DOI:
10.1039/C6RA28514G
(Paper)
RSC Adv., 2017,
7, 23882-23889
Metallomic profiling to evaluate the response to drug treatment: hydroxyurea as a case study in β-thalassemia patients†
Received
21st December 2016
, Accepted 11th April 2017
First published on 3rd May 2017
Abstract
β-Thalassemia is the most common genetic disorder and results in the defective synthesis of β-globin chain, followed by chronic anemia. Hydroxyurea (HU) is among the drugs that effectively enhance fetal hemoglobin (HbF) levels in β-thalassemia patients, hence reducing blood transfusion requirements and transfusion-related complications. The current study focuses on the serum metallomic profiling of 40 β-thalassemia patients before and after treatment with hydroxyurea. Forty-five healthy individuals served as controls. For the profiling, 19 elements were analyzed by inductively coupled plasma-mass spectrometry (ICP-MS). The results showed significant differences in 15 elements at a probability level of 0.05 with a fold-change >3 between the HU-treated and untreated groups. Of these elements, 8 showed the same levels in thalassemic patients after administration of HU as in healthy controls. These results suggest that treatment with HU not only improves Hb levels in β-thalassemia patients but also reduces biometal dysregulations, thus improving the management of β-thalassemia.
Introduction
β-Thalassemia, a genetic autosomal recessive disease, is the most common type of chronic hemolytic anemia and is characterized by the reduction or complete absence of β-globin gene expression.1 The decrease in the production of β-globin chains affects multiple organs, and it is associated with considerable morbidity and mortality.2 Thalassemia major (TM) is the most severe form of the disease, resulting in transfusion-dependent anemia, generally in the first year of life. Thalassemia intermedia (TI), on the other hand, is a less severe form of the disease that also requires transfusion, although infrequently. In the past, therapy of thalassemia has been confined to transfusion, chelation (intravenous and oral), and antioxidants. Recent treatment strategies based on the pathophysiology and molecular pathology of thalassemia major include bone marrow transplantation in addition to gene therapy. Alternative treatments that cause an increase in the HbF levels are also used in the management of β-thalassemia.3
Treatment with hydroxyurea (HU) is one of the most attractive ways to manage the severity of hemolysis of thalassemic RBCs by increasing the rate of γ-globin chain synthesis such that RBCs will contain more fetal Hb4 and less excess α-globin chains, which is the primary factor leading to hemolysis, ultimately resulting in a decreased requirement for transfusions.5,6 Although HU is an alkylating agent commonly used for the treatment of myeloproliferative diseases such as chronic myeloid leukemia and polycythemia vera, it is also currently used for HbF induction with a high rate of success.7,8 Despite the beneficial effects of HU on erythropoiesis, the exact mechanism of action of HU is not entirely clear.9 Moreover, hematological, neurological, dermatological and gastrointestinal adverse effects of HU were reported in β-thalassemia and sickle cell anemia patients.10–12
The metallomics approach primarily focuses on the detection of elemental markers for the diagnosis of diseases. Elements play a vital role in biological systems through their interactions with biomolecules.13,14 Encouraging results were obtained using elemental analysis to the diagnosis and understanding of the disease status in conditions including hemochromatosis,15 lung disease,16 chronic kidney disease,17 renal failure,18 cardiovascular disease,19 liver disease,20 and various neurological and psychological disorders.21
In previous studies, the analyses of zinc, iron, copper, magnesium and selenium levels in plasma, erythrocytes, hair and urine samples of β-thalassemia patients by atomic absorption spectrophotometry were reported.22–24 The changes observed were indicative of the abnormal levels of elements in β-thalassemia patients. An abnormal elemental distribution in individuals with β-thalassemia, and their elemental levels after using different iron chelators such as deferoxamine, deferiprone, and deferasirox have also been reported.25–27 However, to date, no studies on the patterns of trace and ultra-trace elements in β-thalassemia patients after treatment with hydroxyurea have been reported. In this study, an extensive analysis of 19 elements was conducted by inductively coupled plasma-mass spectrometry (ICP-MS). The analysis was conducted on the serum samples of 45 healthy volunteers and 40 follow-up HU-treated β-thalassemia patients, to investigate the differences in the concentrations of aluminum (Al), barium (Ba), cadmium (Cd), calcium (Ca), chromium (Cr), cobalt (Co), copper (Cu), iron (Fe), lead (Pb), lithium (Li), magnesium (Mg), manganese (Mn), nickel (Ni), rubidium (Rb), selenium (Se), silver (Ag), strontium (Sr), vanadium (V), and zinc (Zn). The metallomic profiling in the current study was conducted to better understand the overall effect of treatment with HU on elemental dysregulations and abnormal elemental distribution in β-thalassemia patients, in addition to the previously reported effects of HU on HbF induction.
