DOI:
10.1039/C7RA00743D
(Paper)
RSC Adv., 2017,
7, 34473-34481
Protective effect and mechanistic evaluation of linalool against acute myocardial ischemia and reperfusion injury in rats
Received
17th January 2017
, Accepted 13th June 2017
First published on 10th July 2017
Abstract
The present study describes the protective effect of linalool against the isoproterenol (ISO)-induced myocardial infarction in adult male Sprague Dawley rats. The results of the study showed that linalool causes considerable improvement of the ischemic heart via number of mechanisms. It has been found that it causes significant reduction in the level of serum LDH and CK-MB levels together with improvement in LVSP, +dp/dtmax and −dp/dtmax 24 h after ischemia/reperfusion (I/R). Use of linalool also showed reduction in the levels of Tn-T (cardiac biomarker) and cytokines, such as TNF-α and IL-6. Furthermore, significant inhibition of MDA and MPO activities was also reported in the linalool receiving group together with restoration of the decreased SOD and GPx activities. The western blot analysis of linalool was also carried out to determine its effect on the expression of NF-κB. The effect of linalool was found in the results of the histopathology analysis, which confirmed that the linalool treated group showed restoration of microstructural changes in cardiac tissues as compared to the control. Linalool showed significant cell death and apoptosis of H9c2 cells during I/R. It has been found that linalool showed up-regulation of VEGF-B mRNA and protein levels, which promoted the activation of Akt and the inhibition of GSK3β as a possible additional mechanism to exert a cardioprotective effect.
Introduction
Myocardial diseases greatly contribute to the major cause of morbidity and mortality in both the developed and the developing countries.1–3 As a vital organ of the body, the improper functioning of the heart has severe adverse effects on the other organs. Among the factors responsible for the deterioration of the heart, insufficient oxygen (O2) supply to the myocardial muscles as a result of poor blood flow leads to the propagation of myocardial infarction (MI).4,5 It is a condition where myocardial muscles are damaged which often results in necrosis. The best possible therapeutic measure to overcome this phenomenon is the re-establishment of the blood flow. However, during this reestablishment, the heart undergoes a certain cascade of reactions, which result in the generation of reactive oxygen species (ROS) and apoptosis of myocardial tissues which ultimately leads to the reperfusion injury.6–9 Thus, recent studies have suggested that, with the timely intervention of appropriate therapeutics, it should be possible to manage the condition and provide a beneficial effect.
In this regard, natural products, because of their very high antioxidant capacity, have been found to be very useful in treating reperfusion injury and to give symptomatic relief by inhibition of the reactive mediators.10–12 They are also able to block the apoptotic process and this causes reduction in cardiac injury induced by ischemic/reperfusion (I/R) injury. Among the diverse array of the natural compounds, linalool, a major component of the essential oil identified as a volatile component from many aromatic plant species, has been reported to exhibit numerous pharmacological activities, such as anti-oxidant,13 anti-inflammatory,14,15 hepatoprotective and lipopolysaccharide (LPS) induced acute lung injury in mice.16 Concerning its tremendous antioxidant potential, no exhaustive report has discussed its effect against the I/R injury. Thus in the present study, it was intended that the protective effect of linalool against the I/R injury in rats together with the probable mechanism of action would be elucidated.
Result
Effect of linalool on the ISO-induced myocardial necrosis in rats
As shown in Fig. 1, the outcome of linalool on the serum level of the lactate dehydrogenase (LDH) and creatine kinase-MB (CK-MB) in the myocardial injury induced by isoproterenol (ISO) was determined. The study showed that the serum levels of both CK-MB and LDH were found to be significantly elevated in the ISO-treated rats, whereas in the group treated with linalool there was a noteworthy decline in serum LDH and CK-MB levels as compared with the results for the ISO treated group.
 |
| Fig. 1 Effect of linalool on the serum (A) LDH and (B) CK-MB levels in ISO-induced cardiac injury. *P < 0.05 and **P < 0.01 versus the vehicle treated group. | |
Effect of linalool on myocardial ability
In this study, the effect of linalool on left ventricular systolic pressure (LVSP) and ±dp/dtmax of the left ventricle (LV) during myocardial I/R were determined. As shown in Fig. 2A–C, in comparison with the ISO treated animals, the linalool treated animals showed considerable improvement in LVSP, +dp/dtmax and −dp/dtmax 24 h after I/R.
