DOI:
10.1039/C7RA00786H
(Paper)
RSC Adv., 2017,
7, 19486-19491
Characterization of Dietzia cercidiphylli C-1 isolated from extra-heavy oil contaminated soil†
Received
18th January 2017
, Accepted 25th March 2017
First published on 3rd April 2017
Abstract
Bioremediation of extra-heavy oil contaminated soil has become a hot research field. It is difficult for extra-heavy oil to be degraded by bacteria. In this study, strain C-1 was isolated from Venezuelan extra-heavy oil-contaminated soil. Morphological, physiological and phylogenetic analysis identified strain C-1 as Dietzia cercidiphylli. The strain could degrade 25.58 ± 0.48% of extra-heavy oil at an initial concentration of 100 mg L−1 in 14 days with the degradation rate constant of 0.021 per day. GC-MS analysis demonstrated that the strain could effectively degrade medium (C13–C17) and longer (C44) length chain n-alkanes. This is the first report that the Dietzia strain can degrade extra-heavy oil, especially C44 n-alkanes. Dietzia cercidiphylli C-1 could be applied in extra-heavy oil contaminated soil bioremediation in the future.
1. Introduction
Venezuelan extra-heavy oil is the largest accumulation of heavy oil in the world, containing 90% of the world's extra-heavy oil.1 The study and use of Venezuelan extra-heavy oil is rapidly growing to meet the increasing demand for energy throughout the world.2,3 Subsequently, extra-heavy oil enters into the soil environment via many ways, including processing, transportation, and storage as well as leakage. Compared to conventional crude oil and heavy oil, extra-heavy oil has a much higher density, viscosity and content of high molecular weight hydrocarbons.4 Obviously, extra-heavy oil may cause serious environmental damages and is extremely difficult to clean up. Remediation methods are urgently needed to address extra-heavy oil pollution.
Bioremediation technology has been recognized as an efficient, economic, and versatile alternative to physicochemical treatment of oil contaminated soil. The successful application bioremediation techniques including composting, bioaugmentation and biostimulation for remediating oil contaminated soil has been reported by numerous studies.5–7 It employs microorganisms to achieve complete or partial mineralization of pollutants, resulting in their removal or transformation to a harmless product.5,8 A large number of studies showed that many kinds of microorganisms had strong ability to degrade oil.9–14 Oil-degrading microorganisms have been widely considered to be the most crucial factors governing the bioremediation of oil contaminated soil. However, most of these studies focused on conventional crude oil, which is more readily degradable and easier to clean up than extra-heavy oil. There have been only a few attempts to isolate microorganisms that degrade extra-heavy oil and to use them for bioremediation of the contaminated soil environment.
It is important to obtain microorganisms that can degrade extra-heavy oil. Dietzia sp. has already been found to be capable of utilizing a wide range of n-alkanes. For example, Dietzia sp. A14101 depletes C6 to C26 n-alkanes of crude oil;15 Dietzia sp. E1 has an excellent ability to degrade C12 to C38 n-alkanes,16 Dietzia sp. DQ12-45-1b grows on a wide range of n-alkanes (C6–C40), aromatic compounds and conventional crude oil.17 Unfortunately, it is no report that Dietzia strains can degrade extra-heavy oil.
Here, a Dietzia strain was isolated from the Venezuelan extra-heavy oil contaminated soil in Liaohe oilfield (Liaoning, China), identified based on their morphological and physiological characteristics and 16S rDNA sequence analysis. As the bacterial strain showed high degradation capacity for extra-heavy oil, its degradation kinetics and degradation characteristics of extra-heavy oil were analyzed.
2. Materials and methods
2.1 Sampling
A soil sample was collected from the oil contaminated soil in Liaohe oilfield (Liaoning, China). Soil (500 g) was taken from 1 to 12 cm below the surface of layer using a sterile knife.
The experimental oil was Venezuelan extra-heavy crude oil with the addition of diluents. According to the analysis method mentioned in Section 2.7, the oil contained 35.20% aliphatics, 35.18% aromatics, 19.50% asphaltenes and 10.12% resins.
2.2 Media
The mineral salt medium (MSM) was composed of (L−1): (NH4)2SO4 5 g, KH2PO4 3.0 g, Na2HPO4·2H2O 2 g, MgSO4·7H2O 0.7 g, 1 mL of trace element solution and the medium pH was adjusted to 7.5. Solid MSM plate was prepared by adding 2.0 g agar into 100 mL MSM.