Materials and methods
Reagents and standards
All chemicals used in the study were of analytical grade and checked for possible contamination with trace metals before use. Ultrapure filtered deionized water (resistivity of 18.2 MΩ cm at 25 °C) obtained with a Barnstead MicroPure Water Purification System (Thermo Scientific, USA) was used for sample preparation and dilutions. Analytical grade nitric acid was purified on a NanoPure-1000 Acid Purification System (Nanonex, USA). A multi-element stock standard solution containing 10 μg mL−1 of each element was purchased from Agilent Technologies (CA, USA) and used to prepare mixed calibration standards. Mixed calibration working standards (with concentrations ranging from 0.1–200 μg L−1 for all elements) were prepared daily by dilution of the analytical standards and their stock solutions. An internal standard solution containing 100 μg mL−1 of Bi, Ge, Ir, Lu, Rh, Sc, and Tb elements was purchased from Agilent Technologies (CA, USA). Prior to use, all containers were soaked in 5% v/v HNO3 for 24 hours, followed by rinsing 3 times with ultrapure deionized water and dried in a laminar-flow hood (Airstream® ESCO, Singapore). All operations were performed on a clean bench.
Sample collection
Blood samples were collected from the National Institute of Blood Diseases and Bone Marrow Transplantation (NIBD), Pakistan, after written informed consent of the participants, in accordance with the ethical standards as outlined in the declaration of Helsinki. The study was approved by the Institutional Review Board (IRB)/Ethics Committee of the hospital as per ICH GCP guidelines as well as by the Independent Ethics Committee (IEC) of the main research institute. All experiments were performed in compliance with the relevant laws and institutional guidelines. For the current study, a total of 125 serum samples were collected from both male and female participants. Herein, 45 samples were collected from healthy subjects, 40 samples were collected from β-thalassemia patients before treatment with HU, and the remaining 40 after treatment with HU. All patients were subjected to HU treatment for about 6–12 months prior to the collection of the second sample. The details of patients and healthy controls are shown in Table S1.† After treatment with HU, patients were categorized into three groups based on their response: good responders (GRs), partial responders (PRs), and non-responders (NRs). The group of patients referred to as good responders were initially on regular blood transfusion and upon HU treatment achieved a steady Hb level >7 g dL−1 without any need for blood transfusion. Similarly, partial responders were patients who showed a 50% reduction in the need for blood transfusion after HU treatment, while non-responders showed less than 50% reduction in the need for blood transfusion.
A blood volume of about 5 mL was obtained by venipuncture from each subject and transferred into trace metal-free evacuated gel-based BD® vacutainer tubes (BD Franklin Lakes NJ, USA, REF: 367381) that contained silicon for clot activation. The samples were collected under fasting conditions (minimum 6–8 h) from patients that were not receiving any other medication. The serum was separated immediately by centrifugation at 2000 rpm for 10 min at 4 °C. Finally, a total of 150 μL of serum sample was aliquoted, coded, and immediately stored at −80 °C until processed.
Sample preparation
Serum samples were digested in a high-performance sealed-pressure microwave system (Anton Paar, Graz, Austria) equipped with a 64 MG 5 rotor. Vessels were equipped with a pressure release system. Disposable standard glassware (Wheaton® 15 × 45 mm, cap 13-425) along with a PEEK screw cap with a disposable PTFE lip seal were used. The system was operated (software version 1.51) at 200 W power at a temperature of 200 °C. This 200 watt stage was pulsed to deliver the microwave energy for 7 min and then paused for 9 min.
The digestion of samples was performed by taking a 200 μL aliquot from a serum sample, to which 1 mL of 65% nitric acid was added. After digestion, all samples were allowed to cool at room temperature. Subsequently, the digested samples were transferred into 15 mL polypropylene autosampler tubes, rinsed with ultrapure deionized water three times, and then made up to a final volume of 5 mL. Each sample was also diluted (50% v/v) with deionized water prior to analysis. All the samples were analyzed in triplicates for Li, Mg, Al, Ca, V, Cr, Mn, Fe, Co, Ni, Cu, Zn, Se, Rb, Sr, Ag, Cd, Ba, and Pb metals. For matrix correction, an internal standard containing Bi, Ge, Ir, Lu, Rh, Sc, and Tb was used to validate the ICP-MS results.
Preparation of the standard reference material
In order to verify the accuracy and precision of the proposed method, a standard reference material, Seronorm™ Trace elements serum L-1 was purchased from Sero (Billingstad, Norway). To prepare the standard reference sample, in a vial, 3 mL of ultrapure deionized water was added to it, and the vial contents were completely dissolved by continuous rolling for about 30 min. The entire procedure was conducted as per the manufacturer's protocol. The control material was further diluted with ultrapure deionized water and quantitatively transferred into polypropylene autosampler tubes. Each trace and ultra-trace element was analyzed in triplicate in the diluted control material by ICP-MS.
