DOI:
10.1039/C6RA26737H
(Review Article)
RSC Adv., 2017,
7, 6046-6058
Two decades of new drug discovery and development for Alzheimer's disease†
Received
13th November 2016
, Accepted 16th December 2016
First published on 18th January 2017
Abstract
Alzheimer's disease is a progressive and irreversible neurodegenerative disease, associated with a decreased cognitive function and severe behavioral abnormalities. Due to its complex pathophysiological characteristics, complicated interactions with a large number of genes and proteins, there is still no effective drug treatment of the disease. Amyloid cascade aggregation of senile plaques and hyperphosphorylation of Tau protein to form neurofibrillary tangles are the main pathological features of Alzheimer's disease, other mechanisms, such as oxidative stress, lack of central cholinergic neurotransmitters, inflammatory reaction and toxic metal ions have also been involved. The purpose of this review is to briefly introduce the progress of the development of the therapeutic agents based on their main mechanisms of action.
1. Introduction
Alzheimer's disease (AD), a progressive and irreversible neurodegenerative disease and complicated multi-factorial disorder, is one of the most common forms of dementia.1 It is estimated that there are more than 35 million AD patients worldwide. AD has been recognized as one of the most difficult medical problems with heavy social and economic costs.2 So far, there has been no effective drug or treatment to prevent or reverse the progression of the disease. AD is characterized by progressive memory loss, cognitive impairment and severe behavioral abnormalities. The major pathological features of AD are extracellular aggregation of amyloid β peptide (Aβ) forming senile plaques (SP), the deposits of intracellular neurofibrillary tangles (NFTs).3 Although there is extensive study on the pathogenesis of AD, the exact mechanism of AD is still unknown due to its complex pathophysiological characteristics. Several mechanisms have been proposed attempting to explain the pathogenesis of the disease, the amyloid β (Aβ) cascade,4 the hyperphosphorylated Tau protein,5 oxidative stress,6 deficiency of central cholinergic neurotransmitter,7 inflammation8 and toxic metal ions9 have been involved. Although the pathogenesis of the disease is not clear, new agents associated with the pathological changes of the disease are significantly developed. The purpose of this review is to briefly introduce the progress of the development on the therapeutic agents based on their main mechanisms of action, which hoping to promote the development of the next generation of AD therapeutic drugs.
2. The pathogenesis
2.1. Amyloid cascade hypothesis
One of the main pathological features of AD is the formation of senile plaques (SP), which caused by amyloid beta (Aβ) deposition. Normally, Aβ is soluble small peptides, which is produced by cleavage of the amyloid precursor protein (APP) by the action of α-secretase, β-secretase and γ-secretase.10 The imbalance between β-amyloid (Aβ) production and clearance leads to various types of toxic oligomeric, namely protofibrils, fibrils and plaques depending upon the extent of oligomerization.11 The reason of the formation of Aβ is still unknown, but the sequence, concentration and conditions of stability of Aβ are important factors.12 Some studies suggested that neurotoxicity required assembly of the peptide into oligomers,13 and other evidences suggested that soluble oligomers forms of Aβ could produce more neurotoxicity.14 A thorough study shows that amyloid toxicity associated with both protein-specific and conditional-determined by the function of vascular endothelial growth factor receptor 2 (VEGFR2) loss, which is essential for target protein in a biological context.15 A recent work systematic reviewed of the 25 years of the development and latest findings of the amyloid hypothesis, and elaborated the features of its cell biology and genetics. Suggesting that amyloid dyshomeostasis has emerged as the most extensively validated and compelling therapeutic target.16
2.2. Hyperphosphorylated Tau protein
The neurofibrillary tangles (NFTs) formed by hyperphosphorylated Tau protein are another major pathological feature of AD. Tau protein belongs to the family of microtubule-associated proteins, mainly existing in the axon,17 and its main function is to maintain the stability of microtubules.18 There are several phosphorylation sites on Tau protein, compared with the mature nerve cells, the Tau protein in the growing nerve cells is more likely to be phosphorylated.19 Normally, the phosphorylation and dephosphorylation of Tau protein maintain a dynamic balance, but when the hyperphosphorylated Tau protein aggregates to form a double-helix fiber, it loses the function of connecting and stabilizing microtubules, which leads to the death of neurons.20,21 There has been a long-standing debate over the temporal mechanistic relationship between the two major pathological features of AD, and evidence reports that soluble amyloid beta protein dimers induces Tau protein hyperphosphorylation and neurodegeneration.22
2.3. Oxidative stress hypothesis
Reactive oxygen species (ROS) and reactive nitrogen species (RNS) are produced in many normal and abnormal processes in humans, they play a dual role as both beneficial function of numbers of cellular signaling pathways and deleterious process that can lead to damage of cellular structures (including cell membrane, lipid, protein, and DNA).23 The high oxygen consumption of the brain, which utilizes 20% more oxygen than other mitochondrial respiratory tissues, means that the brain is more vulnerable to oxidative stress.24 Neuron is the basic function unit of the brain, which contains a large number of polyunsaturated fatty acids. It can interact with ROS, leading to the lipid peroxidation reaction and molecular apoptosis,25 in addition, less glutathione in neurons is also one of the causes of oxidative stress injury.26
2.4. Cholinergic hypothesis
Bartus et al. proposed the cholinergic hypothesis that dysfunction of cholinergic activity in the brain of healthy elderly and dementia patients may play a role in the loss of memory and related cognitive impairment, so reconstruction of cholinergic function may be able to reduce the serious lack of cognitive function.27 The activity of the cholinergic system was evaluated by choline acetyl transferase (ChAT) and acetylcholinesterase (AChE) etc.28 The study showed that the Ach, ChAT and AChE in the brain of AD patients showed a continuous decline.29 Acetylcholine (ACh) is neurotransmitters regulating cognitive performance and learning and memory process,30 synthesized by acetyl CoA and choline under the catalysis of ChAT, ACh and its receptor (AChR) combined with transfer nerve impulses, AChE hydrolyzed into acetic acid and choline. Acetylcholine established synaptic contacts in networks of brain cells to remodelling of the cerebral cortical circuits, which will subserve complex cognitive functions.31
2.5. Inflammatory hypothesis
Inflammatory responses in the brain is another pathological characteristic in AD, usually chronic inflammation, main characteristics for a large numbers of mononuclear leucocytes and macrophages in the central nervous system, such as small glial cells.32 Compared with normal subjects, acute phase proteins and proinflammatory cytokines over expression in AD patients brain tissues.33,34 Microglia and astrocytes are the main causes of the inflammatory response, the activated cells produce proinflammatory mediators, such as interleukin 1 beta (IL-1) and interleukin 6 (IL-6) and tumor necrosis factor (TNF alpha), chemotactic factor interleukin 8 (IL-8), macrophage inflammatory protein-1, prostaglandins, leukotrienes, coagulation factor, protease, protease inhibitors;35,36 The production of these substances can kill the neighboring neurons.37
2.6. Metal ion hypothesis
Metal ions play an important role in the maintenance of homeostasis,38 and the relationship between metal ions and neurodegenerative diseases has attracted much attention in recent years.39,40 The brain is rich in metals that act as essential cofactors in metalloproteins to participates in the process of metabolism,41 the concentration of metal ions in the brain is tightly regulated through the blood brain barrier, when the blood brain barrier of metal ion regulation system degradation,42 metal ion transport dysfunction, metal ions (iron, copper, manganese, aluminum, zinc, etc.) begin to affect the oxidative stress response of mitochondria and the wrong folding proteins, and ultimately lead to neurodegeneration.43,44 Studies have indicated that aluminum, zinc, copper and iron can lead to changes in the conformation of the Aβ protein.45 Aluminum can lead to the accumulation of Aβ and Tau protein,46 aluminum and copper are involved in the process of the development of nerve inflammation.47 The increased levels of iron, aluminum and copper in the aged human brain may reflect the relationship between age and neurodegenerative diseases.48
3. Cholinergic drugs
Drachman and Leavitt suggested that memory was associated with the cholinergic system and was age dependent,49 and Bartus27 proposed Alzheimer's cholinergic hypothesis on which the development of cholinergic inhibitors is mainly based.50 Study suggested that the acetylcholine (ACh), neurotransmitters regulating cognitive performance and learning and memory process,51 in the brain of AD patients showed a continuous decline.52 The loss of cholinergic function is related to cognitive impairment and behavioral disorder, and these symptoms can be improved by acetylcholinesterase (AChE) inhibitors or by modulating other cholinergic receptors, such as muscarinic and nicotinic ACh receptors. In 1993, tacrine was first approved by FDA to treat with mild to moderate AD,53,54 in addition three cholinesterase inhibitors were followed: donepezil (1996),55,56 rivastigmine (2000)57 and galantamine (2001).58 However, other drugs have not been approved yet, including AChE inhibitors velnacrine, physostigmine, eptastigmine, metrifonate etc.,59 muscarinic receptor agonists cevimeline (AF102B), milameline, sabcomeline (SB 202026), talsaclidine, xanomeline and alvameline (LU 25-109).60 In addition to the cholinesterase inhibitor, memantine, a N-methyl D-aspartate (NMDA) receptor antagonist which acts on the glutamatergic system, is another FDA approved for treatment of moderate-to-severe AD drugs.61,62 Besides the drugs approved by FDA, there has still been progress in development of cholinergic drugs in clinical trials as well as patented lead compounds. Fig. 1 and Table S1† show five drug approved by the US FDA for treatment in AD.
