vs. the control group). | |
Assay of natural killer cell activity. NK activity is an important reference index for non-specific immune systems. It naturally exists as an important immune cell in a biosome and not only has a close relation to anti-tumor and anti-virus infection and immunity, but also participates in allergic reactions and autoimmune diseases. NK regulate T, B lymphocytes and bone marrow stem cells, and releases cytokines to modulate natural and specific immune responses.40 As shown in Fig. 7, the experimental groups had significant differences compared with the control group. NK activity increased with an increase in the dose concentrations.
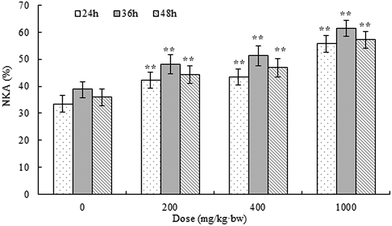 |
| Fig. 7 Effect of APP-I on NK cells activity of mice. (*p < 0.05, **p < 0.01 vs. the control group). | |
APP-I had extreme effects on thymic and splenetic indexes, splenocyte proliferation, and NK activity. All these results mean that APP-I can promote growth of immune organs, stimulate splenocyte proliferation, and enhance NK activity. Due to the large amount of APP-II in APP-I and the bioactivity of APP-II, the bioactivity of APP-I was caused by APP-II. Thus, this indicated that APP-II also had immunostimulatory activity in vivo.
All these results indicated that APP-II is α-pyran polysaccharide with an average molecular weight of 670 kDa and is composed of rhamnose, arabinose, xylose, mannose, glucose, and galactose, with molar a ratio of 4.0
:
4.2
:
2.0
:
41.4
:
22.5
:
25.9. The results of in vitro and in vivo experiments with immunostimulatory activity indicated that APP-II can enhance proliferation of RAW264.7 cells. It can also promote growth of immune organs, stimulate splenocyte proliferation, and enhance NK activity in mice. In previous literature, structure and immunostimulatory activity of polysaccharides from both Astragalus and Cordyceps sinensis were reported. Li et al. studied the characterization of Astragalus polysaccharides (from Astragalus roots) and its immune modulating activities in rats with gastric cancer.41 Those results indicated that the polysaccharide of Astragalus is an α-(1–4)-D-glucan with α-(1–6)-linked branches attached to O-6 of the branch points. Bioactivity tests showed that the polysaccharide of Astragalus administered dose-dependently (100, 200, and 300 mg kg−1 bw) significantly increased proliferation of spleen lymphocytes. The Astragalus polysaccharide administered dose-dependently (100, 200, 300, and 300 mg kg−1 bw) significantly increased blood IL-2 levels and NK activities. Shao et al. demonstrated that Astragalus polysaccharides (Rha
:
Xyl
:
Glc
:
Gal
:
Man
:
Fru = 4.9
:
4.7
:
8.3
:
122.2
:
2.2
:
3.1) activated mouse macrophages and B cells, rather than T cells, in terms of proliferation and cytokine production via the activation of Toll-like receptor 4 (TLR4).42 Wang et al. studied the Structural characterization and immunostimulatory activity of a polysaccharide from natural Cordyceps sinensis.43 The molecular weight was 9.76 × 105 Da. The structure was deduced to be a homogenous glucan, comprised a main chain of (1–4)-linked-α-D-Glcp with a single α-D-Glcp branch substituted at C-6 and this polysaccharide exhibited a significant stimulatory effect on RAW264.7 cells proliferation. In a concentration range of 50–200 μg mL−1, the proliferation rates of polysaccharide-treated groups were significantly higher than that of the positive control group. It was revealed that the polysaccharides can significantly stimulate the proliferation of macrophages. Wang et al. reported an acidic polysaccharide (AEPS-1) which was separated from Cordyceps sinensis Cs-HK1 in a mycelial culture.15 AEPS-1 was composed of glucopyranose (Glcp) and pyrano-glucuronic acid (GlcUp) in an 8
:
1 M ratio plus a trace amount of mannose, and had an average molecular weight of about 36 kDa. In Raw264.7 macrophage cell cultures, AEPS-1, at suitable doses between 25 μg mL−1 and 250 μg mL−1, significantly stimulated the release of several major cytokines, demonstrating an immunomodulatory property. Thus, APP-II is a novel polysaccharide with immunostimulatory activity, which is different from polysaccharides of Astragalus and Cordyceps sinensis.