ICP-MS analysis
Concentrations of different elements in serum were determined using a 7700x ICP-MS system (Agilent Technologies, Santa Clara, CA USA) equipped with a 27 MHz RF generator. The sample introduction system consisted of a low-flow concentric nebulizer connected to a temperature-controlled Scott-type quartz spray chamber with a double-pass, a high-precision 10-roller peristaltic pump with Tygon pump tubing (internal diameter 1.02 mm), and a ASX-520 autosampler. A nickel sampler and skimmer cones (orifice diameter 1.0 mm and 0.4 mm) were used. All components were optimized for high-throughput routine analysis of samples with TDS up to 0.2%. Two rinsing solutions containing 2% and 5% nitric acid were used to ensure sample washout between analyses. Data were processed with the ICP-MS MassHunter Workstation software. The operation parameters for the 7700x ICP-MS instrument are summarized in Table 1. For data collection, the quadrupole mass analyzer was operated in the multiple-ion monitoring mode. The concentration of the analyte was calculated by integrating the corresponding chromatogram peak areas with the MassHunter software.
Table 1 Agilent 7700x ICP-MS operating parameters
Component |
Parameter |
Spray chamber |
Scott-type double-pass |
Nebulizer |
MicroMist (concentric) |
Interface |
Ni cones |
RF power |
1600 W |
Carrier gas |
1.0 L min−1 |
Spray chamber temperature |
2 °C |
Plasma gas flow rate |
15 L min−1 |
Nebulizer gas flow rate |
1.1 L min−1 |
Auxiliary gas flow rate |
0.36 L min−1 |
Sample uptake rate |
0.8 mL min−1 |
Integration time |
3 ms |
Acquisition time |
60 s |
Statistical analysis
Statistical analyses were performed using the Agilent Mass Profiler Professional (MPP) software (version 12.05) for multistep processing. Data was filtered using a minimum absolute abundance of 10
000 counts. An external scalar was used to normalize the data. The Z transform was selected as the baseline option, treating all the entities equally irrespective of their intensity. Statistical significance was determined by the one-way analysis of variance (ANOVA) test. Asymptotic p-value computation and Benjamini–Hochberg FDR with a fold-change of 3.0 and p < 0.05 for multiple testing corrections were used to compare the three groups: healthy subjects as the control group, untreated β-thalassemia patients, and HU-treated β-thalassemia patients. Clustering analysis was performed by applying the hierarchical clustering algorithm to normalized intensity values. Clustering was done by the complete linkage method with the Canberra distance metric. A partial least square discriminant analysis (PLS-DA) model and its three-dimensional principle component analysis (PCA) model was generated for healthy subjects, HU-treated and untreated β-thalassemia patients using pareto scaling and n-fold validation with three folds and ten repeats. A confusion matrix model was then generated to check the accuracy of the experiment. A plot with the normalized intensity values was constructed with Microsoft Excel (version 2013) to determine the amount of each element in all groups. Box-and-whisker plots were generated with GraphPad Prism (version 7.0) for interpreting data distribution.
Results
Method optimization and validation
In order to obtain a linear calibration curve, the concentration range of 0.1–200 μg L−1 was selected for Li, V, Cr, Mn, Fe, Co, Ni, Cu, Zn, Rb, Ag, Cd, and Pb, while a concentration range of 25–200 μg L−1 was selected for Mg, Al, Ca, Se, Sr and Ba. The counts per second (cps) data plotted against the corresponding concentrations was analysed by the least-square regression method. The calibration curves (n = 3) showed a good linear relationship, with correlation coefficients (R2) between 0.99633 and 0.99996. The correlation coefficients and LOD values are shown in Table S2.† These validation parameters were found to be better for various elements in our study compared to a few previous studies related to analysis of elements in blood.28,29
The proposed method was validated for accuracy and precision using the standard reference material, Seronorm™ Trace elements serum L-1 and results are shown in Table S3.† All values were within an acceptable range. No statistically significant differences at the 95% confidence level were found between the target levels and the levels measured. This demonstrated the accuracy of the proposed method. Moreover, the between and within-batch precision for all the elements analyzed were lower than 10% and 5%, respectively.
Multivariate analysis of serum samples
The serum metallomic profile of 40 β-thalassemia patients before treatment was compared with their metallomic profile after treatment with hydroxyurea, and 45 samples of healthy volunteers were used as control. Thus, a total of 125 serum samples were analyzed by ICP-MS.