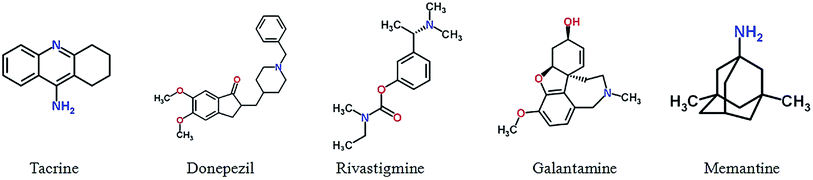 |
| Fig. 1 Five medicines approved by the US FDA for treatment in Alzheimer's disease. | |
Huperzine A, an alkaloid derived from the Chinese herb Huperzia serrata, acts as a selective inhibitor of acetylcholinesterase, which has a mechanism of action similar to donepezil.63 Huperzine A has shown promising effects on the treatment of Alzheimer's disease,64 including improvement of cognitive function, daily living activity, and global clinical assessment.65 However, one trial demonstrated no significant change in cognitive function as measured by Alzheimer's disease Assessment Scale-Cognitive Subscale (ADAS-Cog)66 and clinical data are limited by poor methodological quality.67 Pro-drug of huperzine A, named ZT-1, derived from natural product, is a potent and selective AChE inhibitor. The results from the Phase I clinical trials showed that ZT-1 has an admirable pharmacokinetic with a rapid absorption and a wide distribution in human.68
Physostigmine, originally having been extracted from calabar beans, is an AChE inhibitor, but it has limited treatment effects and serious side effects.69 The (−)-phenserine, a derivative of physostigmine, is an AChE inhibitor that has an effect on cognitive improvement. It also can reduce the translation of APP to reduce Aβ concentrations, suggesting (−)-phenserine may be a promising multitarget drug of AD.70
Memogain (Gln-1062), an inactive pro-drug of galantamine, liberates galantamine on cleavage by a carboxylesterase in the brain. Memogain has more than 15-fold higher bioavailability in the brain than the same doses of galantamine due to the more hydrophobic characteristics. Memogain may represent a valuable drug with higher potency in enhancing cognition for AD treatment, a significantly lower plaque density in the brain, and much lesser gastrointestinal side effects.71,72
Ladostigil is a novel multitarget drug combined with acetylcholine–butyrylcholinesterase cholinesterase inhibitor and brain selective monoamine oxidase A and B inhibitor. It can relieve scopolamine-induced impairment in spatial memory, and increase brain cholinergic activity in rat. Furthermore, it was proved to possess anti-apoptotic and neuroprotective including the regulation of APP process, activation of protein kinase C and mitogen-activated protein kinase signaling pathways.73 NGX267 (AF267B), as M1-selective muscarinic agonists, can enhance the cognitive ability.74 In AD transgenic mice, it also reduced Aβ1-42 and Tau hyperphosphorylation in the cortex and hippocampus, presenting an unique beneficial effects on therapy in AD.75 EVP-6124 is a partial, selective agonist of the α-7 nicotinic acetylcholine receptor (α7-nAChR) with highly CNS-penetrant. It can improve cognitive deficits by boosting the ACh response of α7 nAChRs. EVP-6124 moved into Phase III for AD, supporting a new therapeutic strategy for the treatment of cognitive impairment.76 Additionally, GTS-21 is a selective agonist of the α7 nicotinic receptor, showed promising characteristics during Phase II clinical trial.77 Fig. 2 and Table S2† show cholinergic inhibitors in clinical trials.
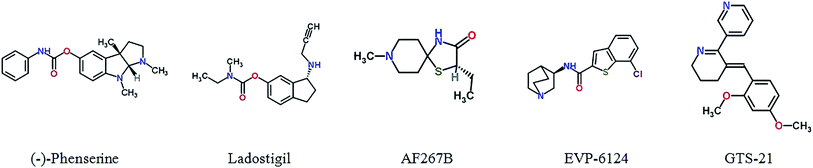 |
| Fig. 2 Cholinergic inhibitors in clinical trials. | |
4. Amyloid-targeted therapies
4.1. Decreasing Aβ production
4.1.1. β-Secretase inhibitors. LY2811376 is the first orally non-peptidic small-molecule BACE1 inhibitor with satisfactory pharmacokinetic and pharmacodynamic properties from preclinical animal models to man.78 It can penetrate the blood brain barrier, and showed long-lasting effect on reducing the level of Aβ in healthy volunteers. However, clinical development was stopped according to a chronic non-target-associated toxicology. LY2886721, next-generation orally available BACE1 inhibitor with agreeable drug properties, reduce the concentrations of cerebral Aβ40, Aβ42 and sAPP-β with safety and good tolerability.79,80 Unfortunately, it was terminated because of abnormal liver biochemical tests. MK-8931 developed by the pharmaceutical company Merck, is a BACE1 inhibitor tested for the treatment of AD in Phase I clinical trial. It can significantly reduce the levels of CSF Aβ in a dose-dependent and sustained way.81 MK-8931 also reduces the concentration of CSF Aβ in patients with mild-to-moderate AD.82 And further research shows that MK-8931 elicits few adverse effects previously ascribed to BACE inhibition, different doses are well tolerated and reduce CNS β-amyloid in both healthy human subjects and AD patients. The human data are suitable for the amyloid pathway model and provide a meaningful guidance for further experiments.83 E2609, an orally available BACE1 inhibitor, showed dose-dependent reductions of Aβ concentrations in CSF and/or plasma in a single oral ascending dose study and multiple oral ascending dose study respectively. Phase 2 clinical trial of E2609 is planned by Eisai.84,85 Fig. 3 and Table S3† show β-secretase inhibitors in clinical trials.
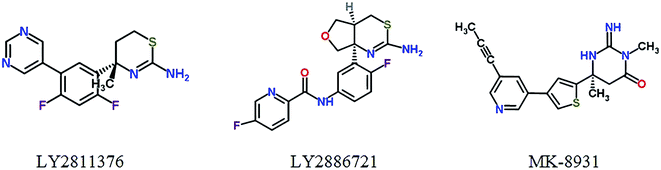 |
| Fig. 3 β-Secretase inhibitors in clinical trials. | |
4.1.2. γ-Secretase inhibitors and modulators. Semagacestat (LY-450139) is a γ-secretase inhibitor in the aim to treat AD. It can lower Aβ concentrations in the plasma and cerebrospinal fluid with a dose-dependent manner.86 However, the trial was terminated owning to severe adverse effects and worsen cognition performance compared to placebo group.87 It is believed that inhibiting γ-secretase may disturb Notch signaling proteins and other cell surface receptors.88 Avagacestat (BMS-708163) is also a γ-secretase inhibitor with Notch-sparing effect. Nevertheless, avagacestat did not demonstrate obvious efficacy from Phase II trials in MIC.89 Begacestat (GSI-953) is a thiophene sulfonamide γ-secretase selectively inhibitor which inhibits cleavage of APP over Notch.90 The compound has shown promise in recent Phase I clinical trials.91NIC5-15 is a natural compound acted as a Notch-sparing γ-secretase inhibitor and an insulin sensitizer. The compound can improve cognitive function through multiple mechanisms including reduce Aβ production by modulating γ-secretase.92 The result shows that NIC5-15 is safe and has good tolerability and further feasibility trials are needed.93 CHF5074, a new microglial modulator, reduces brain Aβ burden to enhance spatial memory cognitive in transgenic mice of AD model.94 CHF5074 shows dose-dependent effects in central nervous system and well tolerated and safety in mild-to-moderate patients.95 E2012 is also a novel γ-secretase modulator which decreases the concentration of Aβ40 and Aβ42 in rat in a dose-dependent manner, and without affecting Notch cleavage.96 Fig. 4 and Table S4† show γ-secretase inhibitors and modulators in clinical trials.