The trace element solution was composed of (L−1): CoCl2·6H2O 0.1 g, MnO2·4H2O 0.425 g, ZnCl2 0.05 g, NiCl2·6H2O 0.01 g, CuSO4·5H2O 0.015 g, Na2MoO4·2H2O 0.01 g, Na2SeO4·2H2O 0.01 g, CaCl2 0.147 g.
R2A medium18 was composed of (L−1): peptone 0.5 g, yeast extract 0.5 g, casamino acid 0.5 g, glucose 0.5 g, soluble starch 0.5 g, K2HPO4 0.3 g, MgSO4·7H2O 0.05 g, sodium pyruvate 0.3 g and the medium was adjusted to 7.5. Solid R2A medium was prepared by adding 2.0 g of agar into 100 mL R2A.
2.3 Isolation and screening of extra-heavy oil degrading bacteria
Bacteria strains were isolated from the contaminated soil by cultivation in R2A medium at 30 °C for 7 days. Selection of strains able to degrade hydrocarbons was conducted by cultivating 5 mL cultured aliquots on MSM ager plates with 0.1% (w/v) of extra-heavy oil as the sole carbon source and energy at 30 °C for 7 days. One strain, designated strain C-1, was selected due to its good ability in the process of extra-heavy oil degradation.
2.4 Morphological and biochemical characteristics
The morphological properties of strain C-1 were examined by optical microscopy. The typical biochemical and physiological characteristics of strain C-1, including Gram staining, catalase activity, oxidase activity, Voges–Proskauer reactions, methyl red, nitrate reduction, acid from glucose, indole production, nitrate reduction and hydrogen sulfide production, were systematically analyzed according to Bergey's Manual of Determinative for Bacteriology.19
2.5 Analysis of 16S rDNA sequence and phylogenetic analysis
Genomic DNA was extracted with D3350-01 genome DNA isolation kit (Sangon Ltd., Shanghai, China), and the extracted DNA was amplified by PCR in a peqSTAR 96 universal thermocycler (PEQLAB Biotechnology) using the genomic DNA as template and the bacterial universal primers were as follows: F27(5′-CAGCGGTACCAGAGTTTGATCCTGGCTCAG-3′); R1492(5′-CTCTCTGCAGTACGGCTACCTTGTTACGACTT-3′). The PCR amplification was performed as follows: 94 °C for 45 s, followed by 30 cycles of 94 °C for 45 s, 55 °C for 30 s and 72 °C for 1.5 min and a final extension at 72 °C for 10 min. The PCR product was sequenced by a commercial service (BGI, China). The sequences were aligned with the 16S rDNA sequence of each strain by Clustal X software.
2.6 Carbon source utilization
Based on the result of the growth curve (Fig. S1†), the cultivation time of strain C-1 was chosen for 3 days (the logarithmic growth phase). To test the ability of strain C-1 to grow on different hydrocarbons as sole carbon sources, cells from 5 mL 3 day-old cultured (OD600 value of 0.9) in R2A medium were harvested by centrifugation at 5000 rpm for 10 min and then washed three times with sterile saline solution to remove the residual organic compounds from the cultured solution, after which they were inoculated into 100 mL of MSM medium supplemented with liquid or solid hydrocarbons in 250 mL Erlenmeyer flasks. The final concentrations of n-alkanes (C6, C16, C24, C40), cyclohexane (C6) and aromatic naphthalin, phenanthrene, fluoranthene, anthracene, pyrene, benzene and ethanol in the flasks were 0.3% (v/v) for liquid alkanes, 0.05% (w/v) for solid alkanes and aromatic hydrocarbons. Two controls, one containing cells and no hydrocarbons and another containing hydrocarbons and no cells, were used. All cultures were incubated in the dark at 30 °C for 7 days while shaking at 180 rpm, then cell growth was recorded by measuring OD600 in the medium.
2.7 Degradation of extra-heavy oil by strain C-1
After growth in R2A liquid medium at 30 °C for 3 days, cells in 5 mL broth (OD600 value of 0.9) were harvested by centrifugation at 5000 rpm for 10 min, washed three times with distilled saline solution and inoculated into 100 mL MSM medium in 250 mL Erlenmeyer flasks amended with 0.1% (w/v) heavy crude oil. The culture conditions were kept at 30 °C with shaking at 180 rpm for 14 days. Meanwhile, a control group was established without adding strain C-1. All experiments were performed in triplicate.