Significance testing and fold-change
Significance testing and fold-change was conducted on a total of 19 elements. From these, 15 elements were found to show significant differences at a probability level of 0.05 with a fold-change >3. Some metals showed higher levels while others showed lower levels in β-thalassemia patients as compared to HU-treated patients and healthy subjects. Briefly, 11 and 7 elements presented lower levels, while 4 and 8 presented higher levels, in HU-treated and healthy subjects, respectively, compared to patients prior to HU treatment (Table 2). The difference in the pattern of significantly differentiated elements in β-thalassemia patients before and after HU treatment and healthy subjects is shown in Fig. 1, plotted as average normalized intensity values. It is quite clear that after HU treatment, the metallomic pattern becomes closer to that of healthy controls.
Table 2 List of elements present in lower levels and higher levels in healthy controls and HU-treated β-thalassemia patients against untreated β-thalassemia patients
Element |
p (Corr) |
log FC (healthy vs. untreated) |
Regulation (healthy vs. untreated) |
log FC (treated vs. untreated) |
Regulation (treated vs. untreated) |
7Li |
5.24 × 10−7 |
0.51836795 |
Up |
−0.667174 |
Down |
24Mg |
1.70 × 10−5 |
0.096624464 |
Up |
−0.8711834 |
Down |
27Al |
0.002786 |
0.5870697 |
Up |
−0.14028192 |
Down |
51V |
1.92 × 10−12 |
−1.3574451 |
Down |
−1.2973746 |
Down |
52Cr |
4.63 × 10−9 |
−1.027559 |
Down |
−1.283315 |
Down |
56Fe |
2.10 × 10−16 |
−1.2139559 |
Down |
0.40955657 |
Up |
59Co |
5.36 × 10−8 |
−1.2397563 |
Down |
−0.5659481 |
Down |
60Ni |
0 |
2.1284356 |
Up |
1.6532539 |
Up |
63Cu |
0.002297 |
−0.7095547 |
Down |
−0.66768885 |
Down |
66Zn |
3.28 × 10−7 |
0.32862908 |
Up |
−0.851271 |
Down |
78Se |
6.16 × 10−5 |
−0.70982546 |
Down |
0.2272544 |
Up |
85Rb |
0.029813 |
0.50035113 |
Up |
0.56463945 |
Up |
107Ag |
2.23 × 10−8 |
0.8216013 |
Up |
−0.44884703 |
Down |
111Cd |
1.02 × 10−5 |
0.69791025 |
Up |
−0.3461811 |
Down |
208Pb |
1.12 × 10−4 |
−0.3597311 |
Down |
−0.9692022 |
Down |
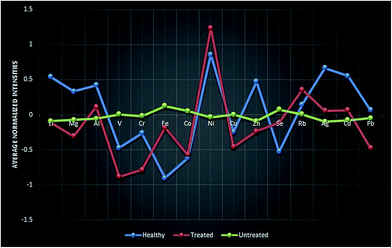 |
| Fig. 1 Average normalized intensity values of healthy controls, HU-treated and untreated β-thalassemia patients. | |
Cluster analysis
For cluster analysis, a dendrogram was generated by taking the average normalized intensity values of the 15 elements that were significantly different between healthy controls and β-thalassemia patients before and after HU treatment. The three groups were clustered into two classes (Fig. 2). When the samples for HU-treated and healthy subjects were clustered, a dissimilarity of 11.05 was obtained, whereas a dissimilarity of 13.36 was found upon clustering of the samples of healthy, HU-treated and untreated subjects. This indicated that the metallomic profile of patients before receiving HU treatment was the most dissimilar of all.
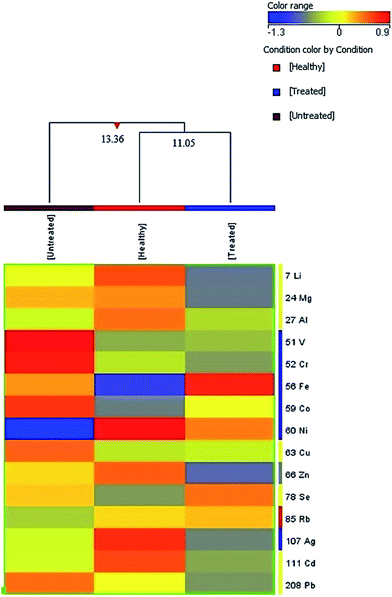 |
| Fig. 2 Dendrogram expressing the normalized intensity values of healthy controls, and untreated and HU-treated β-thalassemia patients. | |
Cluster analysis was also performed on five groups including healthy controls, untreated β-thalassemia patients and three HU-treated groups: good responders (GRs), partial responders (PRs) and non-responders (NRs). The five groups were clustered into four classes (Fig. S1†). PRs, and NRs were clustered in class I, with the least dissimilarity (of 3.72), while the three groups including GRs, PRs and NRs were clustered in class II, with a dissimilarity level of 6.59. In class III, four groups were clustered: healthy subjects, GRs, PRs and NRs, with a dissimilarity level of 11.26. All five groups (healthy controls, untreated patients, GRs, PRs and NRs) were clustered in class IV, revealing the highest dissimilarity level, of 12.36, indicating that the metallomic profile of patients before HU treatment was again the most dissimilar of all, including healthy sujects and all three groups of HU-treated patients.