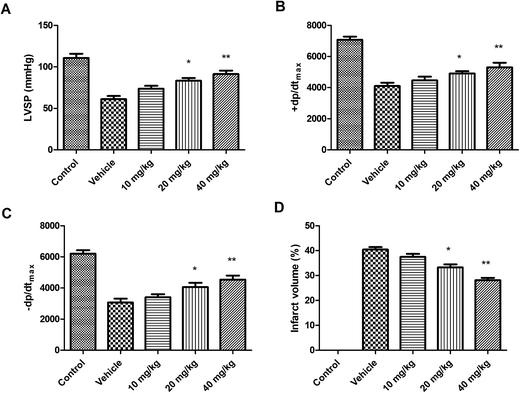 |
| Fig. 2 Effect of linalool on the (A) LVSP, (B) ±dp/dtmax, (C) −dp/dtmax and (D) infarct volume after I/R in experimental animals. *P < 0.05 and **P < 0.01 versus vehicle treated group. | |
Effect of linalool on myocardial infarct volume
As shown in Fig. 2D, it has been found that in linalool treated animals, the infarct size has been significantly reduced when compared with the ISO treated group. Thus, it has been shown that linalool is effective in modulating the myocardial I/R injury.
Effect of linalool on serum levels of Tn-T, TNF-α and IL-6
As shown in Fig. 3, it was found that the levels of cardiac specific troponin (Tn-T), tumour necrosis factor-α (TNF-α) and interleukin-6 (IL-6) were found to be considerably enhanced in rats treated with ISO. Whereas, in linalool treated rats, the levels of these markers were found to be considerably decreased.
 |
| Fig. 3 Effect of linalool on the myocardial function and infarct volume. Where, (A) Tn-T, (B) TNF-α and (C) IL-6 levels. *P < 0.05 versus vehicle-treated group. **P < 0.01 versus vehicle-treated group. | |
Effect of linalool on production of MDA and on MPO, SOD and GPx levels
As shown in Fig. 4, the generation of malondialdehyde (MDA) and myeloperoxidase (MPO) were significantly elevated. The activities of glutathione peroxidase-1 (GPx) and superoxide dismutase (SOD) were found to be considerably decreased in the ISO treated animals. Furthermore, as the treatment with linalool started, it significantly inhibited the MDA and MPO activities as well as restoring the decreased SOD and GPx activities.
 |
| Fig. 4 Effect of linalool on production of (A) MDA and on (B) MPO, (C) SOD and (D) GPx levels. *P < 0.05 versus vehicle treated group; **P < 0.01 versus vehicle treated group. | |
Effect of linalool on pathological changes
As shown in Fig. 5, after the first 24 h, the infarct area was prominent with widespread tissue necrosis, together with an increase in infiltration, and signs of haemorrhage. Whereas, in rats treated with linalool, there were signs of significant restoration of the normal microstructural changes in a dose-dependent manner.
 |
| Fig. 5 Effect of linalool on the histopathology of cardiac tissues, where, (A): vehicle, (B): linalool (10 mg kg−1), (C): linalool (20 mg kg−1), (D): linalool (40 mg kg−1). (Magnification: 100×). | |
Western blotting analysis of the effect of linalool on IκB-α and NF-κB phosphorylation
As shown in Fig. 6, it was found that linalool causes significant inhibition of phosphorylated IκB-α and NF-κB expression when compared to the myocardial tissues after I/R.
 |
| Fig. 6 Effect of linalool on IκB-α and NF-κB phosphorylation. | |
Effect of linalool on the I/R induced cell death and apoptosis of cardiomyocytes
The next part of study was aimed at elucidating the effect of linalool on the cell death occurring during the I/R. For this experiment, calcein-AM/propidium iodide (PI) double staining was carried out. It has been found that a large quantity of H9c2 cells (fetal rat cardiomyocyte derived cell line) were reported to be dead after the I/R, whereas the use of linalool inhibited the cell death when compared to the results obtained with the I/R group as shown in Fig. 7A and B. The effect of linalool on the apoptosis was also quantified with using western blot analysis and quantification of the expression of BCL2-associated X protein (Bax), an apoptotic protein in heart tissue. Results showed that the level of Bax was found to be elevated more in the I/R group than in the control group. However, the group receiving linalool showed significant down-regulation of the expression of the Bax protein level (Fig. 7C). As shown in Fig. 7D, it has been found that the rate of apoptosis was significantly attenuated under influence of linalool.