The residual extra-heavy oil in each Erlenmeyer flask were extracted with 40 mL CCl4 at time intervals of 1, 2, 4, 6, 8, 10, 12 and 14 days, and the extracted extra-heavy oil concentration was measured by an infrared (IR) spectrophotometer (OIL-460, China Invent Instrument Tech. Ltd., China) according to the national standard method in China.20 Meanwhile, the value of OD600 was measured for evaluating the influence of extra-heavy oil on the growth of strain C-1.
2.8 Analysis of saturates, aromatics, resins, and asphaltenics in extra-heavy oil
The relative content of saturates, aromatics, resins, and asphaltenics in extra-heavy oil were analyzed using the methods described.21 The oil was dissolved in n-heptane to separate insoluble asphaltenes, and further fractionated using activated alumina column chromatography. The aliphatic fractions were eluted with petroleum ether, the aromatic fractions were eluted with benzene, and after that, resin fractions were eluted with the mixture of dichloromethane and ethanol (1
:
1 volume ratio), dichloromethane and ethanol. The content of each fraction is obtained by weighing.
2.9 GC-MS analysis of hydrocarbon degradation in extra-heavy oil
The aliphatic and aromatic eluent were concentrated to a volume of 1.0 mL, and then analyzed by GC-MS (Agilent 7890A/5975C, Agilent Technologies Inc., Santa Clara, CA, USA) equipped with an HP-5MS fused-silica capillary column.22 Peak areas of each compound were normalized with respect to the internal standard (17(α),28(β)-hopane) for n-alkanes and reported in this paper as relative abundance, using the methods described.23,24
3. Results and discussion
3.1 Isolation and identification of strain C-1
Nine extra-heavy oil bacterial strains were isolated from long-term Venezuelan extra-heavy oil contaminated soil collected from the Liaohe oilfield. The strain that showed the highest degrading ability and grew best in extra-heavy oil, named C-1, was selected for further study. Strain C-1 is Gram-positive (Fig. 1b), rod-like and almost 1.29 μm long (Fig. 1c). Colonies grown on R2A agar for 3 days at 30 °C were smooth, circular convex, wet and orange in color (Fig. 1a).
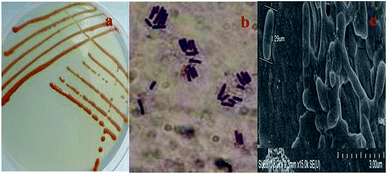 |
| Fig. 1 Photographs of colonies and cells of strain C-1: (a) photograph of C-1 colonies on R2A solid media plate; (b) Gram stain photograph of C-1; (c) SEM photograph of C-1 (15.0 × k). | |
The strain was first identified by classical biochemical tests, and then molecular identification was performed for strain C-1 by amplification and sequencing the 16S rDNA sequencing and comparing them to the database of known 16S rDNA sequences. The results of biochemical identification were shown in Table 1. Strain C-1 was positive for catalase and negative for acid from glucose, oxidase, methyl red, nitrate reduction, indole production, citrate utilization, Voges–Proskauer reaction, hydrogen sulfide. The molecular identification of strain C-1 show that this strain belongs to Dietzia cercidiphylli (99%). The phylogeny tree of this bacterium is shown in Fig. 2.
Table 1 Typical biochemical characteristics of strain C-1
Characteristic |
Strain C-1 |
Acid from glucose |
− |
Oxidase test |
− |
Catalase activity |
+ |
Methyl red |
− |
Nitrate reduction |
− |
Indole production |
− |
Citrate utilization test |
− |
Voges–Proskauer reaction |
− |
Hydrogen sulfide test |
− |
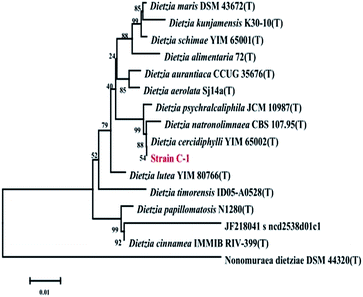 |
| Fig. 2 Phylogenetic analysis of strain C-1 and related species by the Neighbor-Joining method based on 16S rDNA sequences. Bootstrap values (%) are indicated at the nodes, the scale bars represent 0.01 substitutions per site. | |
3.2 Carbon source utilization of strain C-1
Twelve kinds of carbon source were tested as sole carbon substrates of C-1, including various low and high molecular weights of PAHs and some kinds of n-alkanes. All of them exist ubiquitously in the oil contaminated soil. Strain C-1 was found to grow obviously with C16 n-alkanes and ethanol, while it grew a bit slower with C40 n-alkanes, but could not use cyclo-hexane (C6), C24 n-alkanes, naphthalene, phenanthrene, fluoranthene, anthracene, pyrene, benzene for growth (Table 2). It could be inferred that strain C-1 could degrade length and longer length chain n-alkanes of extra-heavy oil.