Class prediction model
For the class prediction model, a confusion matrix was generated, which gives an accuracy of the prediction for each class. The overall prediction accuracy for the groups was found to be 97.391%, as presented in Table 3. The plots obtained by PLS-DA scores are shown in Fig. 3 and 4, showing a remarkable separation trend between healthy, HU-treated, and untreated groups. Most of the samples from HU-treated patients were so similar to those of healthy subjects that the metallome of the samples of HU-treated patients may be considered normal. On the other hand, very few of the samples from HU-treated patients were found to be closer to those of untreated patients.
Table 3 Confusion matrix of the model generated from untreated β-thalassemia patients (n = 40), HU-treated β-thalassemia patients (n = 40), and healthy controls (n = 45)
|
Healthy predicted |
Treated predicted |
Untreated predicted |
Accuracy |
True healthy |
40 |
2 |
0 |
95.238 |
True treated |
0 |
35 |
1 |
97.222 |
True untreated |
0 |
0 |
37 |
100.000 |
Overall accuracy |
|
|
|
97.391 |
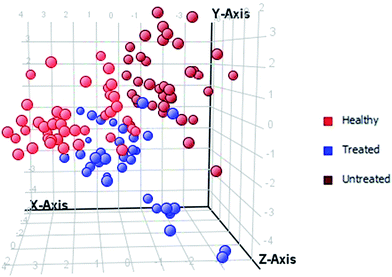 |
| Fig. 3 PCA score plot of healthy controls, and HU-treated and untreated β-thalassemia patients based on 15 significantly differentiated elements, with a fold-change >3. | |
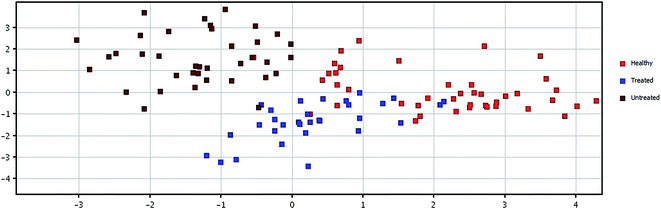 |
| Fig. 4 PLS-DA score scatter plot discriminating among healthy controls, HU-treated and untreated β-thalassemia patients based on 15 significantly differentiated elements, with a fold-change >3. | |
Statistical summary about concentration ranges
The concentration ranges of the analyzed elements in the serum of healthy subjects, and HU-treated and untreated patients with β-thalassemia, which were further classified as GRs, PRs, and NRs, were also evaluated. The concentration ranges of all selected elements, namely, Li, Mg, Al, Ca, V, Cr, Mn, Fe, Co, Ni, Cu, Zn, Se, Rb, Sr, Ag, Cd, Ba, and Pb, in all 5 groups, represented by box and whisker plots (Fig. 5), showed the complete picture of each element at ppb levels. Significant variations were observed in 15 elements, namely Li, Mg, Al, V, Cr, Fe, Co, Ni, Cu, Zn, Se, Rb, Ag, Cd, and Pb, whereas Ca, Mn, Sr, and Ba showed no change among the 5 different groups of serum samples.
