DOI:
10.1039/C7RA00722A
(Paper)
RSC Adv., 2017,
7, 18946-18952
Stability of carbon and nitrogen isotopic compositions of the protein extracted from milk and their potential as “fingerprints” of geographical origin
Received
17th January 2017
, Accepted 16th March 2017
First published on 29th March 2017
Abstract
The present work aimed to determine whether isotopic compositions can be used as “fingerprints” in identifying the geographical origin of milk. For this purpose, δ13C and δ15N values of the proteins extracted from fifty-six Tetra Pak milk samples originating from four continents, including Australia (with New Zealand), Europe (Germany and France), North America (the United States) and Asia (China), were determined using elemental analyzer-isotope ratio mass spectrometry (EA-IRMS). The effects of storage time and temperature on the δ13C and δ15N values were also studied in order to evaluate the stability of the isotopic composition and hence the ability of the developed EA-IRMS method to identify the geographical origin of milk. The results indicated that there were no significant differences (P > 0.05) for δ13C and δ15N values for the samples stored under different conditions, demonstrating the stability of the isotopic compositions in the proteins extracted from these milk samples. The δ13C values of these extracted proteins ranged from −29.36‰ to −15.02‰. Their mean δ15N values were calculated to be between 4.55‰ and 5.80‰, with the highest, second-highest, third-highest, and lowest values for the samples from Australia (with New Zealand), Europe, America, and Asia, respectively. Significant differences (P < 0.05) were found in the δ13C and δ15N values between these four regions, verifying their potential as “fingerprints” of the geographical origin of milk. The results obtained confirm the suitability and potential of stable isotopic composition (δ13C and δ15N) analysis in determining the geographical origin of milk.
Introduction
Food adulteration and mislabeling have become two of the most serious problems in the food industry due to the low transport costs and increasing mobility of foodstuffs. Generally, food products that are of high quality or that originated from certain geographical regions can be sold at premium prices.1 However, some cheaper food products are always mislabeled as more valuable ones in order to yield greater profits, leading to an unfair and dishonest food market. This circumstance on the one hand threatens the livelihood of honest traders, and on the other hand infringes the rights of consumers.2 Fortunately, in recent decades, consumers have been paying increasing attention to the geographical origin of food products mainly because of its associations with food quality and food authenticity.3–5 Hence, it is of great importance to take measures to identify the geographical origin of food products.6
In 2001, Almeida & Vasconcelos (2001) adopted a relatively new technology, stable isotope ratio analysis, to identify the authenticity of wine provenance in the European wine industry.6,7 This technology has since been widely and successfully used to detect adulterations of food products such as honey,8–11 fruit juices,12,13 oils and cheese,14,15 and dairy products,6,16,17 as well as a few other food products.5,18,19
The isotope pairs most commonly measured when assessing the origin of a food product are the light element isotope pairs 2H/1H, 13C/12C, 15N/14N, 18O/16O and 34S/32S and the heavy element isotope pair 87Sr/86Sr.15 Physical, chemical, and biochemical processes could result in isotopic fractionation, leading to changes in the relative abundance of stable isotopes in different environmental conditions. A range of factors such as latitude, climate and agricultural practices could affect the degree of isotopic fractionation for the light elements.6 As for animal products, the stable isotopic composition of the tissues of the animals generally reflects the stable isotopic compositions of their ingested foods.15,20 Hence, the milk from cows with pastures as their main food source will directly reflect the stable isotope ratios in that particular region.21 Unfortunately, analysis of individual stable isotope ratios is insufficient to provide unequivocal and robust information about the origin of food products. However, analysis of multiple stable isotope ratios allows a stable isotopic “fingerprint” for the food product from the particular geographical region to be reliably developed.
Determination of the geographical origin of milk and milk products and the evaluation of their quality have recently become possible.6,16,20,22,23 It was reported that the geographical origin of milk samples from different regions of Europe could be identified by measuring the stable isotope ratios of oxygen (δ18O) in milk water which evaporated from milk sample and the nitrogen (δ15N) and carbon (δ13C) isotope contents of specific milk fractions in the samples.20 However, to the best of our knowledge, there has been no published report of the ability to identify the geographical origin of milk from more than one continent. In addition, the stability of using isotopic compositions to identify the geographical origin of food needs to be determined. Hence, the aim of the present study was to identify the detailed geographical origin of milk samples from countries of four continents (specifically Australia and New Zealand, Germany and France, the United States, and China) using the isotopic compositions of the protein extracted from the milk as the “fingerprints”. Moreover, the effects of storage conditions on the δ13C and δ15N values of the extracted milk protein were also investigated. The results obtained in this work confirmed the stability of these isotopic compositions in milk and the hence the potential of analyzing the isotopic composition in a milk sample for the purpose of determining its geographical origin.