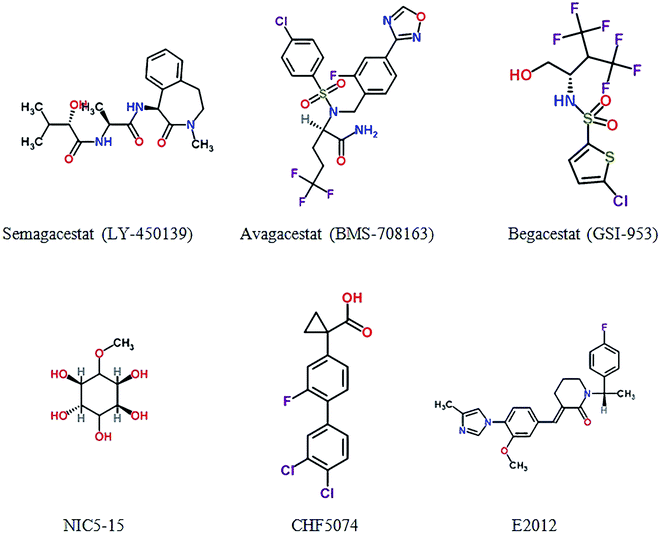 |
| Fig. 4 γ-Secretase inhibitors and modulators in clinical trials. | |
4.2. Promoting Aβ clearance
4.2.1. Active AD immunotherapy. AN-1792 was the first full-length Aβ1-42 active vaccine used in clinical trial. Although the trial was terminated after 6% of the participants developed severe side effect, it provided an important proof of concept.97 CAD106 is the second-generation active immunotherapy vaccine that comprises Aβ1–6 peptide. The study suggests that CAD106 can reduce Aβ accumulation and lead to acceptable antibody response with a safe and tolerance manner in Phase II trials.98 ACI-24 is a Aβ1–15 liposome-based vaccine that can restore the memory defect and reduce plaque in transgenic mice. A phase I/II clinical trial is currently ongoing for investigating the safety and efficacy of mild-to-moderate AD patients.99 UB-311 is synthetic peptides, consisting of UBITh helper T-cell epitopes and coupled to the Aβ1–14 peptide.100 A phase 1 clinical trial of UB-311 has successfully completed, illustrating safety and tolerability.101 In addition, Phase 2 clinical trials are being prepared.Other ongoing trails include ACC-001, V950, Lu AF20513 and AD02 as well. ACC-001 (Vanutide cridificar), an Aβ1–7/Qs21 adjuvant immunotherapeutic vaccine, was evaluated in phase 2a and results came out with safety profile in mild-to-moderate AD patients.102 V950, multivalent Aβ peptide/ISCOMATRIX™ adjuvant, aimed to produce Aβ antibodies to recognize pyroglutamate-modified and other N-terminally truncated Aβ fragments. A phase 1 has been completed and further studies were discontinued.103 Lu AF20513 was developed by Lundbeck A/S (Valby, Denmark) according to Aβ1–12 peptide replaced with two foreign T-helper epitopes from tetanus toxoid. It is currently being tested in preclinical trail.104 Additionally, AD02 is an amyloid-beta (Aβ)-targeting vaccine to elicit anti-Aβ antibodies, and phase II study was finished.105 DNA amyloid-beta protein immunotherapy is currently being investigated in preclinical studies.106 Tau immunotherapy AADvac1 and ACI-35 are also under preclinical.107
4.2.2. Passive AD immunotherapy. AAB-001 (Bapineuzumab) is the first humanized monoclonal antibody targeting the Aβ N-terminus (Aβ1–5). The antibody binds strongly to deposite amyloid plaques to reduce the Aβ plaque burden and induces Fc-mediated microglial phagocytosis of Aβ plaques in mouse.108 Two phase 3 trials involved patients with mild-to-moderate Alzheimer disease were conducted, however, bapineuzumab failed to improve primary clinical outcomes.109 LY-2062430 (Solanezumab) is a humanized monoclonal antibody to the mid-domain of Aβ16–24, which binds to the soluble Aβ.110 The compound has been proved safety in Phase 2 findings, nevertheless, neither clinical trial in phase 3, showing significant cognition improvement nor functional ability in patients with mild-to- moderate AD.111 Two previously phase 3 clinical trial results were renewed in some mild patients, and the secondary outcomes suggested that Solanezumab can slow the cognitive decline of 34% according to the ADAS-Cog and Mini-Mental State Examination (MMSE) (P < 0.05).112 PF-04360365 (Ponezumab) is a humanized IgG2δA monoclonal antibody aiming to reduce immune effector. PF-04360365 showed accepted safety and well tolerated findings without antibody-induced side effects.113 And another clinical trial also showed similar results.114 GSK-933776 is a humanized Fc-attenuated/inactivated anti-Aβ monoclonal antibody. GSK933776 showed pharmacological activity and engaged target in plasma and CSF without causing brain amyloid-related imaging abnormalities-edema (ARIA-E/H) in patients with mild AD or MCI.115,116 MABT5102A was a humanized Aβ1–15 monoclonal antibody with IgG4 isotype. It can inhibit Aβ aggregation and promote its disaggregation without vasogenic edema and cerebral microhemorrhage induced by overactivation of microglial cells.117 Aducanumab (BIIB037) is a human monoclonal antibody that selectively targets misfolded Aβ peptides. Aducanumab restores calcium homeostasis in Tg2576 mice,118 and also reduce soluble and insoluble Aβ in a dose-dependent manner.119 In a recent study, Sevigny et al. reports beneficial effects on the amyloid pathology and the cognitive status in patients with prodromal or mild AD.120 The phase 1b clinical test had revitalizes the “amyloid cascade hypothesis” and bring mononuclear phagocytes to the center stage of AD treatment.
4.3. Preventing Aβ aggregation
Tramiprosate (3-amino-1-propanesulfonic acid, 3APS) is an orally-administered amyloid antagonist, which binds to soluble Aβ peptide and designs to reduce Aβ aggregation and prevent fibril formation.121 Tramiprosate produces cytoprotective effects against Aβ-induced neurotoxicity, and exerts significant reduction of soluble and insoluble Aβ in the brain of transgenic mice.122 Clinical trials show that tramiprosate can slow hippocampal atrophy,123 and have some benefit on cognition.124 However, the further Phase III trial has been terminated due to its unsuccessful in demonstrating efficacy.125,126 Scyllo-inositol, an endogenous inositol stereoisomer, is another anti-aggregation compound, exerting specific health-promoting effects for Alzheimer disease.127 It stabilized a small conformer of Aβ42 in vitro, and neutralized cell-derived Aβ oligomers in vivo. Moreover, scyllo-inositol can decrease neuronal toxicity and abate cognitive deficits in multiple mouse models of AD.128 A Phase II clinical trial demonstrated acceptable safety, however, primary clinical efficacy outcomes were not significant.129 Epigallo-catechin-3-gallate (EGCG),a natural flavanol derived from green tea which shows multiple neuroprotetive activities,130 bind to unfolded peptide to prevent the formation of Aβ toxic oligomers.131 It can also modulate cell signalling and reverse superoxide dismutase activity and the damage effects of AlCl3 neurotoxicity, which improves mitochondrial and cholinergic synaptic functions.132,133 PBT1 is a metal chelator that promotes the solubilisation by disturbing the chelation between Aβ and metal ions in vitro or mouse model studies. However, Phase II clinical suggested that there was no significant positive clinical benefit for patients with AD. Unfortunately, phase III trial was abandoned.134
The second-generation metal–protein attenuating compound PBT2, was developed as a metal chaperone which affected the metal-induced Aβ oligomerisation.135 It has greater blood–brain barrier permeability. PBT2 can obstruct Aβ oligomerization, decrease soluble and insoluble Aβ and promote the clearance of Aβ oligomer.136 Phase II study showed that PBT2 can reduce the concentrations of Aβ42 in CSF and improved cognitive function with safety and tolerance.137 TTP488 (PF-04494700) is a small-molecule oral antagonist of the receptor for advanced glycation end products. The low dose (5 mg) shows good safety profile but associated with conclusive results in Phase II trial with mild to moderate AD.138 Another clinical trial demonstrates that low-dose (5 mg) could be a benefit dose in further Phase 3 trials in patients with mild AD.139
A recent study provided a new framework for the rational identification of a series of drug candidates for neurodegenerative diseases, chemical kinetics approach was applied to study the effect of small molecules on the deposition rate of Aβ42 which was quantitatively analyzed. An anticancer drug, bexarotene, was reported to suppress Aβ42 deposition by targeting the primary nucleation step in the aggregation of Aβ42 and delaying the formation of toxic species in neuroblastoma cells.140 Fig. 5 and Table S5† show drugs in clinical trials to prevent Aβ aggregation.