 |
| Fig. 7 Linalool (LN) causes attenuation of I/R induced cell death and apoptosis either in vitro or in vivo. (A) Evaluation of cell death in H9c2 cells using calcein-AM/PI double staining; (B) the cells survival rate; (C) the protein level of Bax in heart tissues was determined using a western blot technique; (D) apoptotic cells were detected using flow cytometry. #P < 0.05 versus control group, *P < 0.05 versus I/R group. | |
Effect of linalool on the expression of VEGF-B and regulated Akt/GSK3β signaling pathway
As shown in Fig. 8A and B, the administration of linalool (LN receiving group) causes significant up-regulation of messenger ribonucleic acid (mRNA) and protein levels of vascular endothelial growth factor B (VEGF-B) when compared with the results for the I/R group. Furthermore, the level of phosphorylated protein kinase B (Akt) (Ser473) and glycogen synthase kinase 3 beta (GSK3β) (Ser 9) were also found to be up-regulated in the linalool treated group (Fig. 8C and D).
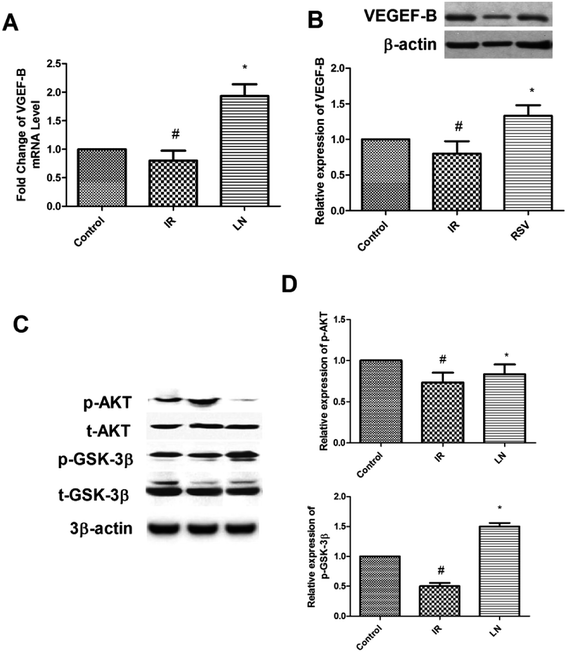 |
| Fig. 8 Linalool regulated the VEGF-B and Akt/GSK3β signaling pathways. (A) The linalool up-regulated the mRNA level of VEGF-B in heart tissues; (B) linalool up-regulated the protein level of VEGF-B in heart tissues; (C) linalool up-regulated phosphorylated Akt(Ser473) and GSK3β(Ser9) protein level in heart tissues; (D) quantification of phosphorylated Akt and GSK3β in heart tissues. #P < 0.05 versus control group, *P < 0.05 versus I/R group. | |
Effect of linalool on the echocardiographic indicators of cardiac morphometry and function
The results of results of echocardiographic analysis are shown Table 1. It has been found that linalool treatment produced considerable improvement in the heart function as shown by the improvement in the posterior wall thickness of the LV together with an increase in the mass of the LV. The end-systolic volume (ESV) was also found to be increased in rats treated with linalool. A significant reduction was observed in the ejection fraction with reduction in the clearance suggesting the protective role of linalool in myocardial I/R injury.