Materials and methods
Materials, chemicals and samples
The casein having δ13C values of −26.98‰ ± 0.15‰ (n = 10) and δ15N values of 5.94‰ ± 0.20‰ (n = 10) was chosen for the standards of carbon and nitrogen isotope ratio analysis. Milli-Q water (18.2 MΩ cm) was used in the whole experiment. Other chemicals used in the present work were of analytical grade and purchased from Guangzhou Chemical Reagent Factory (Guangzhou, China).
Fifty-six Tetra Pak milk samples originating from four continents including Australia and vicinity (fifteen samples mainly obtained from Australia and New Zealand), Europe (fourteen samples mainly obtained from Germany and France), North America (twelve samples mainly obtained from the United States) and Asia (fifteen samples mainly obtained from China) were collected in April and May of 2015. Additionally, four milk samples, labeled as samples #1 to #4, were provided by a dairy manufacturer in Guangzhou, China to conduct the stability experiments.
Instrumentation
An MS3 basic vortex mixer (IKA GmbH, Germany) was used for vortexing. A 5804R centrifuge (Eppendorf Corp., Germany) was used to extract protein from the milk samples. The stable carbon and nitrogen isotopic compositions of the protein extracted from the milk samples were determined by performing elemental analyzer-isotope ratio mass spectrometry (EA-IRMS) using a DELTA V PLUS IRMS (Thermo Electron Corporation) interfaced with a Flash 2000 element analyzer (Thermo Electron Corporation). All samples and standard materials were measured after the balance of reference gases (CO2 and N2). The standard deviations (SD) were less than 0.06‰ (n = 10) and 0.08‰ (n = 10) for CO2 and N2, respectively. A Milli-Q A10 gradient system (Millipore Co., Bedford, USA) was used to produce Milli-Q water (18.2 MΩ cm).
Sample preparation
For each experiment, 10 g of the milk sample, 4 mL of distilled water and 4 mL of a 6% hydrochloric acid solution were placed in a 50 mL centrifuge tube and vortexed. Then the tube was swirled in a water bath at 80 °C until visible floc formed and clear supernatant was observed. If no visible floc formed, or if the supernatant remained turbid, acid was added in 2 mL increments with heating repeated between additions. The tube was centrifuged at 4000 rpm and the supernatant was removed. The precipitate was washed by combining it with 50 mL of Milli-Q water, and the resulting mixture was vortexed and then centrifuged at 4000 rpm, after which the supernatant was removed. This procedure was repeated at least five times until the supernatant was clear. The precipitated protein was freeze-dried and ground to a fine powder prior to EA-IRMS analysis. As for the stability experiments, immediately after sample #1 and sample #2 were produced, portions of each of these two samples were stored at room temperature for three, six, nine and twelve months. Portions of sample #3 and sample #4 were stored at 4 °C, 20 °C, and 37 °C for three and six months, respectively. The protein extracted from the milk samples stored for various durations and at various temperatures were prepared according to the method described above, and their stable carbon and nitrogen isotopic compositions were analyzed using EA-IRMS.
Standards
The δ notation was used to describe the isotopic difference between the sample and an international standard, and was defined according to the formula |
δ (‰) = (Rs − Rstd)/Rstd × 1000
| (1) |
where Rs is the isotope ratio (i.e., 13C/12C, 15N/14N) of the sample, and Rstd is that of the reference material. Variations in stable isotope ratios were reported as parts per thousand (‰) deviations from internationally accepted standards: with the Vienna Pee Dee Belemnite (VPDB) standard used for carbon, and atmospheric nitrogen (air) for nitrogen. Each sample was analyzed three times and the mean value was calculated and reported. The analysis was repeated if the difference between the two values was greater than 0.200‰ for both δ13C and δ15N values. In addition, the values were referenced against reference gases (N2, CO2) previously calibrated against International Standards (IS) from the International Atomic Energy Agency (IAEA). Moreover, for each run, at least one in-house standard was analyzed to check the accuracy of the analysis.