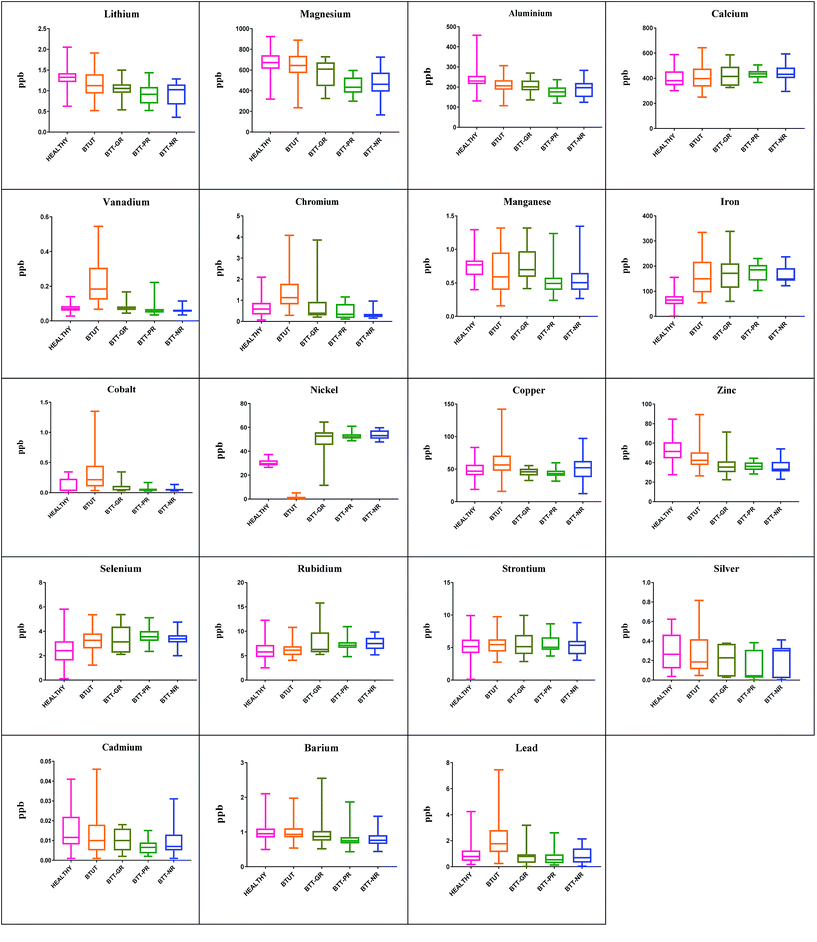 |
| Fig. 5 Graphical representation in box and whisker plots of concentration ranges of all selected metals in healthy controls, HU untreated β-thalassemia patients (BTUT), and GR, PR and NR HU-treated β-thalassemia patients. | |
Discussion
As a result of frequent blood transfusions, β-thalassemia patients often present altered levels of various elements and, most commonly, iron levels are elevated. HU provides the additional benefit of iron chelation, along with HbF induction. It is also reported that HU, when used in combination with other chelators, shows the maximum chelation effect.30,31
The metallomic profile of serum showed that 8 elements, including V, Cr, Fe, Co, Ni, Cu, Rb, and Pb have differential distribution when compared with HU untreated samples. It was found that HU treatment led to a pattern analogous to that of healthy controls. A shifting of the metallomic profile of these distinct elements towards healthy controls was clearly seen in the box and whisker plots (Fig. 5).
Copper is an essential mineral for cell function with a key role as an antioxidant.25 There were contradictory results in the literature about copper in β-thalassemia patients: some studies claimed that thalassemia patients had elevated levels of serum copper,32 whereas others reported copper deficiency in some of the patients.33,34 In our study, copper levels were found to be significantly increased in untreated thalassemia patients as compared to healthy controls. By contrast, HU-treated patients showed a significant decrease in copper concentrations. The levels of copper in the metallomic profile of HU-treated patients were found to be similar to those normally found in healthy individuals. These results indirectly support the lower oxidative stress in HU-treated patients as the copper levels return to normal.
Elements such as vanadium, chromium, cobalt, lead, nickel and rubidium are found in ultra-trace levels in the human body. Except Rb, they all are toxic. The concentrations of V, Cr, Co, and Pb were found to be significantly elevated in thalassemia patients before HU treatment as compared to those of healthy controls. However, their levels decreased after HU treatment. According to evidence reported in the literature, excess vanadium can cause biochemical imbalances in the body, resulting in body aches, arthritis, a weakened immune system, gastrointestinal disorders and various symptoms. Lead toxicity can cause anemia, brain damage, kidney disease, impaired growth, impaired reproductive function, and mental retardation in children. The concentrations of Ni, and Rb were found to be lower in untreated β-thalassemia patients as compared to healthy controls. However, their levels were found to be increased in HU-treated patients as compared to those of healthy and untreated subjects.
Zinc is an essential trace element. Its deficiency results in growth retardation, hypogonadism in males, skin changes, and delayed wound healing. These clinical signs are seen in severe thalassemia.35 In this study, zinc levels were found to be significantly lower in the samples of β-thalassemia patients, and even lower in those of HU-treated patients as compared to healthy controls. Selenium is a component of selenoproteins, such as glutathione peroxidase (GPx) and thioredoxine reductase (TrxR).36 There are contradictory reports in the literature about selenium in thalassemia patients.37,38 However, in this study selenium levels were found to be significantly higher in the samples of β-thalassemia patients, and even higher in those of HU-treated patients as compared to healthy controls.