Table 1 The effect of linalool on cardiac function in rats (echo readings)a
Parameters |
Pre |
Post |
The values are mean ± SEM. AD: aortic diameter, CI: cardiac index, EDV: end-diastolic volume of the LV, EF: ejection fraction of the LV, ESV: end-systolic volume, LVM: left ventricular mass, PWT: left ventricular posterior wall thickness *P < 0.05 and **P < 0.01 versus vehicle treated group. |
PWT (mm) |
1.40 ± 0.02 |
1.53 ± 0.05* |
LVM (g) |
1.12 ± 0.02 |
1.31 ± 0.03 |
AD (mm) |
3.25 ± 0.22 |
3.58 ± 0.31 |
ESV (µl) |
145 ± 9 |
198 ± 11** |
EDV (µl) |
323 ± 14 |
378 ± 18** |
EF (%) |
55.1 ± 2.4 |
47.6 ± 2.6** |
CI (µl [min g]−1) |
185 ± 5 |
167 ± 8** |
Discussion
In the present investigation, it has been observed that the myocardial ability of the rats receiving the linalool showed significant improvement when compared to the I/R groups in the I/R challenge study. This was further supported by the reduction in the infarct size, which was subsequently found to agree with the results of the histopathological analysis. The linalool would be able to modulate the cardiac function and it was suggested that it would be able to inhibit the necrosis of the cardiac tissues.
As shown in recent studies, the Tn-T is well established as a significant marker of cardiac damage.17,18 Thus, in the present study, it was shown that linalool caused significant reduction in the serum level of Tn-T together with prominent protective action against myocardial degradation. It has also been well established that the generation of free radicals following myocardial injury plays a significant role in the progression of the damage. These radicals are believed to cause destruction of proteins, DNA, and lipids, together with severe membrane damage which ultimately induces the death of the tissue.19–21 In the present study, it was found that linalool causes a reduction in MDA production and restored the level of SOD and GPx in affected cardiac tissue to a major extent. This suggests that linalool could exert its action to protect the myocardial damage by possibly limiting the generation of free radicals which can prevent the necrosis of the tissue, and thus, tissue activity will be restored.
The myocardial damage may be the result of the infiltration of polymorphonuclear leukocytes (PMNs), and can cause damage of the cardiomyocytes via the generation of inflammatory mediators.22–25 Thus, limiting its infiltration may have some protective effect against the myocardial damage in an I/R injury. It mediates its action via generation of the ROS, and other hydrolytic enzymes which might lead to the progression of micro-structural injury. It has been found that, after the I/R insult, the activity of MPO was significantly elevated together with an increased leukocyte accumulation. Thus, it has been found that linalool reduces the membrane permeability towards the PMN and MPO activity in the ischemic myocardial tissues. Earlier studies have stated that the role of the activation of NF-κB which is connected with the phosphorylation of IκB-α and transport of p65 from the nucleus.26,27 In the present study, it was found that linalool was able to inhibit the phosphorylation of IκB-α together with reduction of NF-κB in myocardial tissues. Thus, it has been suggested that the protective effects of linalool could be attributed to the attenuation effect which might be because of the signals of the inflammation via inhibition of the NF-κB activation.
The role of VEGF-B in the cardiovascular system is still a matter of debate.28 Therefore, not much is known about the involvement of VEGF-B in the progression or generation of MI. Thus, in the present study, the role of VEGF-B in the cardio-protective effect of linalool as suggested by previous experiments was investigated. The results of the study showed that linalool causes significant up-regulation of VEGF-B expression in the Langendorff perfusion assay. In an extension of this study, an in vitro study was carried out to look at the role of VEGF-B in H9c2 cells because of its excellent function in the I/R injury model.29,30 As suggested previously, the ROS is the important predictor for the progression of an MI, thus to replicate this behaviour in an in vitro system, rotenone was used. The rotenone was believed to be the inhibitor of mitochondrial electron transport chain complex I, and promotes the production of ROS in numerous cells. Previous studies suggested that rotenone causes the induction of ROS-promoted cellular death in H9c2 cells and generates ischemia in renal cells.31,32 Thus, in accordance with the earlier experiments, the results showed that rotenone treatment can mimic I/R in rat cardiomyocytes (Fig. 7A and B). In the flow cytometric cell apoptosis assay, it was found that linalool causes significant inhibition of I/R induced apoptosis (Fig. 7D) together with modulation of the Bax protein expression (Fig. 7C) which might be suggested as a possible mechanism for the cardioprotective action of linalool. Recent studies concluded that activated Akt mediated inhibition of GSK3β could provide protection to the heart from myocardial I/R injury, suggesting the negative role of GSK3β in myocardial I/R.33,34 The cross-talk between Akt and GSK3β was also showed that Akt is upstream of GSK3β. Thus, in the present study, the effect of linalool on this complex was evaluated. As shown in Fig. 8, it has been found that linalool causes activation of Akt and inhibition of GSK3β after administration of linalool treatment (phosphorylation in Ser9 of GSK3β means its activity is inhibited).