Measurement of stable carbon and nitrogen isotope ratios
For each experiment, a mass of approximately 1 mg of the powdered protein extracted from the milk sample was placed into a small tin capsule (3 mm × 2 mm × 5 mm). Then the capsule was folded and compressed to contain the sample and minimize any air present. The prepared protein of the milk sample was introduced into the elemental analyzer using an auto-sampler and the stable carbon and nitrogen isotope ratios were measured by performing EA-IRMS using the previously published one-step method.5 The stable carbon and nitrogen isotopic compositions were recorded in the delta (δ) notations relative to the VPDB standard and the air standard, respectively. Due to the increased amount of sample, the dilutor was switched on just prior to the elution of the CO2 peak and switched off immediately afterwards to prevent the detector from being overloaded with CO2. The CO2 reference gas and N2 reference gas were calibrated against the casein standard.7,8 Each sample was analyzed a minimum of three times and the mean value was calculated and reported. A chromatogram of the protein extracted from a milk sample and measured using the one-step method is shown in Fig. 1.
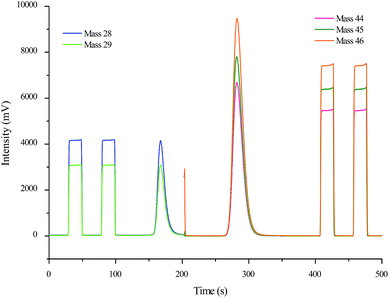 |
| Fig. 1 Chromatogram of the protein extracted from a milk sample acquired by performing EA-IRMS using the one-step method. | |
Statistical analysis
Data were analyzed using SPSS (SPSS Inc., Chicago, IL, USA) and presented as mean ± SD with triplicates. Significance was determined at P < 0.05 by carrying out an analysis of variance (ANOVA) followed by a Duncan's least significant difference test.
Results and discussion
Effects of different storage conditions on the δ13C and δ15N values of protein extracted from milk
δ13C and δ15N values of the protein extracted from the milk samples stored at room temperature for three, six, nine and twelve months are presented in Table 1. From the results, there was no significant (P > 0.05) difference between the δ15N values of extracted proteins stored for the various durations and that for the control except for the extracted proteins stored for three months. As for the δ15C values, no significant (P > 0.05) differences were observed between extracted proteins stored for different durations and the control. These results confirmed the stability of the isotopic compositions in the extracted protein of milk stored for various durations. The δ13C value has been reported to exhibit a strong influence on the ecology, and δ15N in plants has been reported to be primarily controlled by regional agricultural practices.24 Moreover, the δ13C values in plants were previously reported to be correlated with the ratio of intercellular CO2 to CO2 from the environment.25 The same researchers also demonstrated that the association of higher altitude with lower concentrations of atmospheric CO2 may result in different intensities of physiological processes and different δ13C values in wheat and other plants. It has been reported that the nitrogen cycling processes played a strong role in the overall relationship between δ15N values in soil and plants, and soil containing nitrogen sources was the main source of nitrogen for plants.26 Moreover, nitrogen cycling processes were reported to play a strong role in the overall relationship between δ15N values in soil and plants.26 Therefore, a range of factors including latitude, climate, and agricultural practices could affect the degree of fractionation and further influence the stable isotopic compositions in plants and animals, and even in dairy products and some other foods.
Table 1 δ13C and δ15N values of the protein extracted from milk stored at room temperature for different numbers of months (n = 6)a
Storage time (months) |
Control (0) |
3 |
6 |
9 |
12 |
The data are expressed as means ± SD with triplicates. Values within the same row with different letters are significantly different (P < 0.05); otherwise values are not significantly different (P > 0.05). |
Sample #1 |
δ13C value (‰) |
−15.63 ± 0.12a |
−15.78 ± 0.09a |
−15.46 ± 0.10a |
−15.66 ± 0.15a |
−15.51 ± 0.14a |
δ15N value (‰) |
4.56 ± 0.21a |
4.70 ± 0.18b |
4.60 ± 0.12ab |
4.52 ± 0.24a |
4.58 ± 0.11a |
Sample #2 |
δ13C value (‰) |
−16.24 ± 0.15a |
−16.12 ± 0.22a |
−16.30 ± 0.16a |
−16.22 ± 0.13a |
−16.31 ± 0.10a |
δ15N value (‰) |
4.45 ± 0.18a |
4.41 ± 0.16a |
4.48 ± 0.15a |
4.51 ± 0.16a |
4.44 ± 0.20a |
We also investigated the effect of storage temperature on the δ13C and δ15N values of the protein extracted from the milk. From the results (Table 2), no significant (P > 0.05) difference was observed amongst the δ13C and δ15N values for milk stored at 4 °C, 20 °C and 37 °C. Hence, results about the variation of δ13C and δ15N values of the samples stored under different conditions demonstrated the stability of stable isotopic compositions in the extracted protein of milk and their potential as “fingerprints” in tracing the geographical origin of milk stored under different conditions.