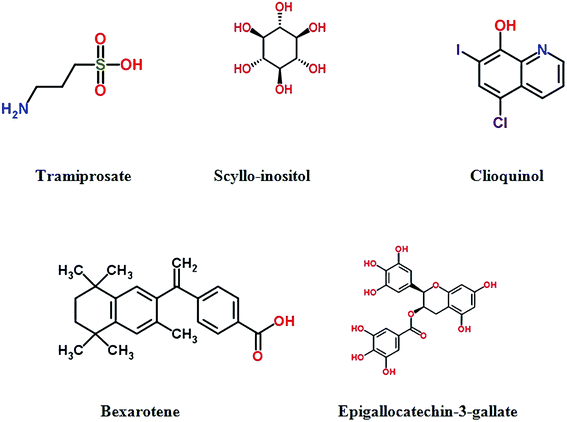 |
| Fig. 5 Drugs in clinical trials to prevent Aβ aggregation. | |
5. Other potential therapeutic strategies in AD
5.1. Drugs to target Tau protein
Leuco-methylthioninium (LMTX), a selective Tau aggregation inhibitor by preventing the formation and spread of NFTs and reducing the Tau pathology in transgenic mouse models, is the reduced form of methylthioninium (MT), which is the first substance that can degrade Tau protein.141 An exploratory phase 2 study in mild or moderate Alzheimer's disease was conducted, safety and effectiveness was determined as well.142 The main results of a broad participation of 115 academic centres in 15 months of randomised, controlled double-blind, parallel-group clinical trial is negative, the results do not suggest the benefit of LMTX as an additional treatment of mild to moderate Alzheimer's disease.143
Glycogen synthase kinase 3 β (GSK3β) is a serine/threonine kinase which plays an important role in regulating Tau protein phosphorylation and involves in processing of amyloid-beta peptides.144,145 Tideglusib (NP-031112) is a small non-ATP competitive GSK-3 inhibitor, for the treatment of Alzheimer's disease in clinical trials. It can lower Tau protein hyperphosphorylation, reduce brain amyloid plaque levels, improve learning and memory and prevent the loss of neurons in some animal models.146 Studies demonstrated valuable safety in clinical trials in AD patients.147,148
5.2. Neurotrophins
Neurotrophins are dimeric peptide hormones. Nerve growth factor (NGF), the first neurotrophin, regulates many aspects of neuronal development and function, plays an important role in the survival and differentiation of neurons.149 Recently, the study shows that BDNF exerts substantial protective effects on crucial neuronal circuitry involved in Alzheimer's disease, revealed the correlation between the decreased NGF and AD.150 Thus, neurotrophins have been acted as an attractive target for treatment of AD. AAV2-NGF (CERE-110) is designed to deliver NGF by gene to cross the blood–brain barrier, which increases production of acetylcholine and enhances the function basal forebrain cholinergic neurons.151,152 A Phase I study has been completed and a multi-center, placebo-controlled Phase II clinical trial in the observation phase.153 T-817MA [1-{3-[2-(1-benzothiophen-5-yl)ethoxy]propyl}azetidin-3-olmaleate] has both neuroprotective and neurotrophic effects and also has the ability to improve the cognitive impairment in transgenic mice,154 The Phase II trial has been completed for its evaluations on safety and tolerability.155
5.3. Targeting mitochondrial dysfunction
There is a growing body of evidence supporting that mitochondrial dysfunction has a significant influence on the process of AD.156 Mitochondrial dysfunction also has connection with oxidative stress and Tau pathology.157,158 Due to the correlation between mitochondrial alterations and AD, strategies targeting decreases the related oxidative stress and removal of damaged mitochondria possess great promise in AD treatment. Latrepirdine (Dimebon®), a small molecule compound, is used for the treatment of AD. Dimebon can modify hippocampal APP/Aβ pathology,159 and ameliorate mitochondrial membrane potential and ATP production,160 indicated the potential treatment for neurodegenerative diseases.161
5.4. PPAR-γ agonists
Pioglitazone is an insulin sensitizer of the thiazolidinedione class of peroxisome-proliferator activated receptor γ (PPAR-γ) agonists. Takeda developed pioglitazone as a once-daily treatment of type 2 diabetes. The PPAR-γ agonist improves cognition in AD mice, and mixed results in prior human trials.162 In August 2013, Takeda and Zinfandel Pharmaceuticals began ‘Tomorrow’, a Phase III trial that is to enroll 5800 cognitively-normal participants and run for 4 years. The study has two separate goals; one is to evaluate how accurately a diagnostic algorithm based on the genes ApoE and TOMM40, developed by Zinfandel, predicts a person's risk of developing mild cognitive impairment due to AD within 5 years. The other is to evaluate pioglitazone's ability to delay this diagnosis.
5.5. Computer simulation
Studies showed that molecular dynamics simulation can be used to design Aβ aggregation inhibitor and a structure-based drug discovery procedure has been explored to identify the binding pockets between small-molecule and Aβ peptide.163,164 In a study, a total 11 compounds were identified which reduce Aβ cytotoxicity by shifting the equilibrium of Aβ from oligomers to fibers by comprehensive performing these methods.165 NQ-Trp (1,4-naphthoquinon-2-yl-L-tryptophan), a small molecular which has been reported to inhibit aggregation of Aβ.166 NQ-trp was found to be the best binders of five small-molecule drugs with Aβ17-42 by using a hierarchical computational procedure,167 further more, an extensive atomistic replica exchange molecular dynamics simulations was used to explain the beneficial effect of NQTrp in reducing both the level of Aβ1-42 aggregation and toxicity.168 Aiming to investigate the molecular mechanism of NQ-Trp combined experimental and simulation studies were performed. The converging results explained its low inhibitory efficiency which due to the lack of specific “binding site”-type between NQ-Trp and Aβ, and suggested that another mechanism was involved in anti-AD activity of NQ-Trp-type molecules models in vivo.169,170 Yang Z. et al. found that graphite can inhibit Aβ peptide monomer fibrils and can clear the mature amyloid fibers through penetration and extraction of peptides. Experimental evidence such as molecular dynamics simulations, atomic force microscopy images, thioflavin fluorescence assays, and cell viability and ROS assays confirmed the prediction results of computer simulation.171 The molecular mechanism and molecular dynamics of (−)-epigallocatechin gallate (EGCG)172 and CQ1-3 (ref. 173) inhibiting Aβ aggregation were studied respectively by computer simulation. Computer simulation provides new insights into the underlying molecular mechanisms that define drug–amyloid interaction and suggests the directions of further AD drug development.174 Fig. 6 and Table S6† show other potential therapeutic strategies in AD.
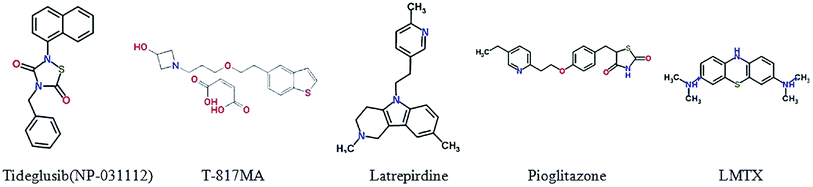 |
| Fig. 6 Other potential therapeutic strategies in AD. | |
6. Concluding remarks and future perspective
So far, the development of AD drugs has achieved some success in the improvement of symptoms, whereas it also has several failed aspects of disease modification. While many clinical and drug design studies are undergoing, we must recognize that it is quite difficult to successfully cure AD with single therapy, which is attributed to the complex pathophysiology of AD. It is believed not to be caused by single gene defects, but rather by a large number of genes, proteins and their complex interactions that ultimately lead to the change of this disease.175 Multi-target drug discovery may be a more promising treatment strategy for AD.176 It could overcome the deficiency of the poor development effects of one-target-one-compound. Several multi target compounds already have been designed, such as dual binding AChE and BACE1 inhibitors,177 AChE inhibitors and antioxidants,178 which provide better therapeutic effects on both symptomatic and disease modifying in AD. At this point, multiple-pharmacology natural products can be used as prototypes for the drugs design of AD treatment.179 Herbal formulae such as Kai-Xin-San (consisting of Ginseng Radix, Poria, Polygalae Radix, and Acori Tatarinowii Rhizoma) has been used in the treatment of Parkinson's disease and Alzheimer's disease,180,181 also provide new insight for the treatment of AD. New methods, such as quantitative systems pharmacology,182 chemogenomics knowledgebase,183 metabolomics184–189 and chinmedomics strategy190–195 will meet the challenge and provide a promising avenue for the discovery and clinical development of new-generation drugs for AD.
Competing financial interests
The authors declare no competing financial interests.
Acknowledgements
This work was supported by grants from the Key Program of Natural Science Foundation of State (Grant No. 81302905, 81373930, 81430093, 81673586), National Key Subject of Drug Innovation (Grant No. 2015ZX09101043-005, 2015ZX09101043-011), TCM State Administration Subject of Public Welfare of (Grant No. 2015468004), Natural Science Foundation of Heilongjiang Province of China (H2015038), University Nursing Program for Young Scholars with Creative Talents in Heilongjiang Province (UNPYSCT-2015118).
