DOI:
10.1039/C6RA26899D
(Paper)
RSC Adv., 2017,
7, 8952-8962
The hematinic effect of Colla corii asini (Ejiao) using 1H-NMR metabolomics coupled with correlation analysis in APH-induced anemic rats†
Received
16th November 2016
, Accepted 19th January 2017
First published on 30th January 2017
Abstract
Colla corii asini (Ejiao), a gelatin-like food and drug obtained from Equus asinus Linnaeus, has been widely used in clinical hematic antianemic therapy for more than 2000 years. The elucidation of the characteristic metabolic alterations achieved during its treatment of anemia is critical to the screening of the biomarkers involved in anemia and improving the understanding of the hematinic effects of Ejiao. This work presents the first 1H-NMR-based metabolomics coupled with a correlation analysis study of the characteristic metabolic profiles of APH-induced anemic rats subjected to Ejiao treatment. Significant differences in the metabolic profiles of the rats in the control, blood deficiency and treatment groups were observed, and the identified serum biomarkers including arginine, aspartate, choline and dimethylglycine, could be used for the diagnosis of anemia. The results also showed that Ejiao may play a hematinic role through regulating the lipid and lipoprotein metabolism, energy metabolism, gut microflora and amino acid metabolism, reflecting the multi-target therapeutic effect of traditional Chinese medicine, which may be helpful for understanding the hematinic mechanism of Ejiao.
Introduction
Anemia, the most common blood disease, is characterized by a decreased quantity of red blood cells (RBC), often accompanied by diminished hemoglobin levels or altered red blood cell morphology, and affects up to 32.9% of the general population based on epidemiological investigation.1 There are three causes which lead to anemia, such as reduced erythropoiesis, excessive destruction of red blood cells and hemorrhage, where excessive destruction of red blood cells, mainly referring to hemolytic anemia, has been little investigated. Although several factors are proposed to be involved in hemolytic anemia, the systemic and characteristic metabolic alterations remain unclear.
Some traditional Chinese medicine (TCM) has good curative effects on anemia, such as Colla corii asini, Angelica sinensis, Fallopia multiflora, Paeonia lactiflora Pall etc. Colla corii asini (Ejiao), a gelatin-like preparation obtained through stewing and concentrating material from Equus asinus Linnaeus, is a health-care food and TCM that has been widely used in life-nourishing and clinical antianemic therapy for more than 2000 years.2,3 The main components were amino acids, proteins/gelatins, polysaccharides, volatile substances, inorganic substances, etc.4 Previous studies mainly research the hematinic effects of Ejiao on the following aspects: enhances the function of internal free radical scavenging system, facilitating the absorption and utilization of iron, improving bone marrow hematopoietic microenvironment, stimulating the expression of IL-1p, IL-3, IL-6, SCF and GM-CSF, etc.5,6 However, to the best of our knowledge, no metabolomics study of the therapeutic effect of Ejiao in APH-induced anemic rats based on NMR metabolomics has been reported.
Metabolomics, a powerful top-down systems biological approach, is capable of simultaneously measuring global metabolic compositions in any biosystem chosen for a study.7 As an effective analytical technology, metabolomics has been used in disease diagnosis and monitor, toxicity study, environment or pharmaceutical interventions.8–10 Nuclear magnetic resonance spectroscopy (NMR), gas chromatography-mass spectrometry (GC-MS), and liquid chromatography-mass spectrometry (LC-MS) were commonly used in metabolomics study.11 NMR-based metabolomics has the advantages of fast, effective and non-destructive for studies on the metabolic changes in biofluids.12
Therefore, the present work describes an untargeted nuclear magnetic resonance spectroscopy-based metabolomics study of serum and urine collected from healthy rats, anemic rats and anemic rats treated with Ejiao. Furthermore, differential metabolite changes measured in serum are correlated here to those observed in urine, a novel approach enabling a more complete two-biofluid metabolic picture to be obtained. Similarly, blood routine parameter were also linked up to differential metabolite in serum and urine. And this results here constitutes an important step toward the understanding the pathogenesis of anemia and the effect of Ejiao.
Materials and methods
Chemicals
Acetyl phenylhydrazine (APH) was purchased from Sangon Biotech (Shanghai, China) Co., Ltd. D2O was purchased from Norell (Landisville, USA). Ejiao purchased from the store were produced in Baoding Saixing Ejiao Co., Ltd. (Hebei, China, license number is 121003), which production method is in term with the criteria of Chinese Pharmacopoeia (2015 edition). Lvjiaobuxue Granule was obtained from Jiuzhitang Co., Ltd. (Hunan, China, license number 201311023).
The main content of four major amino acid (L-hydroxyproline, glycine, alanine and proline) in Ejiao was determined by high performance liquid chromatography according to the criteria of Chinese Pharmacopoeia (2015 edition) are summarized in ESI (Fig. S1, S2 and Table S1†).
Animals
SPF-grade male Sprague–Dawley (SD) rats (180–220 g) were obtained from Vital River Laboratory Animal Technology Co., Ltd. (Beijing) (license number SCKX-2012-0001) and were allowed to adapt to their new environment for 1 week before the experiments started. All animals were housed 5 per cage under controlled breeding room conditions (temperature: 24 ± 1 °C, humidity: 60 ± 5%, 12 h light/dark cycle, lights on at 8:00 a.m.) with free access to food and water. All animal experiments were carried out in accordance with the National Guidelines for Experimental Animal Welfare (MOST, PR China, 2006), which had full accreditation from the Association for Assessment and Accreditation of Laboratory Animal Care International (AAALAC Intl.). This study was approved by the Committee on the Ethics of Animal Experiments of Shanxi University. Maximal effort was made to minimize animal suffering and the number of animals necessary for the acquisition of reliable data.
Treatment
Animals of similar body weight were randomly divided into one normal control group and five APH-induced groups: (1) control group (CG); (2) model group (MG); (3) low dose of Ejiao group (EJ-L); (4) moderate dose of Ejiao group (EJ-M); (5) high dose of Ejiao group (EJ-H); and (6) Lvjiaobuxue Granules (LG), each group was consists of 10 rats. Rats in the MG and the CG were daily administered a 0.5% CMC-Na suspension, the rats in EJ-H, EJ-M, or EJ-L were administered an Ejiao (4 g kg−1, 2 g kg−1, 1 g kg−1) 0.5% CMC-Na suspension daily, and the rats in the LG were administered an Lvjiaobuxue Granule (0.8 g kg−1) 0.5% CMC-Na suspension daily. The five APH-induced groups were subcutaneously injected with 2% APH on the 1st day (100 mg kg−1), 4th day (50 mg kg−1) and 7th day (50 mg kg−1). The treatment lasted for 21 days, and the rats' body weights were recorded every three days.