Table 2 δ13C and δ15N values of the protein extracted from milk stored at 4 °C, 20 °C and 37 °C for three and six months (n = 6)a
Storage temperature (°C) |
4 |
20 |
37 |
The data are expressed as means ± SD with triplicates. Values within the same row with different letters are significantly different (P < 0.05), otherwise, values are not significantly different (P > 0.05). |
Sample #3 (3 months) |
δ13C value (‰) |
−16.67 ± 0.14a |
−16.58 ± 0.13a |
−16.63 ± 0.10a |
δ15N value (‰) |
4.41 ± 0.18a |
4.78 ± 0.15b |
4.46 ± 0.12a |
Sample #4 (6 months) |
δ13C value (‰) |
−16.01 ± 0.09a |
−16.12 ± 0.21a |
−16.10 ± 0.08a |
δ15N value (‰) |
4.68 ± 0.20a |
4.72 ± 0.17a |
4.62 ± 0.21a |
δ13C analysis of the extracted proteins of fifty-six milk samples from four continents
The δ13C values of the extracted proteins of milk originating from four different continents are shown in Fig. 2. The δ13C values of the extracted proteins from Australia (and New Zealand) were observed to be in the range −29.36 to −27.28‰, with the average value being −28.76‰, while those from Asia (specifically, China) were found to be in the range −16.71 to −15.02‰, with the average value being −15.99‰. The stable isotopic compositions of cow tissues and even milk from cows have been reported to mirror the stable isotopic compositions of foods they eat and ingest.15,21 That is to say, the stable isotopic compositions of milk from cows with different pastures are directly determined by the stable isotopic compositions of the different geographical origins. Some scientists have reported that δ13C values of full honey and its protein fraction could be effectively used to identify adulteration of honey.10 This ability is due to honey preferring pollen from C3 plants while the most common adulterations of honey mainly use the C4 sugar addition.9 The carbon isotopic composition of plant materials strongly depends on the type of carbon fixation process used, be it the C3, C4 or crassulacean acid metabolism (CAM) cycle.27,28 Different photosynthesis pathways present biological discrimination effects on the carbon fixation process, and hence yield different 13C/12C ratios in different kinds of plants; accordingly, the δ13C values of plants using the C3 cycle and C4 cycle have been shown to range from −22‰ to −33‰ and −10‰ to −20‰, respectively, while the range of δ13C values of plants using the CAM cycle has been shown to be wider.5,24,27 Indeed, as shown in Fig. 2, the milk samples originating from cows in Asia, grazing on pastures using the C4 cycle, yielded δ13C values between −10‰ and −20‰. Samples originating from Australia (and New Zealand) were derived from cows grazing on pastures using the C3 cycle. Unfortunately, it was difficult to use δ13C values to distinguish the extracted proteins of milk from Europe from those of milk from America since their δ13C values overlapped, i.e., they ranged from −21.36‰ to −21.02‰ and from −22.98‰ to −21.52‰, respectively (Fig. 2). Thus, further statistical investigations based on a larger number of samples from these regions or measuring other isotopic compositions are required.