Iron is an essential nutrient for the growth and proliferation of normal, neoplasmic and microbial cells. In this study, as expected, iron levels were higher in untreated thalassemic patients as compared to healthy controls due to regular blood transfusions, which leads to iron overload in the body.39 Iron accumulation in the liver is usually quite marked, and ferritin, an iron storage protein, can reach very high concentrations.40 In our study, no significant changes in iron levels were found in HU-treated patients.
Hierarchical clustering showed that the dissimilarity level between healthy subjects and HU-treated patients was lower than that between healthy subjects and untreated patients, indicating that the metallomic profile of patients before HU treatment was the most dissimilar of all. Cluster analysis was also performed on five groups including healthy controls, untreated, and HU-treated GR, PR, and NR groups. However, the metallomic profile was not different among the three HU-treated groups: GRs, PRs, and NRs.
Conclusions
A simple and high-throughput method for the determination of 19 biologically important elements in serum of HU treated β-thalassemia patients by inductively coupled plasma-mass spectrometry (ICP-MS) has been developed. The levels of 15 elements were found to be significantly altered in β-thalassemia patients before HU treatment, compared to healthy controls and after HU treatment patients' group. Furthermore, the metallomic profile of 8 distinct elements including chromium (Cr), cobalt (Co), copper (Cu), iron (Fe), lead (Pb), nickel (Ni), rubidium (Rb), and vanadium (V) in HU-treated patients with β-thalassemia was found to be identical to that of healthy subjects. The pattern observed indicated elevated levels of Ni, and Rb after HU treatment. In addition, metals such as V, Cr, Fe, Co, Cu, and Pb were found in lower levels in β-thalassemia patients after HU treatment. This preliminary study will make physicians aware of the consequences of the treatment with hydroxyurea in β-thalassemia patients, as it can also cause changes in the metallomic profile. The results suggest that HU not only improves Hb levels, but also reduces metal toxicity in β-thalassemia patients.
Acknowledgements
The authors acknowledge the volunteers for their participation in the study. The authors are also thankful to Mr Arsalan Tahir for technical assistance in ICP-MS analyses.
References
- R. M. Shawky and T. M. Kamal, Egypt. J. Med. Hum. Genet., 2012, 13, 245–255 CrossRef CAS.
- M. J. Cunningham, E. A. Macklin, E. J. Neufeld and A. R. Cohen, The Thalassemia Clinical Research, Blood, 2004, 104, 34–39 CrossRef CAS PubMed.
- D. Rund and E. Rachmilewitz, Critical Reviews in Oncology/Hematology, 2000, 33, 105–118 CrossRef CAS PubMed.
- K. M. Musallam, A. T. Taher, M. D. Cappellini and V. G. Sankaran, Blood, 2013, 121, 2199–2212 CrossRef CAS PubMed ; quiz 2372.
- M. Bradai, M. T. Abad, S. Pissard, F. Lamraoui, L. Skopinski and M. de Montalembert, Blood, 2003, 102, 1529–1530 CrossRef CAS PubMed.
- C. Borgna-Pignatti, Br. J. Haematol., 2007, 138, 291–304 CrossRef CAS PubMed.
- A. Koren, C. Levin, O. Dgany, T. Kransnov, R. Elhasid, L. Zalman, H. Palmor and H. Tamary, Am. J. Hematol., 2008, 83, 366–370 CrossRef CAS PubMed.
- G. F. Atweh and D. Loukopoulos, Semin. Hematol., 2001, 38, 367–373 CrossRef CAS PubMed.
- H. Cario, M. Wegener, K. M. Debatin and E. Kohne, Ann. Hematol., 2002, 81, 478–482 CrossRef CAS PubMed.
- M. Karimi, N. Cohan, K. Mousavizadeh, M. J. Falahi and S. Haghpanah, Pediatr. Hematol. Oncol., 2010, 27, 205–211 CrossRef CAS PubMed.
- A. Ghasemi, B. Keikhaei and R. Ghodsi, Iranian Journal of Pediatric Hematology and Oncology, 2014, 4, 114–117 CAS.
- M. Kosaryan, M. Zafari, A. Alipur and A. Hedayatizadeh-Omran, Hemoglobin, 2014, 38, 262–271 CrossRef CAS PubMed.
- S. S. Ahmed and W. Santosh, PLoS One, 2010, 5, e11252 Search PubMed.
- S. Mounicou, J. Szpunar and R. Lobinski, Chem. Soc. Rev., 2009, 38, 1119–1138 RSC.
- T. A. Hagve, A. Asberg, R. Ulvik, B. Borch-Iohnsen and K. Thorstensen, Tidsskr. Nor. Laegeforen., 2009, 129, 863–866 CrossRef PubMed.
- W. Koichi and K. Masayoshi, Biomed. Res. Trace Elem., 2003, 14, 275–278 Search PubMed.