Blood routine test
Orbital blood was collected into a 1 mL tube with EDTA within an hour after the last treatment on the 21st day. An analysis of the blood was carried out on an animal blood analyzer (HEMAVET950) using appropriate kits with the following blood routine parameters (BRP): red blood cell count (RBC), red cell distribution width (RDW), red blood cell volume (HCT), mean red cell volume (MCV), white blood cell count (WBC), hemoglobin (Hb), platelet count (PLT), mean corpuscular hemoglobin (MCH) and platelet volume (MPV).
Sample collection and preparation
On the 21st day, the animals were sacrificed, and femoral artery blood was collected. The preparation of the samples was conducted as in the previous literature.13,14 450 μL of serum was mixed with 350 μL of D2O, which was then centrifuged at 13
000 rpm for 20 min at 4 °C, and a 600 μL aliquot of the supernatant was added to a 5 mm NMR tube for NMR analysis.
Urine was collected on ice using metabolic cages at the end of the experiment from 8:00 pm to 8:00 a.m. the next day. A total of 200 μL of phosphate buffer (0.2 M Na2HPO4, 0.2 M Na2H2PO4, pH = 7.4) in D2O-containing sodium 3-trimethylsilyl-(2,2,3,3-d4)-1-propionate (TSP, 0.015%) was added to the 500 μL urine sample and centrifuged for 20 min (13
000 min−1, 4 °C) to minimize chemical shift variations. Then, 600 μL of supernatant was transferred to an NMR tube for the next analysis.
NMR spectroscopy
The 1H-NMR spectra of the serum samples were obtained using a Bruker 600 MHz AVANCE III NMR spectrometer (Bruker Biospin, Rheinstetten, Germany) operating at a 1H frequency of 600.13 MHz and a temperature of 298 K. A one-dimensional (1D) Carr–Purcell–Merboom–Gill (CPMG, RD-90(τcp-180-τcp)-acquisition) with water suppression and a total spin–spin relaxation delay of 320 ms was used to attenuate the broad signals from proteins and lipoproteins due to their short transverse relaxation time.15 The 1H-NMR spectrum for each sample consisted of 64 scans requiring 5 min of acquisition time with the following parameters: spectral width = 12
019.2 Hz, spectral size = 65
536 points, pulse width (PW) = 30° (12.7 μs), and relaxation delay (RD) = 1.0 s. The FIDs were Fourier-transformed with LB = 0.3 Hz.
For good signal dispersion and visualization, two-dimensional (2D) NMR spectra were also recorded for the selected samples at 298 K on a Bruker 600 MHz AVANCE III NMR spectrometer, including 1H–1H correlation spectroscopy (COSY) and 1H–13C heteronuclear multiple quantum coherence (HMQC), as previously reported.16 The COSY spectra were acquired with a 1.5 s relaxation delay, and 48 transients were collected into 1024 data points with a spectral width of 12 ppm for both dimensions. For the 2D 1H–13C HMQC spectra, water suppression was achieved using presaturation during a relaxation delay of 1.5 s. For each 2D spectrum, 2048 × 128 data points were collected using 8 scans per increment. The spectral widths were set to 12 and 240 ppm in the proton and carbon dimensions, respectively.
Chemometrics
The statistical results are shown as the mean ± SD using the one-way ANOVA by SPSS 22.0. Values of p < 0.05 were considered to indicate significant differences.
All the spectra were manually corrected for phase and baseline distortions. Creatinine at δ 3.04 was chosen as the reference for serum spectra, and the regions of residual water (δ 4.50–4.85) were excluded. Each spectrum was then segmented at 0.01 ppm intervals across the chemical shift 0.70–9.00, and each data point was normalized to the sum of its row and then exported as a text file for further multivariate statistical analysis.
The spectra of urine were referenced to the chemical shift of TSP at d 0.00 ppm. The NMR spectra (δ 0.72–9.40) were segmented into sections of equal width (0.02 ppm). Spectral regions of δ 4.50–6.22 were deleted to eliminate the effects of the water suppression and urea signals. The resolution spectra of serum and urine were used to construct data matrices and then imported into SIMCA-P 13.0 (Umetrics, Sweden) software for multivariate pattern recognition for multivariate analysis.
The original spectral data from NMR spectroscopy were scaled to a unit variance, which was calculated by z = (x − y)/s. The x variable represents the level of a particular metabolite in one subject, the y variable represents the mean level of this metabolite across all subjects, and the s variable represents the standard deviation of this metabolite. Then, the scaled data matrix was used to prepare a heatmap. A package within the R framework was used to visualize the changes of differentially expressed metabolites in rats suffering from anemia induced by APH and then administered the treatment.
All of the resulting integral data from the 1H-NMR metabolomics analysis were introduced into SIMCA-P 13.0 (Umetrics, Sweden) for multivariate analysis. In the supervised multivariate approach, partial least-squares-discrimination analysis (PLS-DA) was performed to visualize the discrimination between different groups. The quality of the model was validated by the response values of the permutation test and the calculation of R2 (model fitness) and Q2 (predictive ability), in which the class membership was randomly shuffled 200 times. A large R2 (close to 1) and Q2 (Q2 ≥ 0.5) indicate a good model. Another supervised pattern recognition approach using orthogonal projection to latent structures discriminant analysis (OPLS-DA) was then performed to improve the classification of the different groups, as well as for biomarker screening.17 In the S-plot analysis for the OPLS-DA, the further the plot sits from the origin, the greater the contribution of the metabolite to the separation of the groups involved. The variable importance in the projection (VIP) of the established OPLS-DA model analysis is shown using a color scale. To screen the biomarkers involved in anemia and determine the Ejiao effect of enriching the blood, metabolites of VIP > 1 were required. A p value < 0.05 was used to indicate the level of significance for the group separation.
Correlation analysis was performed in R-project 3.2.4 (https://www.r-project.org/) to search for the relationship between metabolites and blood routine parameters (BRP). A correlation coefficient (r) and significance threshold of |r| ≥ 0.5 and p < 0.05 were applied, and correlations were confirmed by scatter plotting each pair of correlated integrals. These pairs were then used to compute a correlation network, using Gephi 0.8.2 software.
Results
Determination of amino acid in Ejiao
Amino acids are the most abundant components of Ejiao. The chemical structures of main 4 amino acid in Ejiao are shown in Fig. S1.† Fig. S2† demonstrates the separation obtained for a mixture of reference standards and typical sample of Ejiao extract. It can be seen from this that a good separation can be achieved using the conditions described. The content of 4 major amino acid in Ejiao are summarized in Table S1.† The content of L-hydroxyproline was not less than 9.0%, glycine was not less than 19.0%, alanine was not less than 7.0%, proline was not less than 10.0% in Ejiao respectively. That meet the standards specified in 2015 edition of China Pharmacopoeia.