Conclusion
An Astragalus residue was reutilized through solid-state fermentation by Paecilomyces sinensis, which is a member of Ophiocordyceps sinensis (Berk.) Sacc. In this research, pure polysaccharide from AP was primarily studied. First, HPLC, FT-IR, and GC were applied to study the preliminary characterization of a polysaccharide (APP-II) from AP. These results indicated that APP-II was an α-pyran polysaccharide with an average molecular weight of 670 kDa that is composed of rhamnose, arabinose, xylose, mannose, glucose, and galactose, with a molar ratio of 4.0
:
4.2
:
2.0
:
41.4
:
22.5
:
25.9. These results indicated that APP-II is a novel polysaccharide which is different from the polysaccharide of Astragalus and Paecilomyces sinensis. Second, immunostimulatory activity of APP-II was detected from in vivo and in vitro experiments. These results suggested that APP-I and APP-II could promote RAW264.7 cell proliferation, which indicated that APP-II had immunostimulatory activity in vitro. Then, APP-I was used to detect immunostimulatory activity of APP-II in vivo. These results indicated that APP-II can promote growth of immune organs, stimulate splenocyte proliferation, and enhance NK activity, which showed that APP-II has immunostimulatory activity in vivo. All the results indicated that APP-II is a novel polysaccharide with immunostimulatory activity which has significant importance to reutilization of AP.
Acknowledgements
This work was financially supported by the key program of the Natural Science Foundation of Tianjin (16JCZDJC34100), the National Spark Key Program of China (2015GA610001) and the International Science and Technology Cooperation Program of China (2013DFA31160).
References
- G. H. Chen and W. F. Huang, Chin. J. New Drugs, 2008, 17, 1482–1485 CAS.
- R. Z. Chen, L. Tan and C. G. Jin, Ind. Crops Prod., 2015, 77, 434–443 CrossRef CAS.
- H. X. Xue, F. Gan and Z. Q. Zhang, Int. J. Biol. Macromol., 2015, 81, 22–30 CrossRef CAS PubMed.
- Q. Y. Liu, Y. M. Yao and S. W. Zhang, J. Ethnopharmacol., 2011, 136, 457–464 CrossRef CAS PubMed.
- W. Wei, H. T. Xiao and W. R. Bao, J. Ethnopharmacol., 2016, 179, 243–252 CrossRef CAS PubMed.
- B. Yang, B. Xiao and T. Y. Sun, Int. J. Biol. Macromol., 2013, 62, 287–290 CrossRef CAS PubMed.
- W. Chen, Y. M. Li and M. H. Yu, J. Diabetes Complications, 2010, 24, 199–208 CrossRef PubMed.
- Y. Chen, M. Y. Song and Y. X. Wang, Carbohydr. Polym., 2015, 117, 339–345 CrossRef CAS PubMed.
- F. Yan, Q. Y. Zhang and L. Jiao, Phytomedicine, 2009, 16, 805–813 CrossRef CAS PubMed.
- X. F. Wang, J. Shen and S. Z. Li, Int. J. Biol. Macromol., 2014, 69, 146–150 CrossRef CAS PubMed.
- Z. Y. Zhu, L. N. Ding and Q. Yao, Food Res. Dev., 2012, 33, 171–173 CAS.
- H. Wu, H. Zhang and Y. F. Yang, J. Qinghai Univ., 2009, 27, 65–68 Search PubMed.
- G. H. Sung, L. Nigel and J. Hywel, Stud. Mycol., 2007, 57, 55–59 Search PubMed.
- L. Xiang, Y. Li and Y. J. Zhu, Genomics, 2014, 103, 154–159 CrossRef CAS PubMed.
- Z. M. Wang, X. Peng and K. L. D. Lee, Food Chem., 2011, 125, 637–643 CrossRef CAS.
- D. T. Wu, L. Z. Meng and L. Y. Wang, Carbohydr. Polym., 2014, 110, 405–414 CrossRef CAS PubMed.
- X. L. Yao, S. Meran and Y. P. Fang, Food Hydrocolloids, 2014, 35, 444–452 CrossRef CAS.
- J. L. Zhang, Y. C. Yu and Z. F. Zhang, Int. Immunopharmacol., 2011, 11, 2251–2257 CrossRef CAS PubMed.