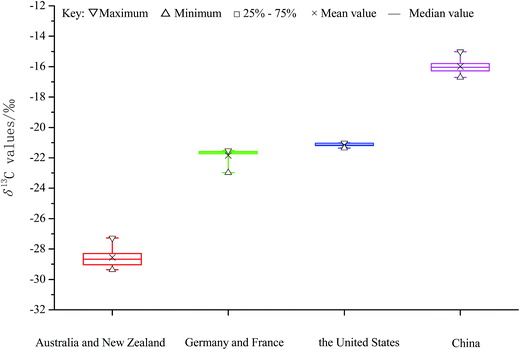 |
| Fig. 2 δ13C values of the protein fractions extracted from fifty-six milk samples of different continents by geographical origin. | |
δ15N analysis of the extracted proteins of fifty-six milk samples from four different continents
The mean δ15N values of the extracted proteins of fifty-six milk samples from the four different continents were calculated to be between 4.55‰ and 5.80‰, with the highest, second-highest, third-highest, and lowest values for Australia (with New Zealand), Europe, America, and Asia, respectively (Fig. 3); this order was completely opposite that of the δ13C values of milk (Fig. 2) whose highest, second-highest, third-highest, and lowest values were for Asia, America, Europe, and Australia (with New Zealand), respectively. Moreover, as shown in Fig. 3, the ranges of the δ15N values were quite different for the samples from the different continents, except for those from America and Asia: the δ15N values ranged from 5.51‰ to 6.02‰ for Australia (with New Zealand), 5.01‰ to 5.39‰ for Europe, 4.57‰ to 4.72‰ for America, and 4.40‰ to 4.71‰ for Asia group. A P-value analysis indicated that there were significant differences in the δ15N values between these four continents (P < 0.05). As can be seen in Fig. 3, the samples from Australia (with New Zealand) and those from Europe could be easily distinguished from each other and the other groups based on the δ15N values, but unfortunately, there was a large overlap between the δ15N values of the samples from America and those from Asia. In addition, a broad distribution of δ15N values was observed for the samples from Australia (with New Zealand) and from Europe, and the standard deviations for these two groups were found to be even bigger than those for the other two groups. The broad distributions of δ15N values for the samples from Australia (with New Zealand) and from Europe may be explained by noting that the sampling in Australia and New Zealand, and in Germany and France, did not cover all the growing regions but only representative locations that were relatively far from each other geographically.
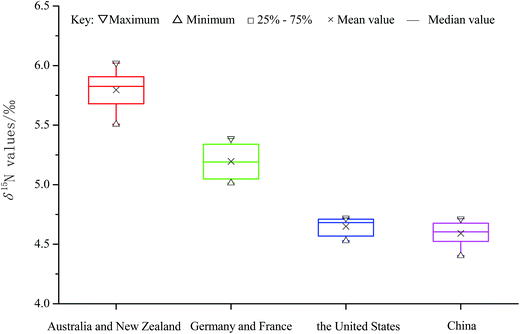 |
| Fig. 3 δ15N values of the protein fractions extracted from fifty-six milk samples of different continents by geographical origin. | |
It was previously reported by researchers that the geographical origin of pure milk could be identified from the δ15N and δ13C values of the extracted protein, or from the δ2H, and δ18O values of milk water; they also found that the δ13C, δ15N, δ2H and δ18O values taken together could indicate the detailed regions from which the pure milk originated.16 In addition, the nitrogen isotopic composition in plants was reported to be influenced by the intensity of agricultural use,16 the climate conditions, and fluctuations in temperature and precipitation.24 Additionally, many studies reported the δ15N values in plants to be highly related to the fertilizer used and the fertilization conditions where the plants grow.2,24,25,29 It has also been reported that the synthetic nitrogen fertilizers generally have δ15N values between −4‰ and 4‰ while the organic fertilizers have higher δ15N values ranging from 2 to 30‰, and that different synthetic nitrogen fertilizers could show different δ15N values.27,30
The distribution of carbon and nitrogen isotopic compositions of milk from different continents
A two-dimensional distribution diagram of carbon and nitrogen isotopic compositions of the extracted proteins of milk samples from different regions is shown in Fig. 4. These distributions for the milk samples from the different continents concentrated in different parts of the plot. Moreover, the isolation of these concentrated regions was also found to be very significant. It has been reported in a previous work that the geographical origin of wheat from different regions could be easily identified by analyzing the two-dimensional distribution diagram based on the δ13C and δ15N values of wheat.4 Moreover, stable isotopic composition analysis combined with a pentagonal radar plot,31 3D scatter plot,16 and LDA32,33 were successfully adopted in tracing the geographical origin of rice, wheat and some other foods. So, analyzing δ13C and δ15N values could potentially be an interesting and rapid way of tracing the origin of a milk sample and hence maintain the normal operation of the milk market all over the world.