Table 2 Utilization of carbon substrates by strain C-1a
Carbon source |
Strain C-1 |
Growth was followed by measuring the increase of OD600nm for 7 days. (++) Good growth: OD600nm > 0.2; (+) growth: OD600nm > 0.1; (−) growth: OD600nm < 0.05. |
n-C6 |
− |
Cyclohexane |
− |
n-C16 |
++ |
n-C24 |
− |
n-C40 |
+ |
Naphthalene |
− |
Phenanthrene |
− |
Fluoranthene |
− |
Anthracene |
− |
Pyrene |
− |
Benzene |
− |
Ethanol |
++ |
3.3 Degradation kinetics of extra-heavy oil
Remediation time can be roughly determined from the degradation step of hydrocarbons in the contaminated soil samples. A number of experimental studies have shown that biodegradation kinetics can be approximated with first order kinetics:25–28
where C is the residual oil content in the media at time t (mg L−1), C0 is the initial oil content in the media (mg L−1), k is the biodegradation rate constant (per day) and t is the time (day).
Change in the concentration of extra-heavy oil was shown in Fig. 3. During the initial 10 days, a decrease of concentration was observed, however, there was a gradual plateau from 10 to 14 days. Extra-heavy oil degradation kinetics was estimated in a first-order degradation rate model (Fig. 3), which gave good description of extra-heavy oil concentration changes. The first-order rate coefficient k (per day) was calculated by applying non-linear regression to the experimental data using SPSS. The k value for extra-heavy oil degradation by strain C-1 was equal to 0.021 per day. Compared with other degradation rate coefficient,29,30 the k value was higher. It indicated that strain C-1 had a good activity for degrading extra-heavy oil.
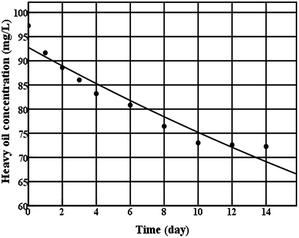 |
| Fig. 3 Extra-heavy oil degradation kinetics curve of strain C-1 (● experimental data; — Monod model kinetic curve). | |
3.4 Biodegradation of extra-heavy oil
Cell growth and extra-heavy oil degradation efficiency of strain C-1 was presented in Fig. 4a. During the initial 10 days, rapid cell growth and sharp increase of extra-heavy oil degradation efficiency were monitored, after that, with the decrease of cell number, extra-heavy oil degradation efficiency remained the 25.58 ± 0.48% (the largest degradation efficiency) until the end of the experiment (14 days). It showed that strain C-1 completely its degradation process of extra-heavy oil within 10 days. Comparisons with other heavy oil-degrading strains suggested that strain C-1 showed high activity in extra-heavy oil degradation.17,31 The result was consistent with the degradation kinetics of strain C-1.
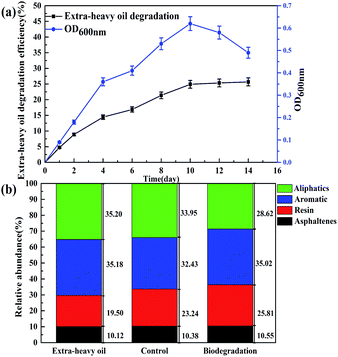 |
| Fig. 4 Changes in degradation efficiency, microbial cell number (a) and four fractions (b). | |
Fig. 4b showed the change of saturates, aromatics, resins, and asphaltenics in extra-heavy oil. After 14 days of incubation (on day 14), the relative content of aliphatics was decreased to 28.62% from 33.95%, causing relative enrichment in aromatics, resins and asphaltenes and redistribution of relative content. It could be inferred that strain C-1 could only degrade aliphatics, but not aromatics, resins and asphaltenes in extra-heavy oil. To further verify the results, the variation of aliphatics and aromatics was analyzed by GC-MS. GC-MS analysis demonstrated that strain C-1 could reduced aliphatics when compared to the control whereas no change of aromatics was monitored for strain C-1 (Fig. S2†).