- I. G. Nikolov, A. Mozar, T. B. Drueke and Z. A. Massy, Blood Purif., 2009, 27, 350–359 CrossRef CAS PubMed.
- C. I. Hur, T. R. Yoon, S. G. Cho, E. K. Song and J. K. Seon, Clin. Orthop. Relat. Res., 2008, 466, 696–699 CrossRef PubMed.
- W. Park, B. S. Kim, J. E. Lee, J. K. Huh, B. J. Kim, K. C. Sung, J. H. Kang, M. H. Lee, J. R. Park, E. J. Rhee, K. W. Oh, W. Y. Lee, C. Y. Park, S. W. Park and S. W. Kim, Diabetes Res. Clin. Pract., 2009, 83, 119–125 CrossRef CAS PubMed.
- D. D. Harrison-Findik, World J. Gastroenterol., 2009, 15, 1186–1193 CrossRef CAS PubMed.
- S. Pfaender and A. M. Grabrucker, Metallomics, 2014, 6, 960–977 RSC.
- U. Dogru, A. Arcasoy and A. O. Cavdar, Acta Haematol., 1979, 62, 41–44 CrossRef CAS.
- A. Arcasoy, D. Canata, B. Sinav, L. Kutlay, N. Oguz and M. Sen, J. Trace Elem. Med. Biol., 2001, 15, 85–87 CAS.
- A. Mahyar, P. Ayazi, A. A. Pahlevan, H. Mojabi, M. R. Sehhat and A. Javadi, Iranian Journal of Pediatrics, 2010, 20, 297–302 Search PubMed.
- G. E. Genc, Z. Ozturk, S. Gumuslu and A. Kupesiz, Biol. Trace Elem. Res., 2016, 170, 9–16 CrossRef CAS PubMed.
- K. Mansi, T. Aburjuri, M. Barqawi and H. Naser, Curr. Res. J. Biol. Sci., 2009, 4, 566–572 Search PubMed.
- E. Erdogan, D. Canatan, A. R. Ormeci, H. Vural and F. Aylak, J. Trace Elem. Med. Biol., 2013, 27, 109–111 CAS.
- A. Cesbron, E. Saussereau, L. Mahieu, I. Couland, M. Guerbet and J. P. Goulle, J. Anal. Toxicol., 2013, 37, 401–405 CrossRef CAS PubMed.
- J. P. Goulle, L. Mahieu, J. Castermant, N. Neveu, L. Bonneau, G. Laine, D. Bouige and C. Lacroix, Forensic Sci. Int., 2005, 153, 39–44 CrossRef CAS PubMed.
- K. Italia, R. Colah and K. Ghosh, PLoS One, 2013, 8, e82928 Search PubMed.
- P. J. Giardina and R. W. Grady, Semin. Hematol., 2001, 38, 360–366 CrossRef CAS PubMed.
- A. H. Al-Samarrai, M. H. Adaay, K. Al-Tikriti and M. M. Al-Anzy, Saudi Medical Journal, 2008, 29, 94–97 Search PubMed.
- M. A. Mashhadi, International Journal of Hematology-Oncology and Stem Cell Research, 2013, 7, 21–24 Search PubMed.
- R. A. Ghone, K. M. Kumbar, A. N. Suryakar, R. V. Katkam and N. G. Joshi, Indian J. Clin. Biochem., 2008, 23, 337–340 CrossRef CAS PubMed.
- S. Kajanachumpol, T. Tatu, W. Sasanakul, A. Chuansumrit and P. Hathirat, Southeast Asian J. Trop. Med. Public Health, 1997, 28, 877–880 CAS.
- L. M. Sherief, S. M. Abd El-Salam, N. M. Kamal, O. El Safy, M. A. Almalky, S. F. Azab, H. M. Morsy and A. F. Gharieb, BioMed Res. Int., 2014 DOI:10.1155/2014/261761.
- T. R. Shuler, P. Pootrakul, P. YarnSukon and F. H. Nielsen, J. Trace Elem. Exp. Med., 1990, 3, 31–43 Search PubMed.
- S. Claster, J. C. Wood, L. Noetzli, S. M. Carson, T. C. Hofstra, R. Khanna and T. D. Coates, Am. J. Hematol., 2009, 84, 344–348 CrossRef CAS PubMed.
- D. I. Zafeiriou, M. Economou and M. Athanasiou-Metaxa, Brain Dev., 2006, 28, 477–481 CrossRef PubMed.
- E. B. Fung, Ann. N. Y. Acad. Sci., 2010, 1202, 188–196 CrossRef PubMed.
Footnote |
† Electronic supplementary information (ESI) available. See DOI: 10.1039/c6ra28514g |
|
This journal is © The Royal Society of Chemistry 2017 |