Blood routine parameters
The parameters of the peripheral blood were investigated to assess the hematotoxicity of APH. As shown in Table 1, the parameters of MG showed significant decreases (p < 0.05) in RBC, Hb and MCHC and significant increases (p < 0.05) in MCV, HCT, RDW, MCH, PLT, and MPV compared with the CG. These are the main diagnostic criteria for anemia, indicating that the anemia model was successfully induced. Compared with the MG, the RBC and Hb in the EJ-L, EJ-M and LG increased significantly (p < 0.05), and the MCV and MCH decreased markedly (p < 0.05), whereas the other parameters did not change significantly but showed a tendency of recovery. For the EJ-H group, there was no significant difference in any of the parameters. The results suggest that EJ has a good effect on enriching the blood.
Table 1 Changes in indexes of blood routine examination on anemic rats with Ejiao treatment (n = 10)
|
WBC (10−9/L) |
RBC (10−12/L) |
HCT (%) |
RDW (%) |
MCV (fl) |
Hb (g L−1) |
MCHC (g L−1) |
MCH (Pg) |
PLT (10−9/L) |
MPV (fl) |
Significant difference compared with MG (p < 0.05). Significant difference compared with MG (p < 0.01). RBC: red blood cell count, RDW: red cell distribution width, HCT: red blood cell volume, MCV: mean red cell volume, WBC: white blood cell count, Hb: hemoglobin, PLT: platelet count, MPV: platelet volume, MCH: mean corpuscular hemoglobin, MCHC = MCH/MCV. |
CG |
12.0 ± 2.4 |
9.3 ± 0.3b |
83.7 ± 2.6a |
16.8 ± 0.9b |
89.7 ± 4.6b |
180.8 ± 4.7b |
216.2 ± 7.1b |
19.4 ± 0.7b |
608 ± 108.7b |
5.9 ± 0.4a |
MG |
10.3 ± 1.7 |
7.3 ± 0.2 |
88.7 ± 2.7 |
22.9 ± 2.4 |
122.3 ± 6.6 |
156.7 ± 10.6 |
205.1 ± 5.2 |
25.1 ± 1.3 |
642.0 ± 198.3 |
6.5 ± 0.7 |
EJ-H |
10.0 ± 3.2 |
7.3 ± 0.3 |
87.3 ± 3.9 |
23.0 ± 1.2 |
122.0 ± 7.3 |
156.9 ± 16.7 |
203.3 ± 5.6 |
24.8 ± 1.4 |
605.9 ± 167.2 |
6.4 ± 0.7 |
EJ-M |
9.8 ± 2.2 |
8.0 ± 0.4b |
86.6 ± 5.6 |
21.4 ± 3.44 |
107.9 ± 7.5b |
178.6 ± 11.0b |
206.4 ± 5.4 |
22.2 ± 1.3b |
789.6 ± 89.3 |
6.5 ± 0.3 |
EJ-L |
10.5 ± 3.3 |
8.1 ± 0.22b |
88.5 ± 3.6 |
23.4 ± 2.9 |
109.6 ± 4.0b |
179.5 ± 7.2b |
202.9 ± 6.68 |
22.2 ± 1.1b |
749.3 ± 117.5 |
6.2 ± 0.3 |
LG |
8.6 ± 2.2 |
8.3 ± 0.2b |
87.7 ± 3.0 |
20.8 ± 1.4 |
106.3 ± 3.5b |
175.8 ± 3.88b |
200.6 ± 4.7 |
21.1 ± 0.6b |
766.5 ± 233.6 |
6.1 ± 0.7 |
Body weight and viscera indexes
The body weight and viscera indexes can be used to show from a macro perspective whether there are lesions or dysfunctions in a rat's body consistent with anemia resulting from APH administration. Fig. S3† shows that there were significant decreases in the body weights of rats in the five blood deficiency groups compared with CG, suggesting the success of the anemia model and toxicity induced by the APH that was sufficient to cause observable body weight changes. However, there was no significant weight recovery with the Ejiao or LG treatment, although the average weight was higher than in MG. As expected, with the body weight loss, the rats began to show a series of blood deficiency symptoms such as lassitude, listlessness, being easily frightened, and the appearance of paleness in the mouth and ears, especially in the 4 d after the injection of APH.
The viscera indexes are presented in Table S2.† Compared with the CG, a significant increase in the liver index was observed in the MG and EJ-H, suggesting that liver toxicity was caused by APH. The liver index in the LG exhibited a significant decrease compared with both the MG and CG, indicating that Lvjiaobuxue Granules have a protective effect on the liver. The noticeable phenomenon of splenomegaly as measured by the spleen index represents a significant increase in the relative spleen weight in the five anemia groups compared with the CG. As important immune and hematopoietic organs, the significant changes in the liver and spleen index are indirect responses to the weakened hematopoietic and immune functions of the rats.
1H-NMR spectra and pattern recognition analysis of serum and urine samples
Typical 600 MHz 1H-NMR spectra of the serum and urine samples obtained from the CG, MG and EJ-M rats are shown in Fig. 1. The assignments of the endogenous metabolites involved in the 1H-NMR spectra were based on the HMDB database, the 600 MHz library of the Chenomx NMR suite 7.0 (Chenomx, Inc., Edmonton, Canada) and the database collected previously by our laboratory and confirmed by 2D NMR spectra including COSY (Fig. S4 and S5†) and HSQC (Fig. S6 and S7†). Table S3† summarize the identification of the serum and urine metabolites.