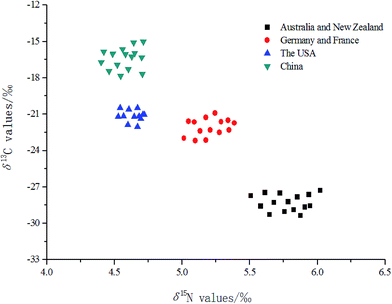 |
| Fig. 4 The distribution of milk samples originating from four different continents based on the stable carbon and nitrogen isotopic compositions. | |
Conclusions
We determined stable carbon and nitrogen isotopic compositions (δ13C, δ15N values) of extracted proteins from fifty-six milk samples from four continents (from several countries including Australia and New Zealand, Germany and France, the United States, and China), in order to develop a method to determine the geographic origin of milk. We also investigated the effects of storage time and temperature on δ13C and δ15N values of the milk and the results indicated that there were no significant differences (P > 0.05) for δ13C and δ15N values of the samples stored under different conditions. This result confirmed the stability of isotopic compositions in the extracted protein of milk and their potential use as “fingerprints” of the geographical origin of milk. We also found that there were significant differences between the mean δ13C and δ15N values of the sample originating from different continents. The highest, second-highest, third-highest, and lowest δ15N values were for the samples from Australia and New Zealand, Germany and France, the United States, and China, respectively; this trend was the reverse of that of the δ13C values, for which the highest, second-highest, third-highest, and lowest values were for samples from China, the United States, Germany and France, and Australia and New Zealand, respectively. It is clear from the obtained results that the δ13C and δ15N values of analytes could potentially be used for a rapid and routine determination of the origin and species of milk. However, it is still uncertain whether the observed difference in isotopic compositions is generally applicable to all milk. A further statistical investigation based on a larger number of samples is thus necessary.
Acknowledgements
This work was financially supported by the Major Special Project of Industry-University-Research Collaborative Innovation of Guangzhou City (201508020086) and the Special Fund Project of Application-oriented for Science and Technology, Research and Development of Guangdong Province (2015B020230001). The authors would also like to thank all of the workers carrying out sampling and sample preparation, and taking measurements. Additionally, the authors gratefully acknowledge the Guangzhou Quality Supervision and Testing Institute for providing the real milk samples from all over the world.
References
- H. Zhao, B. Guo, Y. Wei and B. Zhang, J. Cereal Sci., 2013, 57, 391–397 CrossRef CAS.
- S. Kelly, M. Baxter, S. Chapman, C. Rhodes, J. Dennis and P. Brereton, Eur. Food Res. Technol., 2002, 214, 72–78 CrossRef CAS.
- F. Camin, K. Wietzerbin, A. B. Cortes, G. Haberhauer, M. Lees and G. Versini, J. Agric. Food Chem., 2004, 52, 6592–6601 CrossRef CAS PubMed.
- D. Luo, H. Dong, H. Luo, Y. Xian, J. Wan, X. Guo and Y. Wu, Food Chem., 2015, 174, 197–201 CrossRef CAS PubMed.
- Y. Wu, D. Luo, H. Dong, J. Wan, H. Luo, Y. Xian, X. Guo, F. Qin, W. Han, L. Wang and B. Wang, Food Chem., 2015, 174, 553–557 CrossRef CAS PubMed.
- R. G. Crittenden, A. S. Andrew, M. LeFournour, M. D. Young, H. Middleton and R. Stockmann, Int. Dairy J., 2007, 17, 421–428 CrossRef CAS.
- C. M. Almeida and M. T. S. D. Vasconcelos, J. Anal. At. Spectrom., 2001, 16, 607–611 RSC.
- A. I. Cabañero, J. L. Recio and M. Rupérez, J. Agric. Food Chem., 2006, 54, 9719–9727 CrossRef PubMed.
- H. Dong, D. Luo, Y. Xian, H. Luo, X. Guo, C. Li and M. Zhao, J. Agric. Food Chem., 2016, 64, 3258–3265 CrossRef CAS PubMed.
- D. Luo, H. Luo, H. Dong, Y. Xian, X. Guo and Y. Wu, Food Analytical Methods, 2016, 9, 255–262 CrossRef.
- Y. Chen, D. Kong, L. Liu, S. Song, H. Kuang and C. Xu, Food Analytical Methods, 2016, 9, 905–914 CrossRef.