The electrocardiogram (ECG) is an important tool for assessing the protective effect against the myocardial damage induced by ischemia. A study by Preda and Burlacu describes excellently the use of ECG in validating the myocardial I/R procedures in mice.35 Thus, in the present study, we investigated the protective effect of linalool in myocardial I/R injury via ECG. It has been found that linalool causes growth related increase in posterior ventricular wall thickness, end systolic and diastolic volumes, and reduces the ejection fraction.
Experimental
Chemicals
Linalool and the other chemicals used in the present study were obtained from Sigma-Aldrich (USA).
Animals
For the study, adult male Sprague Dawley (240–270 g) rats were obtained from the institutional animal house and were caged individually in polypropylene cages under controlled temperature and humidity with alternate, dark and light cycle and food and water ad libitum. The entire animal experiments were approved by the Institutional Animal Ethics Committee of Anyang District Hospital Henan Province, Henan, China (ref: 2016/ANDH/imed/A-205) and were performed in strict accordance with the US National Institutes of Health (NIH) guidelines for the care and use of laboratory animals.
Ethics statement
Published research must comply with the guidelines for animal welfare regulations of affiliated Anyang District Hospital Henan Province, Anyang, China. Authors should state in the manuscript text that animal experiments conform to institutional standards (ADHH/16/03/0245).
Induction of myocardial infarction using isoproterenol (ISO)
Briefly, a total of five sub-groups were formed, each containing 10 rats. The groups were as follows: group 1: control, group 2: ISO + saline, group 3: ISO rats + 10 mg kg−1 linalool, group 4: ISO rats + 20 mg kg−1 linalool, group 5: ISO rats + 40 mg kg−1 linalool. All the animals in the groups, except for the control group, received ISO (150 mg kg−1 subcutaneously, for two days). After three days of ISO administration, the blood was obtained from the retro-orbital veins. The serum obtained after centrifugation was used for the determination of the levels of LDH and CK-MB enzymes.
Initiation of the myocardial I/R injury
For induction of the anaesthesia, a barbiturate derivative was given by intra-peritoneal (ip) injection. Briefly, the coronary artery ligation was performed in accordance with earlier established procedures. A suture made of silk was passed underneath the left anterior descending coronary artery and tied. The considerable changes in the ECG were identified as the marker of a flourishing coronary occlusion. The rats were divided into two groups. The first group was further sub-divided into five groups with six rats in the each group: group 1: non-myocardial I/R rat, group 2: I/R + saline, group 3: ISO rats + 10 mg kg−1 linalool, group 4: ISO rats received + 20 mg kg−1 linalool, group 5: ISO rats + 40 mg kg−1 linalool. After 5 min of the reperfusion, all the experimental rats received treatment. For example, the first group of animals were used for the determination of various parameters, such as hemodynamic parameter, infarct size and serum levels of Tn-T, cytokines (e.g., TNF-α and IL-6). Whereas, the next group was used for the determination of the level of GPx, SOD, MDA and MPO activities as well as for the western blots analysis.
Langendorff perfusion test
The rats were anaesthetized with an ip injection of 10% chloral hydrate, supplemented with 0.1 ml heparin (5000 IU ml−1). The hearts were quickly excised from the heart cavity and arrested in ice-cold perfusion fluid, and the hearts were connected to the Langendorff apparatus by inserting a perfusion cannula into the aorta at a constant pressure (80 mm Hg). The hearts underwent retrograde perfusion with Krebs–Henseleit (K–H) solution 118 mM sodium chloride (NaCl), 4.7 mM potassium chloride (KCl), 1.2 mM magnesium sulfate (MgSO4), 1.2 mM (potassium dihydrogen phosphate (KH2PO4) 25 mM sodium bicarbonate (NaHCO3) 11 mM glucose, 2.5 mM calcium chloride (CaCl2) and 2 mM sodium pyruvate), equilibrated to pH 7.4 with a gas mixture comprising 95% O2 and 5% carbon dioxide (CO2). The perfusion solution was maintained at 37 ± 0.5 °C and the temperature was controlled using a water-jacketed organ chamber. A fluid filled balloon was inserted into the LV via the left auricle and connected to a pressure transducer to continuously monitor the changes of LV pressure. Isolated hearts were stabilized for 30 min with K–H solution, followed by 30 min of no-flow ischemia and 60 min of reperfusion to mimic I/R. Resveratrol (RSV; 10 µmol l−1) was added into K–H solution in the RSV group 15 min prior to the period of ischemia. No ischemia treatment was performed in control group. LVSP, maximal rate of increase of left ventricular pressure (+dp/dtmax), and maximal rate of decrease of left ventricular pressure (−dp/dtmax) were recorded using a computer-based data acquisition system (RM6240B, Chengdu, China).