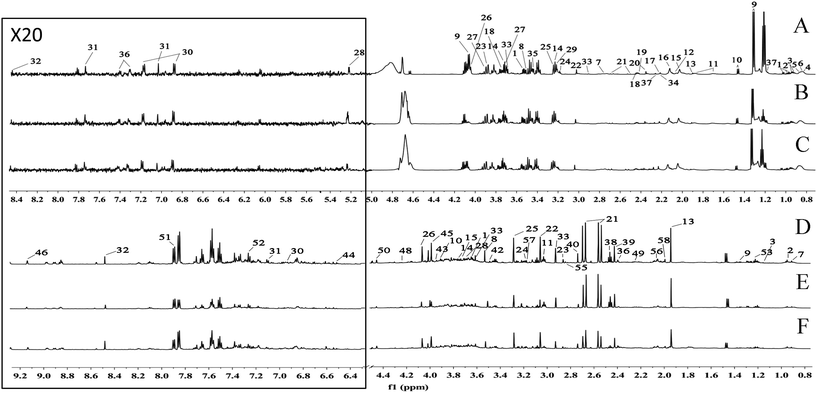 |
| Fig. 1 A total of 58 metabolites were unambiguously assigned, their chemical shifts and peak multiplicities are given in Table S3.† (A) Serum spectra of control group, (B) serum spectra of model group, (C) serum spectra of moderate dose of Ejiao group, (D) urine spectra of control group, (E) urine spectra of model group, (F) urine spectra of moderate dose of Ejiao group. | |
The numerous identified endogenous metabolites in the serum and urine were visualized (Fig. 2). This result mirrored the concentrations of the different metabolites in different biofluids, which are dissimilar under healthy and pathological conditions. To excavate the specific marker metabolites responsible for the changes in the differentially expressed metabolites after the rats were stricken with anemia by APH and then administered the treatment, the supervised multivariate approaches PLS-DA and OPLS-DA were applied. Fig. S8† shows a global view of all the group trends, in which the CG is completely separate from the MG, and EJ-H, EJ-M, EJ-D and LG are separate from the MG, with trends closer to the CG, indicating that the anemia model was successfully established and the Ejiao exhibits an excellent blood-enriching effect. To identify the anemia-specific metabolic changes, the metabolic trajectory between the MG and CG was analyzed and is presented in Fig. 3A and B. The observed completely difference along the t1 axis may be due to the pathogenesis of anemia. The established PLS-DA models were subjected to model validation with permutation testing. As presented in Fig. 3C and D, the permutation tests of the PLS-DA models of the CG and MG showed that the established models had appropriate R2Y and Q2Y intercept values, and the high values obtained indicated that our models were not overfitted and have good predictive ability.18 Furthermore, to minimize the possible contribution of intergroup variability and to further improve the separation between the two groups, orthogonal projection to latent structures discriminant analysis (OPLS-DA), a method that could improve the biomarker discovery effects, was applied to discriminate the CG and MG by their serum and urine. Fig. 3E and F illustrates the corresponding loading S-plot with color-coded VIP. The metabolites contributing to the blood-enriching effect were identified by corresponding S-plots and statistical analysis.
 |
| Fig. 2 Heat maps of normalized integrals of the metabolites resonances in serum and urine with R-project. Rows represent metabolites and columns groups. Integrals are shown using a color scale from minimum (dark blue) to maximum (dark yellow). | |
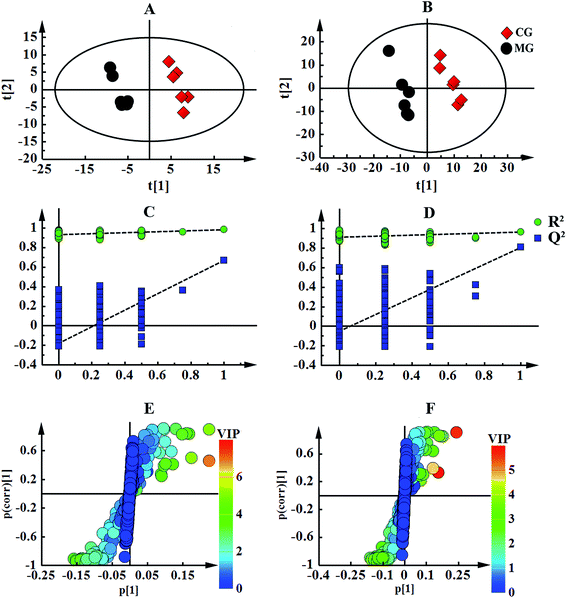 |
| Fig. 3 Pattern recognition of the CG and MG with Simca-P13.0. The PLS-DA score plot trends of CG and MG derived from 1H-NMR serum spectra (A) and urine spectra (B). The model validation of PLS-DA score plot derived from 1H-NMR serum spectra (C) and urine spectra (D). The corresponding S-plot showed with color-coded VIP derived from 1H-NMR serum spectra (E) and urine spectra (F). | |
Quantitative analysis of metabolite profiles
The distinct metabolite variances of the different groups can be attributed to the metabolic changes associated with anemia and the effect of Ejiao. The changes of the key metabolites between MG and CG in the serum are shown in Fig. S9.† Compared with the CG rats, elevated levels of VDL (δ 0.88, m), VLDL (δ 0.89, m), lipids (δ 5.30, br) and acetone (δ 2.23, s) were evident in the serum samples from the MG rats. Additionally, we observed lower levels of arginine (δ 3.25, t), glutamate (δ 2.44, d), creatine (δ 3.04, s), aspartate (δ 3.89, d), choline (δ 3.20, s), α-glucose (δ 3.72, dd), β-glucose (δ 5.23, d) and dimethylglycine (δ 2.92, s) in the serum of the MG compared with the CG. The changes in these endogenous metabolites are considered to be a direct result of the anemia resulting from APH, indicating altered amino acid metabolism, galactose and glucose metabolism, and lipid and lipoprotein metabolism during the development of anemia. Meaningfully, the levels of VDL, VLDL, lipids, acetone, arginine, creatine, aspartate, choline, and dimethylglycine were regulated by Ejiao treatment, suggesting that Ejiao may play enrich the blood function by leveling off the divergences of the metabolites.
Fig. S10† shows the relative integrals of the metabolites in the urine between groups. Similar to the serum metabolites, there were low levels of creatine and choline, and it was interesting that metabolites such as dimethylglycine, α-glucose and aspartate that were reduced in the blood were increased in the urine. In addition, increased levels of valine (δ 3.62, d), N-acetylglutamate (δ 1.99, s), and trimethylamine oxide (TMAO) (δ 3.26, s) and reduced levels of succinic acid (δ 2.40, s) and α-ketoglutarate (δ 2.44, t) were observed in the MG compared with the CG. As in the serum, the effect of Ejiao on anemia via regulating the levels of the disturbed metabolites was reflected in the urinary metabolites.
Correlation analysis of metabolites and blood routine parameters
To investigate the relationship among the metabolites, their level fluctuations in the serum and urine samples from all groups were correlated using Pearson's correlation. The blood routine parameters are well-known for the diagnosis of anemia. An analysis of the correlation between the BRP and identified metabolites can be used to screen for specific biomarkers. Consequently, the significant changes in RBC, Hb, MCV and MCH were observed. The correlation map in Fig. 4, where the circle size and color reflect the correlation strength and sign, shows that besides correlating positively with each other, the RBC presented negative correlations with MCV and MCH and positive correlations with arginine, glutamate, creatine, aspartate, choline, α-glucose, β-glucose and dimethylglycine in the serum. In addition, the fluctuation of the serum creatine levels showed negative correlations with MCV and MCH. In the urine samples, no such correlation was observed with the BRP. These metabolites, which showed |r| ≥ 0.5 and p < 0.05 with BRP in Fig. 5, could serve as potential biomarkers for assessing the anemia and the effect of Ejiao.