- F. Guyon, P. Auberger, L. Gaillard, C. Loublanches, M. Viateau, N. Sabathié, M.-H. Salagoïty and B. Médina, Food Chem., 2014, 146, 36–40 CrossRef CAS PubMed.
- E. Jamin, F. Martin, R. Santamaria-Fernandez and M. Lees, J. Agric. Food Chem., 2005, 53, 5130–5133 CrossRef CAS PubMed.
- L. Pillonel, R. Badertscher, M. Casey, J. Meyer, A. Rossmann, H. Schlichtherle-Cerny, R. Tabacchi and J. O. Bosset, Int. Dairy J., 2005, 15, 547–556 CrossRef CAS.
- A. Rossmann, Food Rev. Int., 2001, 17, 347–381 CrossRef CAS.
- D. Luo, H. Dong, H. Luo, Y. Xian, X. Guo and Y. Wu, Food Analytical Methods, 2016, 9, 437–442 CrossRef.
- J.-P. Renou, C. Deponge, P. Gachon, J.-C. Bonnefoy, J.-B. Coulon, J.-P. Garel, R. Vérité and P. Ritz, Food Chem., 2004, 85, 63–66 CrossRef CAS.
- D. Luo, H. Dong, Y. Xian, Y. Wu and X. Zeng, Food Analytical Methods, 2016, 9, 2400–2405 CrossRef.
- T. Nietner, S. A. Haughey, N. Ogle, C. Fauhl-Hassek and C. T. Elliott, Food
Res. Int., 2014, 60, 146–153 CrossRef CAS.
- B. E. Kornexl, T. Werner, A. Roßmann and H.-L. Schmidt, Z. Lebensm.-Unters. -Forsch. A, 1997, 205, 19–24 CrossRef CAS.
- A. Rossmann, G. Haberhauer, S. Hölzl, P. Horn, F. Pichlmayer and S. Voerkelius, Eur. Food Res. Technol., 2000, 211, 32–40 CrossRef CAS.
- D. Kong, L. Liu, S. Song, H. Kuang and C. Xu, Food Agric. Immunol., 2016, 27, 841–854 CrossRef CAS.
- Y. Chen, L. Liu, S. Song, H. Kuang and C. Xu, Food Agric. Immunol., 2016, 27, 830–840 CrossRef.
- S. Kelly, K. Heaton and J. Hoogewerff, Trends Food Sci. Technol., 2005, 16, 555–567 CrossRef CAS.
- H. Liu, B. Guo, Y. Wei, S. Wei, Y. Ma and W. Zhang, Food Chem., 2015, 171, 56–61 CrossRef CAS PubMed.
- D. M. Vallano and J. P. Sparks, Oecologia, 2013, 172, 47–58 CrossRef PubMed.
- A. Guler, H. Kocaokutgen, A. V. Garipoglu, H. Onder, D. Ekinci and S. Biyik, Food Chem., 2014, 155, 155–160 CrossRef CAS PubMed.
- S. Wang, Q. Guo, L. Wang, L. Lin, H. Shi, H. Cao and B. Cao, Food Chem., 2015, 172, 669–674 CrossRef CAS PubMed.
- X. Liu, B. Guo, Y. Wei, J. Shi and S. Sun, Food Chem., 2013, 140, 135–140 CrossRef CAS PubMed.
- A. S. Bateman and S. D. Kelly, Isot. Environ. Health Stud., 2007, 43, 237–247 CrossRef CAS PubMed.
- Y. Suzuki, Y. Chikaraishi, N. O. Ogawa, N. Ohkouchi and T. Korenaga, Food Chem., 2008, 109, 470–475 CrossRef CAS PubMed.
- H. Zhao, B. Guo, Y. Wei and B. Zhang, J. Agric. Food Chem., 2012, 60, 10957–10962 CrossRef CAS PubMed.
- C. Li, H. Dong, D. Luo, Y. Xian and X. Fu, Food Analytical Methods, 2016, 9, 1512–1519 CrossRef.
Footnote |
† Current address: No. 381, Wushan Road, Tianhe District, Guangzhou, China, Tel: +86-20-87113843; Fax: +86-20-87113843. |
|
This journal is © The Royal Society of Chemistry 2017 |
Click here to see how this site uses Cookies. View our privacy policy here.