Assessment of serum levels of Tn-T, TNF-α and IL-6
The blood samples were collected after 24 h of I/R and the serum levels of Tn-T, TNF-α and IL-6 were measured using enzyme-linked immunosorbent assay (ELISA) kits in accordance with the manufacturer's protocol.
Determination of the levels of MDA, SOD, GPx and MPO activities
The levels of MDA, SOD, GPx and MPO activities in myocardial tissues affected with ischemia were quantified using kits in accordance with the protocol supplied by the manufacturers.
Cell culture and in vitro I/R model
Fetal rat cardiomyocyte-derived H9c2 cells were purchased from the Type Culture Collection of the Chinese Academy of Sciences, Shanghai, China. Cells were cultured in Dulbecco's Modified Eagle's Medium (DMEM) supplemented with 10% fetal bovine serum (FBS), 100 U ml−1 of penicillin, and 100 µg ml−1 of streptomycin in 95% O2 and 5% CO2 at 37 °C. The culture medium was changed every two days, and cells were subcultured until they reached 60–70% confluence. The in vitro I/R model was established in H9c2 cells. H9c2 cells were treated with 5 µmol l−1 rotenone for 2 h, followed by rotenone free DMEM medium reperfusion for 1 h. Linalool (10 µmol l−1) was added into the culture medium 24 h prior to the rotenone treatment.
Calcein-AM/PI double staining
H9c2 cells were incubated in phosphate buffered saline (PBS) containing 2 µg ml−1 of calcein AM and 1 µg ml−1 of PI at 37 °C for 15 min. After washing with PBS, the cells were examined with fluorescence microscopy. Five random fields were observed, and the calcein AM positive area, and the global cells area were measured using ImageJ software (NIH, USA). The calcein AM positive area rate was defined as the percentage of the calcein AM positive area out of the whole cell area.
Cellular apoptosis
Apoptosis of H9c2 cells was observed using Hoechst 33342 staining (5 µg ml−1; Sigma, USA) and quantified using flow after cells were stained with annexin V and PI using a fluorescein isothiocyanate (FITC) annexin V apoptosis detection kit (BD Biosciences, USA).
Small interfering RNA (siRNA) transfection
VEGF-B siRNA was purchased from GenePharma (Shanghai, China). Briefly, H9c2 cells were cultured in DMEM medium (FBS free) for 2 h before siRNA transfection. VEGF-B siRNA (100 nM), or negative control (NC) siRNA was mixed with Lipofectamine 2000 (Invitrogen, USA). The cells were incubated with the transfection mixture for 6 h and then washed with DMEM medium containing 10% FBS. The cells were then incubated for an additional 24 h before harvest.
Western blot analysis
The heart samples after 24 of I/R were mixed in a buffer that consisted of 10 mM tris (pH 7.5), 1.5 mM magnesium chloride (MgCl2), 10 mM KCl, and 0.1% Triton X-100. The tissues were degraded using homogenization and the nuclei were isolated by centrifugation at 7500 rpm. The resulting supernatant was collected and stored at −80 °C before the analysis. The nuclear proteins were extracted at 4 °C by resuspending the nuclei pellet in a buffer consisting of 20 mM tris (pH 7.5), 20% glycerol, 1.5 mM MgCl2, 420 mM NaCl, 0.2 mM ethylenediaminetetraacetic acid (EDTA), and 0.1% Triton X-100, followed by 1 h incubation and after microcentrifugation for 15 min, the supernatant was collected. The concentration of protein was estimated using a bicinchoninic acid (BCA) assay kit (ThermoFisher, USA). Thereafter, the total protein extract (50 µg) was separated on 15% sodium dodecyl sulfate polyacrylamide (SDS-PAGE) gel and by probing with rabbit anti-phosphor-NF-κB and anti-phosphor-IκB-α using anti-β-actin antibody (control), VEGF-B, MnSOD (Abcam, USA), phosphorylated (Ser473) and total Akt, phosphorylated (Ser 9) and total GSK3β (Cell Signaling Technology, USA), Bax and β-actin (Santa Cruz Biotechnology, CA, USA).