References
- H. W. Querfurth and F. M. Laferla, N. Engl. J. Med., 2010, 362(4), 329–344 CrossRef CAS PubMed
. - A. Wimo, B. Winblad and L. Jonsson, Alzheimer's & Dementia, 2010, 6(2), 98–103 Search PubMed
. - M. P. Mattson, Nature, 2004, 430(7000), 631–639 CrossRef CAS PubMed
. - K. G. Mawuenyega, Science, 2010, 330, 1774 CrossRef CAS PubMed
. - D. P. Hanger, B. H. Anderton and W. Noble, Trends Mol. Med., 2009, 15(3), 112–119 CrossRef CAS PubMed
. - B. Su, X. Wang, A. Nunomura, P. I. Moreira, H. G. Lee, G. Perry, M. A. Smith and X. Zhu, Curr. Alzheimer Res., 2008, 5(6), 525–532 CrossRef CAS PubMed
. - L. A. Craig, N. S. Hong and R. J. McDonald, Neurosci. Biobehav. Rev., 2011, 35(6), 1397–1409 CrossRef CAS PubMed
. - C. Holmes, Neuropathol. Appl. Neurobiol., 2013, 39(1), 51–68 CrossRef CAS PubMed
. - M. A. Greenough, J. Camakaris and A. I. Bush, Neurochem. Int., 2013, 62(5), 540–555 CrossRef CAS PubMed
. - J. Hardy, Curr. Alzheimer Res., 2006, 3, 71–73 CrossRef CAS PubMed
. - C. Haass and D. J. Selkoe, Nat. Rev. Mol. Cell Biol., 2007, 8, 101–112 CrossRef CAS PubMed
. - C. Nerelius, M. Fitzen and J. Johansson, Biochem. Biophys. Res. Commun., 2010, 396, 2–6 CrossRef CAS PubMed
. - R. E. Tanzi and L. Bertram, Cell, 2005, 120, 545–555 CrossRef CAS PubMed
. - D. M. Walsh and D. J. Selkoe, J. Neurochem., 2007, 101, 1172–1184 CrossRef CAS PubMed
. - R. Gallardo, M. Ramakers, F. De Smet, F. Claes, L. Khodaparast, J. R. Couceiro, T. Langenberg, M. Siemons, S. Nyström, L. J. Young, R. F. Laine, L. Young, E. Radaelli, I. Benilova, M. Kumar, A. Staes, M. Desager, M. Beerens, P. Vandervoort, A. Luttun, K. Gevaert, G. Bormans, M. Dewerchin, J. Van Eldere, P. Carmeliet, G. Vande Velde, C. Verfaillie, C. F. Kaminski, B. De Strooper, P. Hammarström, K. P. Nilsson, L. Serpell, J. Schymkowitz and F. Rousseau, Science, 2016, 354(6313), aah4949 CrossRef PubMed
. - D. J. Selkoe and J. Hardy, EMBO Mol. Med., 2016, 8(6), 595–608 CrossRef CAS PubMed
. - S. Konzack, E. Thies, A. Marx, E. M. Mandelkow and E. Mandelkow, J. Neurosci., 2007, 27, 9916–9927 CrossRef CAS PubMed
. - L. Buée, T. Bussière, V. Buée-Scherrer, A. Delacourte and P. R. Hof, Brain Res. Rev., 2000, 33, 95–130 CrossRef
. - J. P. Brion, C. Smith, A. M. Couck, J. M. Gallo and B. H. Anderton, J. Neurochem., 1993, 61, 2071–2080 CrossRef CAS PubMed
. - D. P. Hanger, B. H. Anderton and W. Noble, Trends Mol. Med., 2009, 15(3), 112–119 CrossRef CAS PubMed
. - E. D. Roberson, Science, 2007, 316, 750–754 CrossRef CAS PubMed
. - M. Jin, N. Shepardson, T. Yang, G. Chen, D. Walsh and D. J. Selkoe, Proc. Natl. Acad. Sci. U. S. A., 2011, 108(14), 5819–5824 CrossRef CAS PubMed
. - M. Valko, D. Leibfritz, J. Moncol, M. T. Cronin, M. Mazur and J. Telser, Int. J. Biochem. Cell Biol., 2007, 39(1), 44–84 CrossRef CAS PubMed
. - M. Mohsenzadegan and A. Mirshafiey, Iran. J. Allergy, Asthma Immunol., 2012, 11(3), 203–216 CAS
. - A. Nunomura, R. J. Castellani, X. Zhu, P. I. Moreira, G. Perry and M. A. Smith, J. Neuropathol. Exp. Neurol., 2006, 65, 631–641 CrossRef CAS PubMed
. - C. Pocernich and D. Butterfield, Biochim. Biophys. Acta, 2012, 1822(5), 625–630 CrossRef CAS PubMed
. - R. T. Bartus, R. L. Dean 3rd, B. Beer and A. S. Lippa, Science, 1982, 217(4558), 408–414 CAS
. - R. Schliebs and T. Arendt, Behav. Brain Res., 2011, 221, 555–563 CrossRef CAS PubMed
. - D. S. Auld, T. J. Kornecook, S. Bastianetto and R. Quirion, Prog. Neurobiol., 2003, 3, 209–245 Search PubMed
. - R. Schliebs and T. Arendt, J. Neural Transm., 2006, 113, 1625–1644 CrossRef CAS PubMed
. - J. Berger-Sweeney, Neurosci. Biobehav. Rev., 2003, 27, 401–411 CrossRef CAS PubMed
. - H. Akiyama, S. Barger, S. Barnum, B. Bradt, J. Bauer, G. M. Cole, N. R. Cooper, P. Eikelenboom, M. Emmerling, B. L. Fiebich, C. E. Finch, S. Frautschy, W. S. Griffin, H. Hampel, M. Hull, G. Landreth, L. Lue, R. Mrak, I. R. Mackenzie, P. L. McGeer, M. K. O'Banion, J. Pachter, G. Pasinetti, C. Plata-Salaman, J. Rogers, R. Rydel, Y. Shen, W. Streit, R. Strohmeyer, I. Tooyoma, F. L. Van Muiswinkel, R. Veerhuis, D. Walker, S. Webster, B. Wegrzyniak, G. Wenk and T. Wyss-Coray, Neurobiol. Aging, 2000, 21, 383–421 CrossRef CAS PubMed
. - W. S. Griffin and R. E. Mrak, J. Leukocyte Biol., 2002, 72(2), 233–238 CAS
. - C. E. Finch and T. E. Morgan, Curr. Alzheimer Res., 2007, 4, 185–189 CrossRef CAS PubMed
. - T. Town, V. Nikolic and J. Tan, J. Neuroinflammation, 2005, 2, 24 CrossRef PubMed
. - E. E. Tuppo and H. R. Arias, Cell Biol., 2005, 37, 289–305 CAS
. - G. Halliday, S. R. Robinson, C. Shepherd and J. Kril, Clin. Exp. Pharmacol. Physiol., 2000, 27, 1–8 CrossRef CAS PubMed
. - M. Farina, D. S. Avila, J. B. da Rocha and M. Aschner, Neurochem. Int., 2013, 62(5), 575–594 CrossRef CAS PubMed
. - S. Bolognin, L. Messori and P. Zatta, NeuroMol. Med., 2009, 11(4), 223–238 CrossRef CAS PubMed
. - M. G. Savelieff, S. Lee, Y. Liu and M. H. Lim, ACS Chem. Biol., 2013, 8(5), 856–865 CrossRef CAS PubMed
. - B. F. Popescu and H. Nichol, CNS Neurosci. Ther., 2011, 17, 256–268 CrossRef CAS PubMed
. - W. Zheng and A. D. Monnot, Pharmacol. Ther., 2012, 133(2), 177–188 CrossRef CAS PubMed
. - K. Jomova, D. Vondrakova, M. Lawson and M. Valko, Mol. Cell. Biochem., 2010, 345(1–2), 91–104 CrossRef CAS PubMed
. - B. B. Muhoberac and R. Vidal, Front. Aging Neurosci., 2013, 5, 32 Search PubMed
. - M. Kawahara, J. Alzheimer's Dis., 2005, 8(2), 171–182 CAS
, discussion 209–115. - J. R. Walton, J. Alzheimer's Dis., 2013, 35(1), 7–43 CAS
. - A. Campbell, J. Alzheimer's Dis., 2006, 10(2–3), 165–172 Search PubMed
. - E. House, M. Esiri, G. Forster, P. G. Ince and C. Exley, Metallomics, 2012, 4(1), 56–65 RSC
. - D. A. Drachman and J. Leavitt, Arch. Neurol., 1974, 30, 113–121 CrossRef CAS PubMed
. - T. Babic, J. Neurol., Neurosurg. Psychiatry, 1999, 67(4), 558 CrossRef CAS
. - R. Schliebs and T. Arendt, J. Neural Transm., 2006, 113, 1625–1644 CrossRef CAS PubMed
. - D. S. Auld, T. J. Kornecook, S. Bastianetto and R. Quirion, Prog. Neurobiol., 2003, 3, 209–245 Search PubMed
. - A. S. Relman, N. Engl. J. Med., 1991, 324, 349–352, DOI:10.1056/nejm199101313240525.