The above results about the degradation characteristics of strain C-1 were consistent with previous studies. Although some Dietzia strains have been reported to utilize pure polycyclic aromatic groups for growth,17,31,32 no obvious degradation of polycyclic aromatic groups of crude oil had been reported for these strains. In addition, only the gene encoding alkanes terminal monooxygenases33 were amplified from Dietzia sp.;16,17 the gene encoding polycyclic aromatic groups dioxygenases34 has not yet been reported from Dietzia sp. More research is needed to answer this question (Fig. 5).
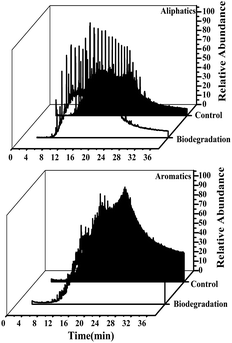 |
| Fig. 5 GC-MS spectrum of aliphatics and aromatics of extra-heavy oil. | |
3.5 Biodegradation of n-alkanes of extra-heavy oil
Extra-heavy oil is a complex mixture primarily composed of insoluble compounds, including different chain-length n-alkanes, which are not easily dispersed in water. GC-MS analysis detected someindividual n-alkanes, which included 37.59% of n-C44; 24.36% of n-C20, n-C21, and n-C24; and 38.06% of n-C13–C17 (Fig. 7). For strain C-1, n-C13–C17 had higher removal efficiencies, followed by n-C44, n-C20, n-C21, and n-C24 (Fig. 7a). Fig. 7b shows that the 14 day removal efficiencies of n-C13, n-C14, n-C15, n-C16, n-C17, and n-C44 were 43.11 ± 0.7%, 69.43 ± 1.3%, 55.69 ± 0.8%, 51.49 ± 0.4, 15.56 ± 0.3 and 32.4 ± 3.0%, respectively; however, n-C20, n-C21, and n-C24 had almost no degradation. Variation in removal efficiencies led to changes in the relative abundance of n-C44, n-C20, n-C21, n-C24 and n-C1–C17 groups after 14 days of incubation (Fig. 6).
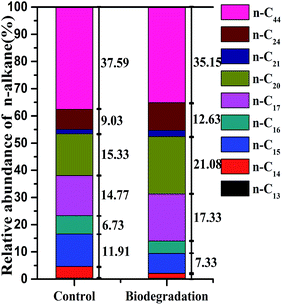 |
| Fig. 6 Relative abundance (%) of n-alkanes after 14 days of strain C-1 biodegradation. | |
The above results showed that strain C-1 had better degradation for n-alkanes with medium length (C13–C17) chain and longer length (C44) chain, the n-alkanes with long length (including C20, C21 and C24) chain had no degradation. These findings are consistent with previous research. The widely accepted n-alkanes sequential degradation theory suggests that C12–C15 are preferentially degraded during the early stages of crude oil biodegradation,15,17 which could be one of the reasons why strain C-1 had superiority in biodegradation C13–C17 hydrocarbons during the 14 days cultivation (Fig. 7). In fact, a novel AlkB rubredoxin fused alkane hydroxylase encoding gene was cloned in Dietzia strain DQ12-45-1b (strain C-1 belonging to Dietzia sp.) in an accompanying study with the GenBank Accession Number of HQ850582, which was proved to be responsible for the degradation of longer hydrocarbons. The CYP153 gene was cloned and sequenced in another accompanying study with the ability to degrade n-alkanes with the chain-length of C14 and C16.17 Overall, the chemical composition data (measured by GC-MS) corroborated the extra-heavy oil degradation data and carbon source utilization result, showing that strain C-1 could use length (C13–C17) and longer length chain (C44) n-alkanes as the sole carbon sources.