Histopathological analysis
Briefly, the hearts of the experimental animals were sectioned and then fixed in 10% formalin and then the sections were embedded in paraffin and then cut in sections. The resulting sections were stained with hematoxylin and eosin (H&E) stain after fixation and evaluated under a microscope (100×).
Echocardiography
The echocardiography (echo) was performed on each of the rats on linalool at a single dose of 10 mg kg−1 to evaluate the effects on the cardiovascular morphology and function. Under anaesthesia with sodium pentobarbital (30 mg kg−1, ip), a 12 MHz transducer (HP SONOS 5500, Hewlett Packard, Inc., Andover, MA, USA) was used to obtain two-dimensional (2D) images of the LV at long and short axes. LV mass (LVM) and LV posterior wall thickness were measured from the M-mode LV tracings. LV ESV and LV EDV were calculated from the 2D images using a modified Simpson's rule. The LV ejection fraction (EF) was calculated from the EDV and ESV. The cardiac index (CI) was calculated as cardiac output adjusted for body weight.
Statistical analysis
The experimental data were expressed as mean ± SD, using the one-way analysis of ANOVA followed by the Tukey's test. The P value <0.05 was considered as statistically significant.
Conclusion
In conclusion, the theory that linalool protects the cardiac system of the rats in myocardial I/R injury and exerts its beneficial role via a number of mechanisms has been corroborated. However, its pharmacological significance in I/R warrants further investigation in terms of its exact mechanism of action. The present study may serve as a template for the further studies on linalool for I/R injury.
Conflict of interest
The authors declare no conflict of interest.
Notes and references
- V. T. Nkomo, J. M. Gardin, T. N. Skelton, J. S. Gottdiener, C. G. Scott and M. Enriquez-Sarano, Lancet, 2006, 368, 1005–1011 CrossRef.
- A. Boutayeb and S. Boutayeb, Int. J. Equity Health., 2005, 4, 2 CrossRef PubMed.
- A. E. Moran, K. Y. Tzong, M. H. Forouzanfar, G. A. Roth, G. A. Mensah, M. Ezzati, C. J. L. Murray and M. Naghavi, Glob. Heart., 2014, 9, 91–99 CrossRef PubMed.
- H. D. White and D. P. Chew, Lancet, 2008, 372, 570–584 CrossRef CAS.
- G. Ertl and S. Frantz, Cardiovasc. Res., 2005, 66, 22–32 CrossRef CAS PubMed.
- E. D. Grech, C. I. Jack, C. Bleasdale, M. J. Jackson, M. Baines, E. B. Faragher, C. R. Hind and R. A. Perry, Coron. Artery Dis., 1993, 4, 769–774 CrossRef CAS PubMed.
- D. Bell, M. Jackson, J. J. Nicoll, A. Millar, J. Dawes and A. L. Muir, Br. Heart J., 1990, 63, 82–87 CrossRef CAS.
- D. M. Ansley and B. Wang, J. Pathol., 2013, 229, 232–241 CrossRef CAS PubMed.
- N. G. Frangogiannis, C. W. Smith and M. L. Entman, Cardiovasc. Res., 2002, 53, 31–47 CrossRef CAS PubMed.
- H. Yin, L. Xu and N. A. Porter, Chem. Rev., 2011, 111, 5944–5972 CrossRef CAS PubMed.
- L. L. De Zwart, J. H. N. Meerman, J. N. M. Commandeur and N. P. E. Vermeulen, Free Radical Biol. Med., 1999, 26, 202–226 CrossRef CAS PubMed.
- T. P. A. Devasagayam, J. Tilak, K. K. Boloor, K. S. Sane, S. S. Ghaskadbi and R. D. Lele, J Assoc Physicians India, 2004, 52, 794–804 CAS.