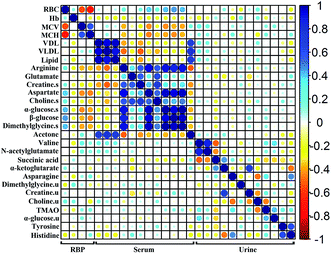 |
| Fig. 4 Correlation analysis of BRP and serum and urinary metabolites with R-project. Red and blue represent positive and negative correlations respectively, the circle size and color scale represents Pearson's correlation coefficients. | |
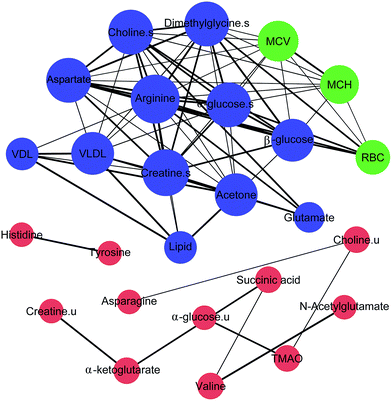 |
| Fig. 5 Correlation network of BRP and serum and urine metabolites based on Pearson's correlation coefficients (|r| ≥ 0.5 and p < 0.05) with Gephi software. Node size denotes the effect size on anemia for each metabolite. Blue, red and green node represent serum metabolites, urine metabolites and BRP. Line thickness reflects the magnitude of the correlation coefficients (|r| ≥ 0.5, thin; 0.6 ≤ |r| < 0.7, medium; |r| ≥ 0.7, broad). | |
A correlation analysis of the serum and urinary metabolites was performed to obtain insight into the pathogenic characteristics and pathways involved in anemia. A positive correlation indicates a relationship between metabolites on the same pathways, whereas a negative correlation suggests that the metabolites may be of different pathways. For example, in the serum metabolites, positive correlations of lipid and lipoprotein (VDL + VLDL) with acetone and negative correlations with arginine, aspartate, choline, α-glucose, β-glucose and dimethylglycine were evident. Furthermore, arginine, creatine, aspartate, choline, α-glucose, β-glucose and dimethylglycine are remarkably related to each other. Moreover, in the urinary metabolites, positive correlations of valine with N-acetylglutamate and α-glucose, creatine with α-ketoglutarate, and asparagine with TMAO, tyrosine and histidine were conspicuous. Additionally, succinic acid was negatively related to valine and N-acetylglutamate, and there was a negative correlation of choline with asparagine (Fig. 4 and 5).
Significant external biofluid correlations (serum/urine) were also observed among the metabolites. Interestingly, the lipids and lipoprotein (VDL + VLDL) in the serum seems to be specifically negatively correlated to histidine in the urine, although their correlation coefficient is close to 0.5. Further, the glutamate in the serum seems to be negatively related to the excretion of creatine and α-ketoglutarate in the urine. The significance test plot is shown in Fig. S11.† The correlations among the serum/serum, urine/urine and serum/urine metabolites suggest anemia-specific alterations in specific metabolic pathways.
Disturbed metabolic pathways in anemic rats
The pathway analysis was performed using the KEGG database (http://www.genome.jp/kegg/pathway.html) and the MetaboAnalyst software (http://www.metaboanalyst.ca/). The results showing alterations in the metabolic pathways involved in anemia and the Ejiao hematinic effects are summarized in Fig. 6. These metabolic changes and the associated pathways provide insights into the mechanisms involved in anemia and the blood-enriching functions of Ejiao.
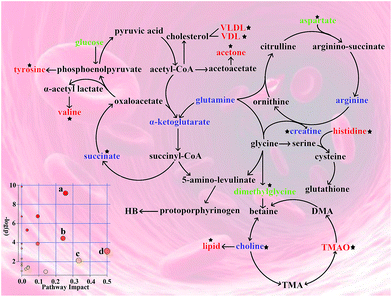 |
| Fig. 6 Disturbed metabolic pathways related to anemia and Ejiao hematinic effects. Metabolites in red, blue and green represent increase, decrease and disturbance levels in serum and urine, respectively, compared with CG. Black stars represent the metabolites that Ejiao could regulating. (a) Alanine, aspartate and glutamate metabolism; (b) histidine metabolism; (c) valine, leucine and isoleucine biosynthesis; (d) phenylalanine, tyrosine and tryptophan biosynthesis (p < 0.01 and impact >0.1). | |
Discussion
Anemia is a serious complication that appears as different types such as iron-deficiency anemia, nutritional megaloblastic anemia, aplastic anemia and hemolytic anemia. Although various factors have been found to influence the progression of anemia, the global metabolic alterations have remained poorly characterized. In this study, we used 1H-NMR and correlative analysis to identify the characteristic metabolic alterations and pathways associated with the therapeutic response in anemia by the traditional Chinese medicine Ejiao in serum and urine samples. The valuable results can provide us with insight into the pathogenesis of anemia and the treatment effect of Ejiao.
Blood routine parameters
APH, a strong oxidizing agent, can produce benzene diazine free radicals and generates the metabolic end product of malonaldehyde (MDA) through membrane lipid peroxidation.19 On the other hand, in the process of the red blood cell membrane metabolism, the protection system of superoxide dismutase (SOD), glutathione (GSH) and glucose 6-phosphatedehydrogenase (G6PD) is destroyed, which leads to hemolytic anemia. In this experimental condition, significant decreases in RBC and Hb and increases in MCV and MCH were observed through the impact of APH, and these altered BRP were clearly regulated by Ejiao treatment (Table 1). The RBC membrane contains an important ATP enzyme that catalyzes the ATP hydrolysis, releases energy, and drives the active transport of Na+ and K+, and APH toxicity may disturb the maintenance of the normal structure and function of the RBCs. Hb is a protein containing iron that easily combines with oxygen in an environment with a high concentration of oxygen and separates from oxygen in a low oxygen concentration environment. Hence, Ejiao plays a role of enriching the blood through the improvement of the oxygen-carrying capacity and bone marrow hematopoietic function. The elevated MCH level suggests an imbalance between RBC and Hb. In addition, changes in the morphology of the RBCs were mirrored by the MCV, symbolizing a megaloblastic appearance that is commonly induced by folic acid and vitamin B12 deficiencies.
Viscera index
Liver, a main hematopoietic organ in the embryonic stage, its hematopoietic function is replaced by bone marrow until adulthood.20 But in some pathological conditions, especially in the state of anemia, liver can restore a certain hematopoietic function. In addition, liver contains rich natural immune cells such as NK, NKT and gamma delta T cells, therefore it considered to be the natural immune organs. Spleen is not only immune organs, but also have a certain relationship with hemopoiesis, it can compensate for production of blood when blood deficiency, resulting in the spleen volume expansion.21 Changes in the weight of immune organs can be an indirect response to the hemopoiesis and the anemia model reliable. EJ-L had an effect on liver index but fail to work on other viscera index may be due to short duration of administration or APH dose-dependent toxicity.