- M. Wang, X. Y. Meng and R. L. Yang, Carbohydr. Polym., 2012, 89, 461–466 CrossRef CAS PubMed.
- N. Kazuki, S. Kazumasa and Y. Noriko, J. Pharm. Sci., 2015, 127, 53–56 CrossRef PubMed.
- T. B. Ng and H. X. Wang, J. Pharm. Pharmacol., 2005, 57, 1509–1519 CrossRef CAS PubMed.
- P. T. Li, Z. Q. Liu and D. Zhao, Inflammation, 2011, 34, 639–644 CrossRef PubMed.
- W. F. Chiou, P. C. Chang and C. J. Chou, Life Sci., 2000, 66, 1369–1376 CrossRef CAS PubMed.
- T. W. Balon, A. P. Jasman and J. Zhu, J. Altern. Complement. Med., 2002, 8, 315–323 CrossRef PubMed.
- J. H. Koh, J. M. Kim and U. J. Chang, Biol. Pharm. Bull., 2003, 26, 84–87 CAS.
- Z. Y. Zhu, Y. Li, H. Q. Sun, L. J. Chen, Y. L. Tang, X. C. Liu and Y. M. Zhang, Cellul. Chem. Technol., 2016, 50, 257–263 CAS.
- Z. Y. Zhu, C. L. Xiao, H. Q. Sun, L. C. Dai and H. Gao, J. Tianjin Univ. Sci. Technol., 2013, 28, 1–3 Search PubMed.
- M. F. Chaplin and J. F. Kennedy, Carbohydrate analysis. A practical approach, IRL Press, New York, Oxford, 1986, p. 3 Search PubMed.
- Z. Y. Zhu, C. L. Si, Y. R. Zhong, C. M. Zhu, J. P. Zhou and A. J. Liu, J. Food Biochem., 2011, 35, 303–322 CrossRef CAS.
- N. Alam and P. C. Gupta, Planta Med., 1986, 52, 308–310 CrossRef PubMed.
- E. Gómez-Ordónez, A. Jiménez-Escrig and P. Rupérez, Talanta, 2012, 93, 153–159 CrossRef PubMed.
- J. K. Yan, L. Li, Z. M. Wang and J. Y. Wu, Carbohydr. Polym., 2010, 79, 125–130 CrossRef CAS.
- G. H. Zhao, J. Q. Kan, Z. X. Li and Z. D. Chen, Carbohydr. Polym., 2005, 61, 125–131 CrossRef CAS.
- T. Mosmann, J. Immunol. Methods, 1983, 65, 55–63 CrossRef CAS PubMed.
- Z. M. Gu, Z. S. Ma and J. H. An, J. Jilin Univ., 2003, 29, 119–120 CAS.
- Y. C. Ning, Structural identification of organic compounds and organic spectroscopy, Science Press, 2000, pp. 332–335 Search PubMed.
- S. A. Barker, E. J. Bourne, M. Stacey and D. H. Whiffen, J. Chem. Soc., 1954, 1, 171–176 RSC.
- Z. Y. Zhu, Y. Luo, G. L. Dong, Y. Y. Ren, L. J. Chen, M. Z. Guo, X. T. Wang, X. Y. Yang and Y. M. Zhang, Int. J. Biol. Macromol., 2016, 87, 570–576 CrossRef CAS PubMed.
- Z. Y. Zhu, M. Meng, H. Q. Sun, Y. Li, N. Yu and Y. M. Zhang, Food Funct., 2016, 7, 1593–1600 CAS.
- K. Noriko and K. N. Tadahiro, Mycoscience, 2003, 44, 257–261 CrossRef.
- R. Li, W. C. Chen, W. P. Wang, W. Y. Tian and X. G. Zhang, Carbohydr. Polym., 2009, 78, 738–742 CrossRef CAS.
- B. M. Shao, W. Xu, H. Dai, P. F. Tu, Z. J. Li and X. M. Gao, Biochem. Biophys. Res. Commun., 2004, 320, 1103–1111 CrossRef CAS PubMed.
- J. Q. Wang, S. P. Nie, S. W. Cui, Z. J. Wang, A. O. Phillips, G. O. Phillips, Y. J. Li and M. Y. Xie, Food Hydrocolloids, 2017, 67, 139–147 CrossRef CAS.
|
This journal is © The Royal Society of Chemistry 2017 |