- G.-H. Seol, P. Kang, H. S. Lee and G. H. Seol, BMC Neurol., 2016, 16, 4–9 CrossRef PubMed.
- M. Lavy, A. Zuker, E. Lewinsohn, O. Larkov, U. Ravid, A. Vainstein and D. Weiss, Mol. Breed., 2002, 9, 103–111 CrossRef CAS.
- E. Elisabetsky, J. Marschner and D. Onofre Souza, Neurochem. Res., 1995, 20, 461–465 CrossRef CAS PubMed.
- A. M. Kamatou and G. P. P. Viljoen, Nat. Prod. Commun., 2008, 3, 1183–1192 Search PubMed.
- K. M. Ver Elst, H. D. Spapen, D. N. Nguyen, C. Garbar, L. P. Huyghens and F. K. Gorus, Clin. Chem., 2000, 46, 650–657 CAS.
- N. Van Der Vekens, A. Decloedt, S. Ven, D. De Clercq and G. van Loon, J. Vet. Intern. Med., 2015, 29, 348–354 CrossRef CAS PubMed.
- M. Dizdaroglu, P. Jaruga, M. Birincioglu and H. Rodriguez, Free Radic. Biol. Med., 2002, 32, 1102–1115 CrossRef CAS PubMed.
- J. R. Speakman and C. Selman, BioEssays, 2011, 33, 255–259 CrossRef PubMed.
- L. L. de Zwart, J. H. Meerman, J. N. Commandeur and N. P. Vermeulen, Free Radic. Biol. Med., 1999, 26, 202–226 CrossRef CAS PubMed.
- P. Libby, Circulation, 2002, 105, 1135–1143 CrossRef CAS PubMed.
- Y. Feng and W. Chao, Int. J. Inflam., 2011, 2011, 170352 Search PubMed.
- D. J. Marchant, J. H. Boyd, D. C. Lin, D. J. Granville, F. S. Garmaroudi and B. M. McManus, Circ. Res., 2012, 110, 126–144 CrossRef CAS PubMed.
- K. H. Melamed and S. Z. Goldhaber, Circulation, 2014, 130, e334–e336 CrossRef PubMed.
- A. Wullaert, M. C. Bonnet and M. Pasparakis, Cell Res., 2011, 21, 146–158 CrossRef CAS PubMed.
- M. Sangeetha, M. S. Pillai, L. Philip, E. G. Lakatta and K. Shivakumar, Exp. Cell Res., 2011, 317, 899–909 CrossRef CAS PubMed.
- L. Zentilin, U. Puligadda, V. Lionetti, S. Zacchigna, C. Collesi, L. Pattarini, G. Ruozi, S. Camporesi, G. Sinagra, M. Pepe, F. A. Recchia and M. Giacca, FASEB J., 2010, 24, 1467–1478 CrossRef CAS PubMed.
- L. Sun, C. K. Isaak, Y. Zhou, J. C. Petkau, O. Karmin, Y. Liu and Y. L. Siow, Life Sci., 2012, 91, 151–158 CrossRef CAS PubMed.
- B. Wang, J. Shravah, H. Luo, K. Raedschelders, D. D. Y. Chen and D. M. Ansley, Biochem. Biophys. Res. Commun., 2009, 389, 105–111 CrossRef CAS PubMed.
- K. Radad, W. D. Rausch and G. Gille, Neurochem. Int., 2006, 49, 379–386 CrossRef CAS PubMed.
- N. Li, K. Ragheb, G. Lawler, J. Sturgis, B. Rajwa, J. A. Melendez and J. P. Robinson, J. Biol. Chem., 2003, 278, 8516–8525 CrossRef CAS PubMed.
- M. Le Grand, A. Rovini, V. Bourgarel-Rey, S. Honore, S. Bastonero, D. Braguer and M. Carre, Oncotarget, 2014, 5, 3408–3423 CrossRef PubMed.
- J. Ryder, Y. Su and B. Ni, Cell. Signalling, 2004, 16, 187–200 CrossRef CAS PubMed.
- M. B. Preda and A. Burlacu, Comp. Med., 2010, 60, 443–447 CAS.
|
This journal is © The Royal Society of Chemistry 2017 |
Click here to see how this site uses Cookies. View our privacy policy here.