Candidate biomarker screening
Our NMR-based metabolomics analysis revealed several metabolic changes in the serum samples. These characteristic changes could serve as potential biomarkers for the diagnosis of anemia. However, as diagnosis based on the quantification of a large number of metabolites would be implausible, further screening to optimize the metabolite biomarkers is necessary.
Glycosylated Hb, a non-enzymatic condensation product of valine in the Hb β-chain N terminal combined with glucose, is a new diagnostic criterion for diabetes, reflecting the blood glucose levels in the last 8 to 12 weeks. The decreases of α-glucose, β-glucose and hemoglobin can predict anemia that is complicated by abnormal blood glucose, but this is only a complication of anemia and not the main cause, so the α-glucose and β-glucose levels might not be reliable biomarkers of anemia, despite their strong correlations with BRP (r > 0.7). This result also provides us with a warning that anemia may lead to the early onset of diabetes by reducing the value of glycosylated Hb.22 Furthermore, the strong correlations of arginine, aspartate, choline and dimethylglycine with BRP demonstrate that combinations of these metabolites in the serum could be reliable biomarkers for anemia. Additional pharmacological or biochemical studies are needed to test the specificity and sensitivity of these metabolites as biomarkers of anemia.
Lipid and lipoprotein metabolism
The decreased oxygen-carrying capacity of the RBC causes a glucose utilization deficiency, so the synthesis of lipids in the liver provides energy to the organism. Combined with the observed elevated acetone, an important product of fatty acid β-oxidation in the mitochondria, it suggests a deficiency in the energy utilization and the dysfunction of the mitochondria in anemia. Previous studies have reported,23 under the condition of chronic hypoxia that the concentration of erythropoietin (EPO) in the blood was increased, and it participates in mitochondrial biosynthesis by activating the Akt and eNOS pathways. In this experiment, Ejiao could regulate the concentration of lipids/ketones, indicating that the mechanism of Ejiao regulating lipid metabolism disorders associated with anemia is probably the maintenance of the hypoxia adaptive protection by stabilizing the EPO and mitochondrial function. Additionally, as a constituent of the cell membranes and lipoprotein phospholipids, a decreased level of choline and a negative correlation with lipids were observed, providing evidence that the lipid metabolism and membrane fluidity were disrupted.24
The significant correlations of lipids and acetone with lipoprotein (r > 0.9) also provide valuable information on their association with anemia. The observed elevated VDL and VLDL levels could produce Ox-VLDL and Ox-VDL via APH oxidation. Then, lipid peroxidation could damage the unsaturated fatty acids in the erythrocyte membrane, thus causing an abnormal membrane lipid distribution.25 Furthermore, increases in the hematocrit under the condition of anemia initiate pachemia and relative elevations in the blood cholesterol levels. High levels of cholesterol in the erythrocyte membrane decrease its fluidity, making it more susceptible to hemolysis. However, we found that the serum lipoprotein levels of anemic rats were reduced by Ejiao administration, indicating that Ejiao plays a role in enriching the blood through exerting an antioxidant effect. Together, these results indicate that the presence of anemia and the disturbance of the lipid and lipoprotein metabolism do not exist independently but rather influence each other and have a reciprocal causation, although our correlation analysis found only a weak correlation between them.
Energy metabolism
The ability of the RBCs to transport oxygen is greatly reduced in the condition of anemia, and the mature RBCs do not have a TCA cycle. Therefore, RBCs are produced by ATP by way of glycolysis, in which a molecule of glucose produces 2 molecules of pyruvate and 2 molecules of ATP after anaerobic respiration, with the energy produced negligible compared with that by aerobic respiration. Compared with the CG, a significantly decreased level of serum glucose was observed in the MG rats, suggesting that the massive consumption of glucose produced a small amount of energy that accelerated the aging and death of RBCs. Additionally, the levels of serum glucose were not significantly regulated by Ejiao, indicating that there are differences between the pathogenesis of anemia and the treatment mechanism of Ejiao, with the contributions of specific metabolic pathways requiring further research. Our correlation analysis also found that glucose has a negative correlation with acetone (r = −0.69, r = −0.63), which is consistent with the finding that an increase in acetone preserves glucose for the brain and RBCs.
We found that creatine was negatively correlated with MCV (r = −0.61) and MCH (−0.58) in the serum of anemic rats, which suggests that it plays a key role in the progression of anemia. Endogenous creatine is an important compound in vivo that can rapidly synthesize, consume and provide energy when the ATP characteristics of low storage capacity and sluggish synthesis prevent it from satisfying the body's energy requirements. The changes in the relative concentrations of creatine in anemic rats were reversed by Ejiao treatment, confirming the capability of improving the energy metabolism involved in the mechanism of the Ejiao treatment of blood deficiency. Previous reports showed that the concentration of creatine in the RBCs is closely related to the survival time of the RBCs and hemolysis,26 which is in agreement with the results of our correlation analysis. The reduction of the relative concentration of creatine in both the serum and urine also reflects the dysfunction of the renal and glomerular filtration rates under APH-induced anemia.27 Succinate and α-oxoglutarate present a close correlation (r = 0.64), which is in accordance with their both being important intermediate products of the TCA cycle. The results of our analysis showed that there were decreased concentrations of α-oxoglutarate and succinate in the urine samples of anemic mice, reflecting that the TCA cycle is slackened during the stages of anemia. Notably, only the succinate level showed a correction with Ejiao treatment. Therefore, it appears that the treatment mechanism of Ejiao is that pathways other than the TCA cycle, such as the use of specific succinate transporters, are responsible for the changes in the succinate levels and may play a role in determining the disease status.28
Gut microflora
Some methylamine metabolites are important non-perturbing renal osmolytes produced through the degradation of dietary choline to TMA and its di- and monoamine metabolites by the action of gut microflora.29 The decreased concentrations of choline and increased urinary concentration of TMAO and their negative correlation (r = −0.66) suggest a disorder of the gut microflora in anemic rats. Anemia can cause a gut microfloral disturbance, which affects the normal physiological function of the gut. In turn, the generation, transformation and absorption of various related substances (hematopoietic factors) are dependent on the normal physiological function of the gastrointestinal tract. Growing evidence suggests that the gut microflora appear to be governed by a combination of the genotype and diet and thus affects the host's metabolism.30 The megaloblastic appearance mirrored by the MCV in the RBP results again confirmed a problem in the absorption of folic acid or iron in the gut.31 Folic acid can promote the maturation of immature cells in the bone marrow, and as humans and other mammals do not synthesize their own folic acid, they must absorb exogenous folic acid via the intestinal tract.32 In addition, the intestine absorbs iron derived from food or released from the destruction of aging RBCs and transports it to young red blood cells and iron storage tissues by serum ferritin, which combines with protoporphyrin to form ferroheme in the mitochondria, and then the protoporphyrin combines with globin to form Hb. However, reports show that TMAO could reduce the intestinal absorption rate of iron (by more than 70%), consistent with our results that the increased concentration of TMAO in anemic rats caused a gut microfloral disorder, which may affect the absorption of iron. Asymmetric dimethylarginine (ADMA), an endogenous inhibitor of nitric oxide (NO) synthase, is a major precursor of DMA. TMAO generates DMA by enzymatic degradation, so we hypothesize that it may affect the concentration of NO. The endothelial type of NO enzyme is found in the blood cells, and the RBCs may be an important carrier of NO storage, which can significantly improve the red blood cells' deformation capacity to resist breakage.33
These results indicate that the metabolism of intestinal microorganisms is indirectly involved in the development of anemia. Ejiao can regulate the concentrations of choline and TMAO, suggesting a possible mechanism for its function of the stabilization of gut microflora to promote the intestinal absorption of folic acid and iron and the regulation of NO to enhance the deformation ability to resist hemolysis. However, further studies are needed to understand the precise nature of these contributing factors.