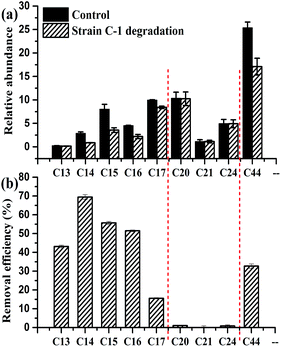 |
| Fig. 7 Relative abundance (a) corresponding removal efficiencies (b) of individual n-alkanes compounds after 14 days of strain C-1 degradation. | |
4. Conclusions
Strain C-1 belonging to the genus of Dietzia was isolated and characterized according to its biochemical characteristics and 16S rDNA sequences. It was Gram-positive, rod-like and almost 1.29 μm long. Colonies were smooth, circular convex, wet and orange in color. It was positive for catalase and negative for acid from glucose, oxidase, methyl red, nitrate reduction, indole production, citrate utilization, Voges–Proskauer reaction, and hydrogen sulfide. Strain C-1 can utilize C16 n-alkanes, C40 n-alkanes and ethanol as sole carbon source. Strain C-1 had a high extra-heavy oil degradation activity, and the reaction rate constant k was 0.021 per day. In 14 days of degradation, the strain could degrade nearly 25.58 ± 0.48% of extra-heavy oil. Four fractions and GC-MS analysis showed that strain C-1 could degrade aliphatics. GC-MS analysis also showed that strain C-1 could effectively degrade medium length (C13–C17) chain and longer length (C44) chain n-alkanes, but did not show obvious degradation for the long length (including C20, C21 and C24) chain n-alkanes. To date, it is first report that Dietzia strain is able to degrade extra-heavy oil, especially to C44 n-alkanes.
Acknowledgements
This work was funded by the National Natural Science Foundation of China (U1462201), and China National Petroleum Corporation projects (2014D-4607). We thank the Liaohe Petrochemical Company (Liaoning, China) for providing heavy oil samples.
References
- R. Meyer and E. Attanasi, Geological Survey Fact Sheet 70-03, 2003, http://pubs.usgs.gov/fs/fs070-03/fs070-03.html.
- G. D. Ji, Y. S. Yang, Q. Zhou, T. Sunc and J. R. Ni, Environ. Int., 2004, 30, 509–517 CrossRef CAS PubMed.
- R. M. Palou, M. Lourdes Mosqueira, B. Z. Rendónb, E. M. Juárezc, C. B. Huicochead, J. C. C. Lópeze and J. Aburto, J. Pet. Sci. Eng., 2011, 75, 274–282 CrossRef.
- H. J. Kang, S. Y. Lee, J. Y. Roh, U. H. Yim, W. J. Shim and J. H. Kwon, Environ. Sci. Technol., 2014, 48, 2962–2970 CrossRef CAS PubMed.
- M. Chen, P. Xu, G. M. Zeng, C. P. Yang, D. L. Huang and J. C. Zhang, Biotechnol. Adv., 2015, 33, 745–755 CrossRef CAS PubMed.
- A. Mrozik and Z. Piotrowska-Seget, Microbiol. Res., 2010, 165, 363–375 CrossRef CAS PubMed.
- H. Zhong, Y. Liu, Z. F. Liu, Y. B. Jiang, F. Tan, G. M. Zeng, X. Z. Yuan, M. Yan, Q. Y. Niu and Y. S. Liang, Int. Biodeterior. Biodegrad., 2014, 94, 152–159 CrossRef CAS.
- Y. Cheng, H. J. He, C. P. Yang, G. M. Zeng, X. Li, H. Chen and G. L. Yu, Biotechnol. Adv., 2016, 34, 1091–1102 CrossRef CAS PubMed.
- G. H. Lan, Q. Fan, Y. Q. Liu, Y. Liu, Y. C. Liu, X. B. Yin and M. N. Luo, Biochem. Eng. J., 2015, 103, 219–226 CrossRef CAS.
- L. Huang, T. Ma, D. Li, F. L. Liang, R. L. Liu and G. Q. Li, Mar. Pollut. Bull., 2008, 56, 1714–1718 CrossRef CAS PubMed.
- J. Vila and M. Grifoll, Appl. Environ. Microbiol., 2016, 82, 6232–6239 Search PubMed.
- Y. C. Liu, L. Z. Li, Y. Wu, W. Tian, L. P. Zhang, L. Xu, Q. R. Shen and B. Shen, Bioresour. Technol., 2010, 101, 310–316 CrossRef CAS PubMed.
- Z. Z. Zhang, L. X. Gai, Z. W. Hou, C. Y. Yang, C. Q. Ma, Z. G. Wang, B. P. Sun, X. F. He, H. Z. Tang and P. Xu, Bioresour. Technol., 2010, 101, 8452–8456 CrossRef CAS PubMed.