- M. J. Knapp, D. S. Knopman, P. R. Solomon, W. W. Pendlebury, C. S. Davis and S. I. Gracon, J. Am. Med. Assoc., 1994, 271, 985–991 CrossRef CAS PubMed
. - J. S. Birks, D. Melzer and H. Beppu, Cochrane Database of Systematic Reviews, 2000, 4, CD001190 Search PubMed
. - J. Birks and R. J. Harvey, Cochrane Database of Systematic Reviews, 2006, 25(1), CD001190 Search PubMed
. - J. Birks, J. Grimley Evans, V. Iakovidou and M. Tsolaki, Cochrane Database of Systematic Reviews, 2009, CD001191 Search PubMed
. - C. Loy and L. Schneider, Cochrane Database of Systematic Reviews, 2006, 1, CD001747 Search PubMed
. - R. E. Becker, N. H. Greig and E. Giacobini, J. Alzheimer's Dis., 2008, 15, 303–325 Search PubMed
. - R. A. McArthur, J. Gray and R. Schreiber, Curr. Opin. Invest. Drugs, 2010, 11, 740–760 CAS
. - B. Reisberg, R. Doody, A. Stöffler, F. Schmitt, S. Ferris, H. J. Möbius and Memantine Study Group, N. Engl. J. Med., 2003, 348(14), 1333–1341 CrossRef CAS PubMed
. - D. Olivares, V. K. Deshpande, Y. Shi, D. K. Lahiri, N. H. Greig, J. T. Rogers and X. Huang, Curr. Alzheimer Res., 2012, 9(6), 746–758 CrossRef CAS PubMed
. - L. M. Fu and J. T. Li, J. Evidence-Based Complementary Altern. Med., 2011, 2011, 640284 Search PubMed
. - S. H. Xing, C. X. Zhu, R. Zhang and L. An, J. Evidence-Based Complementary Altern. Med., 2014, 2014, 363985 Search PubMed
. - G. Yang, Y. Wang, J. Tian and J. P. Liu, PLoS One, 2013, 8(9), e74916 CAS
. - M. S. Rafii, S. Walsh, J. T. Little, K. Behan, B. Reynolds, C. Ward, S. Jin, R. Thomas and P. S. Aisen, Neurology, 2011, 76(16), 1389–1394 CrossRef CAS PubMed
. - J. Yue, B. R. Dong, X. Lin, M. Yang, H. M. Wu and T. Wu, Cochrane Database of Systematic Reviews, 2012, 12, CD008827 Search PubMed
. - J. Y. Jia, Q. H. Zhao, Y. Liu, Y. Z. Gui, G. Y. Liu, D. Y. Zhu, C. Yu and Z. Hong, Acta Pharmacol. Sin., 2013, 34(7), 976–982 CrossRef CAS PubMed
. - F. Coelho and J. Birks, Cochrane Database of Systematic Reviews, 2001, 2, CD001499 Search PubMed
. - B. Winblad, E. Giacobini, L. Frolich, L. T. Friedhoff, G. Bruinsma, R. E. Becker and N. H. Greig, J. Alzheimer's Dis., 2010, 22(4), 1201–1208 CAS
. - S. Bhattacharya, A. Maelicke and D. Montag, J. Alzheimer's Dis., 2015, 46(1), 123–136 CrossRef CAS PubMed
. - A. Maelicke, A. Hoeffle-Maas, J. Ludwig, A. Maus, M. Samochocki, U. Jordis and A. K. Koepke, J. Mol. Neurosci., 2010, 40(1–2), 135–137 CrossRef CAS PubMed
. - O. Weinreb, T. Amit, O. Bar-Am and M. B. Youdim, Curr. Drug Targets, 2012, 13(4), 483–494 CrossRef CAS PubMed
. - A. Fisher, Neurodegener. Dis., 2008, 5(3–4), 237–240 CrossRef CAS PubMed
. - A. Fisher, R. Brandeis, R. H. Bar-Ner, M. Kliger-Spatz, N. Natan, H. Sonego, I. Marcovitch and Z. Pittel, J. Mol. Neurosci., 2002, 19(1–2), 145–153 CrossRef CAS PubMed
. - J. Prickaerts, N. P. van Goethem, R. Chesworth, G. Shapiro, F. G. Boess, C. Methfessel, O. A. Reneerkens, D. G. Flood, D. Hilt, M. Gawryl, S. Bertrand, D. Bertrand and G. König, Neuropharmacology, 2012, 62(2), 1099–1110 CrossRef CAS PubMed
. - P. Zawieja, J. M. Kornprobst and P. Metais, Geriatrics & Gerontology International, 2012, 12(3), 365–371 Search PubMed
. - P. C. May, R. A. Dean, S. L. Lowe, F. Martenyi, S. M. Sheehan, L. N. Boggs, S. A. Monk, B. M. Mathes, D. J. Mergott, B. M. Watson, S. L. Stout, D. E. Timm, E. Smith Labell, C. R. Gonzales, M. Nakano, S. Jhee, S. M. Yen, Y. L. Ereshefsk, T. D. Lindstrom, D. O. Calligaro, P. J. Cocke, D. Greg Hall, S. Friedrich, M. Citron and J. E. Audia, J. Neurosci., 2011, 31(46), 16507–16516 CrossRef CAS PubMed
. - F. Martenyi, R. A. Dean, S. Lowe, M. Nakano, S. Monk, B. A. Willis, C. Gonzales, D. Mergott, D. Leslie, P. May, A. James, H. Gevorkyan, S. Jhee, L. Ereshefsky and M. Citron, Alzheimer's & Dementia, 2012, 8(4), P583–P584 Search PubMed
. - B. Willis, F. Martenyi, R. Dean, S. Lowe, M. Nakano, S. Monk, C. Gonzales, D. Mergott, L. Daugherty, M. Citron and P. May, Alzheimer's & Dementia, 2012, 8, P582 Search PubMed
. - M. Forman, J. Palcza, J. Tseng, J. Leempoels, S. Ramael, D. Han, S. Jhee, L. Ereshefsky, M. Tanen, O. Laterza, M. Dockendorf, G. Krishna, L. Ma, J. Wagner and M. Troyer, Alzheimer's & Dementia, 2012, 8(4), 704–705 Search PubMed
. - M. Forman, H. J. Kleijn, M. Dockendorf, J. Palcza, J. Tseng, C. Canales, M. Egan, M. Kennedy, O. Laterza, L. Ma, J. Scott, M. Tanen, J. Apter, M. Backonja, L. Ereshefsky, H. Gevorkyan, S. Jhee, R. Rynders, A. Zari, E. Bryan, J. Wagner, M. Troyer and J. Stone, Alzheimer's & Dementia, 2013, 9, 139 Search PubMed
. - M. E. Kennedy, A. W. Stamford, X. Chen, K. Cox, J. N. Cumming, M. F. Dockendorf, M. Egan, L. Ereshefsky, R. A. Hodgson, L. A. Hyde, S. Jhee, H. J. Kleijn, R. Kuvelkar, W. Li, B. A. Mattson, H. Mei, J. Palcza, J. D. Scott, M. Tanen, M. D. Troyer, J. L. Tseng, J. A. Stone, E. M. Parker and M. S. Forman, Sci. Transl. Med., 2016, 8(363), 363ra150 CrossRef PubMed
. - R. Lai, B. Albala, J. M. Kaplow, J. Aluri, M. Yen and A. Satlin, Alzheimer's & Dementia, 2012, 8, 96 Search PubMed
. - F. Bernier, Y. Sato, M. Matijevic, H. Desmond, S. M. Grath, L. Burns, J. M. Kaplow and B. Albala, Alzheimer's & Dementia, 2013, 9, 886 Search PubMed
. - D. B. Henley, P. C. May, R. A. Dean and E. R. Siemers, Expert Opin. Pharmacother., 2009, 10(10), 1657–1664 CrossRef CAS PubMed
. - K. Blennow, H. Zetterberg, C. Haass and T. Finucane, Nat. Med., 2013, 19(10), 1214–1215 CrossRef PubMed
. - R. S. Doody, R. Raman, M. Farlow, T. Iwatsubo, B. Vellas, S. Joffe, K. Kieburtz, F. He, X. Sun, R. G. Thomas, P. S. Aisen, E. Siemers, G. Sethuraman and R. Mohs, N. Engl. J. Med., 2013, 369(4), 341–350 CrossRef CAS PubMed
. - V. Coric, S. Salloway, C. H. van Dyck, B. Dubois, N. Andreasen, M. Brody, C. Curtis, H. Soininen, S. Thein, T. Shiovitz, G. Pilcher, K. Ferrarek, J. Seibyl, J. M. Cedarbaum, C. Albright, H. H. Feldman and R. M. Berman, JAMA Neurology, 2015, 72(11), 1324–1333 CrossRef PubMed
. - R. L. Martone, H. Zhou, K. Atchison, T. Comery, J. Z. Xu, X. Huang, X. Gong, M. Jin, A. Kreft, B. Harrison, S. C. Mayer, S. Aschmies, C. Gonzales, M. M. Zaleska, D. R. Riddell, E. Wagner, P. Lu, S. C. Sun, J. Sonnenberg-Reines, A. Oganesian, K. Adkins, M. W. Leach, D. W. Clarke, D. Huryn, M. Abou-Gharbia, R. Magolda, J. Bard, G. Frick, S. Raje, S. B. Forlow, C. Balliet, M. E. Burczynski, P. H. Reinhart, H. I. Wan, M. N. Pangalos and J. S. Jacobsen, J. Pharmacol. Exp. Ther., 2009, 331(2), 598–608 CrossRef CAS PubMed