Amino acid metabolism
The metabolome view in Fig. 6 shows all matched pathways according to the p-values from pathway enrichment analysis and the pathway impact values from pathway topology analysis, with pathways that have p < 0.01 and an impact value greater than 0.1, including alanine, aspartate and glutamate metabolism; histidine metabolism; valine, leucine and isoleucine biosynthesis; and phenylalanine, tyrosine and tryptophan biosynthesis. Finding metabolite and BRP correlations should provide additional information on the mechanism of anemia and the effect of Ejiao, although the origin of the correlations and the intermetabolite distance in the metabolic space may not be defined without further biochemical studies.
The decreased levels of arginine, glutamate dimethylglycine and aspartate in serum and the elevated levels of valine, N-acetylglutamate, aspartate and dimethylglycine in urine are indicative of amino acid metabolism disorder. These amino acids are mostly neutral amino acids and acidic amino acids that have a polyanionic group structure. The negative ion group has a great influence on the oxygen-binding degree of Hb, promoting the connection between Hb and dextran. Compared with MG, the levels of these amino acids except for glutamate were regulated by Ejiao, suggesting that it could accommodate the affinity between oxygen and hemoglobin and improve the microenvironment of the cells involved in anemia. ALA (δ-aminolaevulinic acid) synthase is the rate-limiting enzyme in the heme synthase, and heme has a feedback inhibition effect on this enzyme. The study shows that the chemical structure of glycine chelate iron is similar to that of the porphyrin pyrrole in the heme,34,35 so as a competitive analogue, it could compete with the ALA synthase, which has an effect on the activity of ALA synthase. Moreover, the imidazole ring in histidine and histidine residues in the protein can promote the activity of the heme peroxidase.36,37 Together, Ejiao reversed the changes in concentrations of glycine and histidine from APH, showing that the absorption of iron and the synthesis of heme were improved by means of Ejiao administration. Tyrosine is a major endogenous peroxidase containing a phenolic hydroxyl, and the presence of tyrosine and its derivatives could reduce oxidative damage in vivo. In addition, it is reported that tyrosine has the function to remove free radicals,38 which confirms its intervention effect on Hb-mediated redox reaction. Arginine, the only precursor of endogenous NO, is one of the most functional amino acids yet discovered,39 with functions including anti-oxidation, regulation of intracellular calcium, and inhibition of mitochondrial permeability. Additionally, glutathione (GSH), a combination of glutamic acid, cysteine and glycine, could protect hemoglobin from hydrogen peroxide and free radical oxidation, so it continues to play a role in maintaining the normal oxygen transport capacity. This altered amino acid profile demonstrates that the oxidative stress induced by APH is involved in the development of anemia. Furthermore, in this study we found that the levels of dimethylglycine, histidine, tyrosine, arginine and glutamate returned to normal after Ejiao treatment, suggesting that Ejiao exerts an important antioxidative effect. Generally, valine, leucine and isoleucine work together to promote the body's normal growth, tissue repair, and regulation of blood sugar and provide energy for the body.40 Compared with the NG, the increased level of valine combined with the disturbance of the aspartate level in the MG reflected liver function failure due to APH.41 In the current investigation, we observed a significant correction of the valine and aspartate levels. Therefore, it is plausible that Ejiao has a hepatoprotective effect and thus indirectly replenishes the blood.
Conclusions
In summary, we integrated information obtained from the 1H-NMR-based metabolomics analysis of serum and urine samples of anemic rats with Ejiao treatment. Combined metabolic alterations and correlation analysis identified arginine, aspartate, choline and dimethylglycine as serum-biomarkers of anemia. Also the results revealed the changed metabolic profiles by APH were reversed by Ejiao, indicating Ejiao may play a hematinic role through regulating lipid and lipoprotein metabolism, energy metabolism, gut microflora and amino acids metabolism.
Acknowledgements
This work was supported by the National Natural Science Foundation of China (No. 81441096), the Science and Technology of Shanxi Province (201603D321077), the Key Laboratory of Shanxi Province (201605D111004) and the Science and Technology Innovation Team of Shanxi Province (201605D131045-18). Furthermore, the full paper was edited for proper English language, grammar, punctuation, spelling, and overall style by one or more of the highly qualified native English speaking editors at American Journal Experts.
References
- N. J. Kassebaum, R. Jasrasaria, M. Naghavi, S. K. Wulf, N. Johns, R. Lozano, M. Regan, D. Weatherall, D. P. Chou, T. P. Eisele, S. R. Flaxman, R. L. Pullan, S. J. Brooker and C. J. L. Murray, Blood, 2014, 123, 615–624 CrossRef CAS PubMed.
- P. Lv, Y. Zhao, F. Qi, X. Zhou, J. You, Y. Qin and Y. Zhang, J. Food Drug Anal., 2011, 19, 123–130 CAS.
- D. Wang, M. Liu, J. Cao, Y. Cheng, C. Zhuo, H. Xu, S. Tian, Y. Zhang, J. Zhang and F. Wang, Biol. Pharm. Bull., 2012, 35, 2128–2132 CAS.
- D. Wang, W. Ru, Y. Xu, J. Zhang, X. He, G. Fan, B. Mao, X. Zhou and Y. Qin, Drug Discoveries Ther., 2014, 8, 201–207 CrossRef CAS.