- H. J. Liu, J. Yao, Z. M. Yuan, Y. F. Shang, H. L. Chen, F. Wang, K. Masakorala, C. Yu, M. M. Cai, R. E. Blake and M. M. Choi, Int. Biodeterior. Biodegrad., 2014, 87, 52–59 CrossRef CAS.
- G. Bødtker, I. V. Hvidsten, T. Barth and T. Torsvik, Antonie van Leeuwenhoek, 2009, 96, 459–469 CrossRef PubMed.
- Z. Bihari, Z. Szabó, A. Szvetnik, M. Balázs, P. Bartos, P. Tolmacsov, Z. Zombori and I. Kiss, Z. Naturforsch., C: J. Biosci., 2010, 65, 693–700 CAS.
- X. B. Wang, C. Q. Chi, Y. Nie, Y. Q. Tang, Y. Tan, G. Wu and X. L. Wu, Bioresour. Technol., 2011, 102, 7755–7761 CrossRef CAS PubMed.
- D. J. Reasoner and E. E. Geldreih, Appl. Environ. Microbiol., 1985, 49, 1–7 CAS.
- S. G. Holt, N. R. Kriey, P. H. A. Sneath, J. T. Staley and S. T. Williams, Bergy's manual of determinative for bacteriology, Williams and Wilkins, New York, 1998 Search PubMed.
- MEPChina, Chinese Ministry of Environmental Protection, 2012 Search PubMed.
- N. X. Huang, H. R. Ma, X. R. Wang, J. F. Feng and P. Ding, Journal of shanxi university of science & technology (Shanxi Keji Daxue), 2003, 21, 25–29 Search PubMed.
- B. Cai, J. Ma, G. X. Yan, X. L. Dai, M. Li and S. H. Guo, Biochem. Eng. J., 2016, 112, 170–177 CrossRef CAS.
- R. C. Prlnce, D. L. Elmendorf, J. R. Lute, C. S. Hsu, C. E. Haith, J. D. Senius, G. J. Dechert, G. S. Douglas and E. L. Butler, Environ. Sci. Technol., 1994, 28, 142–145 CrossRef PubMed.
- I. Tolosa, S. D. Mora, M. R. Sheikholeslami, J. P. Villeneuve, J. Bartocci and C. Cattini, Mar. Pollut. Bull., 2004, 48, 44–60 CrossRef CAS PubMed.
- P. Hohener, C. Duwig, G. Pasteris, K. Kaufmann, N. Dakhel and H. Harms, J. Contam. Hydrol., 2003, 66, 93–115 CrossRef CAS PubMed.
- E. Collina, G. Bestetti, P. Di Gennaro, A. Franzetti, F. Franzetti, M. Lasagni and D. Pitea, Environ. Int., 2005, 167–171 CrossRef CAS PubMed.
- J. L. Chen, M. H. Wong, Y. S. Wong and N. F. Y. Tam, Mar. Pollut. Bull., 2008, 57, 695–702 CrossRef CAS PubMed.
- S. Maletić, B. Dalmacija, S. Rončević, J. Agbaba and O. Petrović, Water, Air, Soil Pollut., 2009, 202, 149–159 CrossRef.
- E. Y. Hwang, W. Namkoong and J. S. Park, Compost Sci. Util., 2001, 9, 143–149 CrossRef.
- B. E. Abbassi and W. D. Shquirat, Water, Air, Soil Pollut., 2008, 192, 221–226 CrossRef CAS.
- I. V. D. Weid, J. M. Marques, C. D. Cunha, R. K. Lippi, S. C. C. D. Santos, A. S. Rosado, U. Lins and L. Seldin, Syst. Appl. Microbiol., 2007, 30, 331–339 CrossRef PubMed.
- H. A. Awadhi, R. H. D. Sulaiman, H. M. Mahmoud and S. S. Radwan, Appl. Microbiol. Biotechnol., 2007, 77, 183–186 CrossRef PubMed.
- L. Li, X. Liu, W. Yang, F. Xu, W. Wang, L. Feng, M. Bartiam, L. Wang and Z. H. Rao, J. Mol. Biol., 2008, 376, 453–465 CrossRef CAS PubMed.
- J. S. Seo, Y. S. Keum and Q. X. Li, Int. J. Environ. Res. Public Health, 2009, 6, 278–309 CrossRef CAS PubMed.
Footnote |
† Electronic supplementary information (ESI) available. See DOI: 10.1039/c7ra00786h |
|
This journal is © The Royal Society of Chemistry 2017 |