. - C. R. Hopkins, ACS Chem. Neurosci., 2012, 3(1), 3–4 CrossRef CAS PubMed
. - G. Pasinetti, Z. Rosen and H. Grossman, Alzheimer's & Dementia, 2009, 5(4), e28 Search PubMed
. - H. Grossman, G. Marzloff, X. Luo, D. Leroith, M. Sano and G. Pasinetti, Alzheimer's & Dementia, 2009, 5(4), P259 Search PubMed
. - B. P. Imbimbo, B. Hutter-Paier, G. Villetti, F. Facchinetti, V. Cenacchi, R. Volta, A. Lanzillotta, M. Pizzi and M. Windisch, Br. J. Pharmacol., 2009, 156(6), 982–993 CrossRef CAS PubMed
. - J. Ross, S. Sharma, J. Winston, M. Nunez, G. Bottini, M. Franceschi, E. Scarpini, E. Frigerio, F. Fiorentini, M. Fernandez, S. Sivilia, L. Giardino, L. Calza, D. Norris, H. Cicirello, D. Casula and B. P. Imbimbo, Curr. Alzheimer Res., 2013, 10(7), 742–753 CrossRef CAS PubMed
. - T. Hashimoto, A. Ishibashi, H. Hagiwara, Y. Murata, O. Takenaka and T. Miyagawa, Alzheimer's & Dementia, 2010, 6(4), S242 Search PubMed
. - C. Holmes, D. Boche, D. Wilkinson, G. Yadegarfar, V. Hopkins, A. Bayer, R. W. Jones, R. Bullock, S. Love, J. W. Neal, E. Zotova and J. A. Nicoll, Lancet, 2008, 372, 216–223 CrossRef CAS
. - B. Winblad, N. Andreasen, L. Minthon, A. Floesser, G. Imbert, T. Dumortier, R. P. Maguire, K. Blennow, J. Lundmark, M. Staufenbiel, J. M. Orgogozo and A. Graf, Lancet Neurol., 2012, 11(7), 597–604 CrossRef CAS PubMed
. - A. Muhs, D. T. Hickman, M. Pihlgren, N. Chuard, V. Giriens, C. Meerschman, I. van der Auwera, F. van Leuven, M. Sugawara, M. C. Weingertner, B. Bechinger, R. Greferath, N. Kolonko, L. Nagel-Steger, D. Riesner, R. O. Brady, A. Pfeifer and C. Nicolau, Proc. Natl. Acad. Sci. U. S. A., 2007, 104(23), 9810–9815 CrossRef CAS PubMed
. - C. Y. Wang, C. L. Finstad, A. M. Walfield, C. Sia, K. K. Sokoll, T. Y. Chang, X. D. Fang, C. H. Hung, B. Hutter-Paier and M. Windisch, Vaccine, 2007, 25, 3041–3052 CrossRef CAS PubMed
. - D. Galimberti, L. Ghezzi and E. Scarpini, J. Neurol. Sci., 2013, 333(1–2), 50–54 CrossRef CAS PubMed
. - H. Arai, H. Suzuki and T. Yoshiyama, Curr. Alzheimer Res., 2015, 12(3), 242–254 CrossRef CAS PubMed
. - J. M. Savage, G. Wu, A. McCampbell, R. K. Wessner, M. Citron, X. Liang, S. Hsieh, A. L. Wolfe, G. G. Kinney, B. L. Rosen and J. J. Renger, Alzheimer's & Dementia, 2010, 6, S142 Search PubMed
. - H. Davtyan, A. Ghochikyan, I. Petrushina, A. Hovakimyan, A. Davtyan, A. Poghosyan, A. M. Marleau, N. Movsesyan, A. Kiyatkin, S. Rasool, A. K. Larsen, P. J. Madsen, K. M. Wegener, D. K. Ditlevsen, D. H. Cribbs, L. O. Pedersen and M. G. Agadjanyan, J. Neurosci., 2013, 33(11), 4923–4934 CrossRef CAS PubMed
. - S. Hendrix, N. Ellison, S. Stanworth, L. Tierney, F. Mattner, W. Schmidt, B. Dubois and A. Schneeberger, Journal of Prevention of Alzheimer's Disease, 2015, 2(2), 91–102 CAS
. - D. Lambracht-Washington, B. X. Qu, M. Fu, L. D. Anderson Jr, O. Stuve, T. N. Eagar and R. N. Rosenberg, Cell. Mol. Neurobiol., 2011, 31, 867–874 CrossRef CAS PubMed
. - B. Winblad, A. Graf, M. E. Riviere, N. Andreasen and J. M. Ryan, Alzheimer's Res. Ther., 2014, 6(1), 7 CrossRef PubMed
. - F. Bard, R. Barbour, C. Cannon, R. Carretto, M. Fox, D. Games, T. Guido, K. Hoenow, K. Hu, K. Johnson-Wood, K. Khan, D. Kholodenko, C. Lee, M. Lee, R. Motter, M. Nguyen, A. Reed, D. Schenk, P. Tang, N. Vasquez, P. Seubert and T. Yednock, Proc. Natl. Acad. Sci. U. S. A., 2003, 100(4), 2023–2028 CrossRef CAS PubMed
. - S. Salloway, R. Sperling, N. C. Fox, K. Blennow, W. Klunk, M. Raskind, M. Sabbagh, L. S. Honig, A. P. Porsteinsson, S. Ferris, M. Reichert, N. Ketter, B. Nejadnik, V. Guenzler, M. Miloslavsky, D. Wang, Y. Lu, J. Lull, I. C. Tudor, E. Liu, M. Grundman, E. Yuen, R. Black, H. R. Brashear and Bapineuzumab 301 and 302 Clinical Trial Investigators, N. Engl. J. Med., 2014, 370, 322–333 CrossRef CAS PubMed
. - H. Samadi and D. Sultzer, Expert Opin. Biol. Ther., 2011, 11(6), 787–798 CrossRef CAS PubMed
. - R. S. Doody, R. G. Thomas, M. Farlow, T. Iwatsubo, B. Vellas, S. Joffe, K. Kieburtz, R. Raman, X. Sun, P. S. Aisen, E. Siemers, H. Liu-Seifert, R. Mohs, Alzheimer's Disease Cooperative Study Steering Committee and Solanezumab Study Group, N. Engl. J. Med., 2014, 370(4), 311–321 CrossRef CAS PubMed
. - E. R. Siemers, K. L. Sundell, C. Carlson, M. Case, G. Sethuraman, H. Liu-Seifert, S. A. Dowsett, M. J. Pontecorvo, R. A. Dean and R. Demattos, Alzheimer's & Dementia, 2016, 12(2), 110–120 Search PubMed
. - G. B. Freeman, J. C. Lin, J. Pons and N. M. Raha, J. Alzheimer's Dis., 2012, 28(3), 531–541 CAS
. - I. Miyoshi, Y. Fujimoto, M. Yamada, S. Abe, Q. Zhao, C. Cronenberger, K. Togo, T. Ishibashi, M. M. Bednar, J. W. Kupiec and B. Binneman, Int. J. Clin. Pharmacol. Ther., 2013, 51(12), 911–923 CrossRef CAS PubMed
. - T. Leyhe, N. Andreasen, M. Simeoni, A. Reich, C. A. von Arnim, X. Tong, A. Yeo, S. Khan, A. Loercher, M. Chalker, C. Hottenstein, H. Zetterberg, J. Hilpert and P. Mistry, Alzheimer's Res. Ther., 2014, 6(2), 19 CrossRef PubMed
. - N. Andreasen, M. Simeoni, H. Ostlund, P. I. Lisjo, T. Fladby, A. E. Loercher, G. J. Byrne, F. Murray, P. T. Scott-Stevens, A. Wallin, Y. Y. Zhang, L. H. Bronge, H. Zetterberg, A. K. Nordberg, A. J. Yeo, S. A. Khan, J. Hilpert and P. C. Mistry, PLoS One, 2015, 10(3), e0098153 Search PubMed
. - O. Adolfsson, M. Pihlgren, N. Toni, Y. Varisco, A. L. Buccarello, K. Antoniello, S. Lohmann, K. Piorkowska, V. Gafner, J. K. Atwal, J. Maloney, M. Chen, A. Gogineni, R. M. Weimer, D. L. Mortensen, M. Friesenhahn, C. Ho, R. Paul, A. Pfeifer, A. Muhs and R. J. Watts, J. Neurosci., 2012, 32(28), 9677–9689 CrossRef CAS PubMed
. - K. V. Kastanenka, T. Bussiere, N. Shakerdge, F. Qian, P. H. Weinreb, K. Rhodes and B. J. Bacskai, J. Neurosci., 2016, pii, 2080–2116 Search PubMed
. - J. Ferrero, L. Williams, H. Stella, K. Leitermann, A. Mikulskis, J. O'Gorman and J. Sevigny, Alzheimer's & Dementia, Translational Research & Clinical Interventions, 2016, 2(3), 169–176 Search PubMed
. - J. Sevigny, P. Chiao, T. Bussière, P. H. Weinreb, L. Williams, M. Maier, R. Dunstan, S. Salloway, T. Chen, Y. Ling, J. O'Gorman, F. Qian, M. Arastu, M. Li, S. Chollate, M. S. Brennan, O. Quintero-Monzon, R. H. Scannevin, H. M. Arnold, T. Engber, K. Rhodes, J. Ferrero, Y. Hang, A. Mikulskis, J. Grimm, C. Hock, R. M. Nitsch and A. Sandrock, Nature, 2016, 537(7618), 50–56 CrossRef CAS PubMed
. - P. S. Aisen, S. Gauthier, B. Vellas, R. Briand, D. Saumier, J. Laurin and D. Garceau, Curr. Alzheimer Res., 2007, 4(4), 473–478 CrossRef CAS PubMed