- D. Wang, M. Liu, J. Cao, Y. Cheng, C. Zhuo, H. Xu, S. Tian, Y. Zhang, J. Zhang and F. Wang, Biol. Pharm. Bull., 2012, 35, 2128–2132 CAS.
- M. Liu, H. Tan, X. Zhang, Z. Liu, Y. Cheng, D. Wang and F. Wang, J. Ethnopharmacol., 2014, 152, 575–584 CrossRef PubMed.
- J. K. Nicholson, J. C. Lindon and E. Holmes, Xenobiotica, 1999, 29, 1181–1189 CrossRef CAS PubMed.
- W. Xu, J. Wu, Y. An, C. Xiao, F. Hao, H. Liu, Y. Wang and H. Tang, J. Proteome Res., 2012, 11, 3423–3435 CrossRef CAS PubMed.
- L. Peiqiu, W. Lai, W. Huifeng, L. Weisheng, W. Yijie, L. Xiaojing, N. Jiazuan and P. Fengkui, J. Rare Earths, 2009, 27, 280–287 CrossRef.
- P. Masson, K. Spagou, J. K. Nicholson and E. J. Want, Anal. Chem., 2011, 83, 1116–1123 CrossRef CAS PubMed.
- J. Chen, B. Shi, H. Xiang, W. Hou, X. Qin, J. Tian and G. Du, J. Pharm. Biomed. Anal., 2015, 115, 150–158 CrossRef CAS PubMed.
- C. Liu, Y. Wu, G. Feng, X. Gao, Y. Zhou, W. Hou, X. Qin, G. Du and J. Tian, J. Affective Disord., 2015, 185, 156–163 CrossRef CAS PubMed.
- B. Shi, J. Tian, H. Xiang, X. Guo, L. Zhang, G. Du and X. Qin, Behav. Brain Res., 2013, 241, 86–91 CrossRef CAS PubMed.
- J. Tian, B. Shi, H. Xiang, S. Gao, X. Qin and G. Du, PLoS One, 2013, 8, e75721 CAS.
- Q. He, H. Tang, P. Ren, X. Kong, G. Wu, Y. Yin and Y. Wang, J. Proteome Res., 2011, 10, 5214–5221 CrossRef CAS PubMed.
- J. Tian, G. Peng, X. Gao, Y. Zhou, J. Xing, X. Qin and G. Du, J. Ethnopharmacol., 2014, 158, 1–10 CrossRef PubMed.
- M. Bylesjo, M. Rantalainen, O. Cloarec, J. K. Nicholson, E. Holmes and J. Trygg, J. Chemom., 2006, 20, 341–351 CrossRef.
- D. Chang, A. Weljie and J. Newton, Leveraging latent information in NMR spectra for robust predictive models, 2007 Search PubMed.
- E. Beutler, Pharmacol. Rev., 1969, 21, 73–103 CAS.
- H. Xu, L. Foltz, Y. Sha, M. Madlansacay, C. Cain, G. Lindemann, J. Vargas, D. Nagy, B. Harriman, W. Mahoney and P. Schueler, Genomics, 2001, 76, 2–4 CrossRef CAS PubMed.
- S. Yun, T. Zhang, M. Li, B. Chen and G. Zhao, Plant Foods Hum. Nutr., 2011, 66, 212–217 CrossRef CAS PubMed.
- C. Jurkovitz, J. Abramson and W. M. McClellan, Curr. Opin. Nephrol. Hypertens., 2006, 15, 117–122 CrossRef PubMed.
- C. Stockmann and J. Fandrey, Clin. Exp. Pharmacol. Physiol., 2006, 33, 968–979 CrossRef CAS PubMed.
- B. Tang, J. Ding, Y. Yang, F. Wu and F. Song, J. Ethnopharmacol., 2014, 153, 511–520 CrossRef PubMed.
- C. Stockmann and J. Fandrey, Clin. Exp. Pharmacol. Physiol., 2006, 33, 968–979 CrossRef CAS PubMed.
- W. Griffiths and M. Fitzpatrick, Br. J. Haematol., 1967, 13, 175–180 CrossRef CAS PubMed.
- J. Baron, X. Cheng, H. Bazari, I. Bhan, C. Lofgren, R. Jaromin, K. Lewandrowski and A. S. Dighe, Am. J. Clin. Pathol., 2015, 143, 42–49 CrossRef PubMed.
- J. Peti-Peterdi, Kidney Int., 2010, 78, 1214–1217 CrossRef CAS PubMed.
- J. Messenger, S. Clark, S. Massick and M. Bechtel, Journal of Clinical and Aesthetic Dermatology, 2013, 6, 45–48 Search PubMed.
- J. Stock, Atherosclerosis, 2013, 229, 440–442 CrossRef CAS PubMed.
- V. Singh, P. Nigwekar, A. Dhyabar, A. Garg, S. Vaidya and N. Lonare, Pravara Medical Review, 2015, 7, 22–24 Search PubMed.
- S. Thakur, B. Rahat, A. Hamid, R. A. Najar and J. Kaur, J. Nutr. Biochem., 2015, 26, 1084–1094 CrossRef CAS PubMed.
- B. Riquelme, P. Foresto, M. D'Arrigo, F. Filippini and J. Valverde, Clin. Hemorheol. Microcirc., 2006, 35, 277–281 Search PubMed.
- I. Saberikia and E. Safaei, Synth. React. Inorg., Met.-Org., Nano-Met. Chem., 2015, 45, 984–987 CrossRef CAS.
- H. Wichi, Food Chem. Toxicol., 1988, 26, 717–723 CrossRef.
- H. Atamna and K. Boyle, Proc. Natl. Acad. Sci. U. S. A., 2006, 103, 3381–3386 CrossRef CAS PubMed.
- H. Atamna, W. H. Frey II and N. Ko, Arch. Biochem. Biophys., 2009, 487, 59–65 CrossRef CAS PubMed.
- C. Nimalaratne, D. Lopes-Lutz, A. Schieber and J. Wu, Food Chem., 2011, 129, 155–161 CrossRef CAS.
- J. Appleton, Alternative Med. Rev., 2002, 7, 512–522 Search PubMed.
- P. L. Campos-Ferraz, T. Bozza, H. Nicastro and A. H. Lancha, Nutrition, 2013, 29, 1388–1394 CrossRef CAS PubMed.
- M. Morgan, J. Milsom and S. Sherlock, Gut, 1978, 19, 1068–1073 CrossRef CAS PubMed.
Footnotes |
† Electronic supplementary information (ESI) available. See DOI: 10.1039/c6ra26899d |
‡ Xiao Zhang is the Co-first author. |
|
This journal is © The Royal Society of Chemistry 2017 |
Click here to see how this site uses Cookies. View our privacy policy here.