. - F. Gervais, J. Paquette, C. Morissette, P. Krzywkowski, M. Yu, M. Azzi, D. Lacombe, X. Kong, A. Aman, J. Laurin, W. A. Szarek and P. Tremblay, Neurobiol. Aging, 2007, 28(4), 537–547 CrossRef CAS PubMed
. - S. Gauthier, P. S. Aisen, S. H. Ferris, D. Saumier, A. Duong, D. Haine, D. Garceau, J. Suhy, J. Oh, W. Lau and J. Sampalis, J. Nutr., Health Aging, 2009, 13(6), 550–557 CrossRef CAS
. - D. Saumier, A. Duong, D. Haine, D. Garceau and J. Sampalis, J. Nutr., Health Aging, 2009, 13(9), 808–812 CrossRef CAS
. - P. S. Aisen, S. Gauthier, S. H. Ferris, D. Saumier, D. Haine, D. Garceau, A. Duong, J. Suhy, J. Oh, W. C. Lau and J. Sampalis, Arch. Med. Sci., 2011, 7(1), 102–111 CrossRef CAS PubMed
. - N. Herrmann, S. A. Chau, I. Kircanski and K. L. Lanctôt, Drugs, 2011, 71(15), 2031–2065 CrossRef CAS PubMed
. - K. Tanaka, S. Takanaka and K. Yoshida, Bioengineered, 2014, 5(5), 331–334 CrossRef PubMed
. - K. Ma, L. A. Thomason and J. Mclaurin, Adv. Pharmacol., 2012, 64, 177–212 CAS
. - S. Salloway, R. Sperling, R. Keren, A. P. Porsteinsson, C. H. van Dyck, P. N. Tariot, S. Gilman, D. Arnold, S. Abushakra, C. Hernandez, G. Crans, E. Liang, G. Quinn, M. Bairu, A. Pastrak, J. M. Cedarbaum and ELND005-AD201 Investigators, Neurology, 2011, 77(13), 1253–1262 CrossRef CAS PubMed
. - L. Xicota, J. Rodríguez-Morató, M. Dierssen and R. de la Torre, Curr. Drug Targets, 2015 Search PubMed
, Epub ahead of print. - S. A. Mandel, T. Amit, L. Kalfon, L. Reznichenko, O. Weinreb and M. B. Youdim, J. Alzheimer's Dis., 2008, 15(2), 211–222 CAS
. - T. Jayasena, A. Poljak, G. Smythe, N. Braidy, G. Münch and P. Sachdev, Ageing Res. Rev., 2013, 12(4), 867–883 CrossRef CAS PubMed
. - A. Jelenković, M. D. Jovanović, I. Stevanović, N. Petronijević, D. Bokonjić, J. Zivković and R. Igić, Phytother. Res., 2014, 28(1), 82–87 CrossRef PubMed
. - E. L. Sampson, L. Jenagaratnam and R. McShane, Cochrane Database of Systematic Reviews, 2012, 5, CD005380 Search PubMed
. - P. A. Adlard, A. Sedjahtera, L. Gunawan, L. Bray, D. Hare, J. Lear, P. Doble, A. I. Bush, D. I. Finkelstein and R. A. Cherny, Aging Cell, 2013, 13(2), 351–359 CrossRef PubMed
. - P. A. Adlard, R. A. Cherny, D. I. Finkelstein, E. Gautier, E. Robb, M. Cortes, I. Volitakis, X. Liu, J. P. Smith, K. Perez, K. Laughton, Q. X. Li, S. A. Charman, J. A. Nicolazzo, S. Wilkins, K. Deleva, T. Lynch, G. Kok, C. W. Ritchie, R. E. Tanzi, R. Cappai, C. L. Masters, K. J. Barnham and A. I. Bush, Neuron, 2008, 59(1), 43–55 CrossRef CAS PubMed
. - L. Lannfelt, K. Blennow, H. Zetterberg, S. Batsman, D. Ames, J. Harrison, C. L. Masters, S. Targum, A. I. Bush, R. Murdoch, J. Wilson, C. W. Ritchie and PBT2-201-EURO study group, Lancet Neurol., 2008, 7(9), 779–786 CrossRef CAS PubMed
. - D. Galasko, J. Bell, J. Y. Mancuso, J. W. Kupiec, M. N. Sabbagh, C. van Dyck, R. G. Thomas and P. S. Aisen, Neurology, 2014, 82, 1536–1542 CrossRef CAS PubMed
. - A. H. Burstein, I. Grimes, D. R. Galasko, P. S. Aisen and M. Sabbagh, BMC Neurol., 2014, 14, 12 CrossRef PubMed
. - J. Habchi, P. Arosio, M. Perni, A. R. Costa, M. Yagi-Utsumi, P. Joshi, S. Chia, S. I. Cohen, M. B. Müller, S. Linse, E. A. Nollen, C. M. Dobson, T. P. Knowles and M. Vendruscolo, Sci. Adv., 2016, 2(2), e1501244 Search PubMed
. - D. Seripa, V. Solfrizzi, B. P. Imbimbo, A. Daniele, A. Santamato, M. Lozupone, G. Zuliani, A. Greco, G. Logroscino and F. Panza, Expert Rev. Neurother., 2016, 16(3), 259–277 CrossRef CAS PubMed
. - C. M. Wischik, R. T. Staff, D. J. Wischik, P. Bentham, A. D. Murray, J. M. Storey, K. A. Kook and C. R. Harrington, J. Alzheimer's Dis., 2015, 44(2), 705–720 CAS
. - S. Gauthier, H. H. Feldman, L. S. Schneider, G. K. Wilcock, G. B. Frisoni, J. H. Hardlund, H. J. Moebius, P. Bentham, K. A. Kook, D. J. Wischik, B. O. Schelter, C. S. Davis, R. T. Staff, L. Bracoud, K. Shamsi, J. M. Storey, C. R. Harrington and C. M. Wischik, Lancet, 2016, pii, S0140 Search PubMed
. - C. J. Phiel, C. A. Wilson, V. M. Lee and P. S. Klein, Nature, 2003, 423(6938), 435–439 CrossRef CAS PubMed
. - E. Rockenstein, M. Torrance, A. Adame, M. Mante, P. Bar-on, J. B. Rose, L. Crews and E. Masliah, J. Neurosci., 2007, 27(8), 1981–1991 CrossRef CAS PubMed
. - J. M. Domínguez, A. Fuertes, L. Orozco, M. del Monte-Millán, E. Delgado and M. Medina, J. Biol. Chem., 2012, 287(2), 893–904 CrossRef PubMed
. - T. del Ser, K. C. Steinwachs, H. J. Gertz, M. V. Andrés, B. Gómez-Carrillo, M. Medina, J. A. Vericat, P. Redondo, D. Fleet and T. León, J. Alzheimer's Dis., 2013, 33(1), 205–215 CAS
. - S. Lovestone, M. Boada, B. Dubois, M. Hüll, J. O. Rinne, H. J. Huppertz, M. Calero, M. V. Andrés, B. Gómez-Carrillo, T. León, T. del Ser and ARGO investigators, J. Alzheimer's Dis., 2015, 45(1), 75–88 CAS
. - L. F. Reichardt, Philos. Trans. R. Soc. London, Ser. B, 2006, 361(1473), 1545–1564 CrossRef CAS PubMed
. - A. H. Nagahara, D. A. Merrill, G. Coppola, S. Tsukada, B. E. Schroeder, G. M. Shaked, L. Wang, A. Blesch, A. Kim, J. M. Conner, E. Rockenstein, M. V. Chao, E. H. Koo, D. Geschwind, E. Masliah, A. A. Chiba and M. H. Tuszynski, Nat. Med., 2009, 15(3), 331–337 CrossRef CAS PubMed
. - R. J. Mandel, Curr. Opin. Mol. Ther., 2010, 12(2), 240–247 CAS
. - F. Hefti, J. Neurosci., 1986, 6, 2155–2162 CAS
. - M. S. Rafii, T. L. Baumann, R. A. Bakay, J. M. Ostrove, J. Siffert, A. S. Fleisher, C. D. Herzog, D. Barba, M. Pay, D. P. Salmon, Y. Chu, J. H. Kordower, K. Bishop, D. Keator, S. Potkin and R. T. Bartus, Alzheimer's & Dementia, 2014, 10, 571–581 Search PubMed
. - T. Fukushima, A. Nakamura, N. Iwakami, Y. Nakada, H. Hattori, S. Hoki, H. Yamaguchi, M. Nakagawa, N. Terashima and H. Narita, Biochem. Biophys. Res. Commun., 2011, 407(4), 730–734 CrossRef CAS PubMed
. - H. Liu, L. Wang, W. Su and X. Q. Xie, Pharm. Pat. Anal., 2014, 3(4), 429–447 CrossRef CAS PubMed
. - V. García-Escudero, P. Martín-Maestro, G. Perry and J. Avila, Oxid. Med. Cell. Longevity, 2013, 2013, 162152 Search PubMed
. - X. Wang, W. Wang, L. Li, G. Perry, H. G. Lee and X. Zhu, Biochim. Biophys. Acta, 2013, 1842(8), 1240–1247 CrossRef PubMed
. - R. A. Quintanilla, T. A. Matthews-Roberson, P. J. Dolan and G. V. Johnson, J. Biol. Chem., 2009, 284(28), 18754–18766 CrossRef CAS PubMed
. - S. E. Perez, M. Nadeem, K. R. Sadleir, J. Matras, C. M. Kelley, S. E. Counts, R. Vassar and E. J. Mufson, Int. J. Physiol., Pathophysiol. Pharmacol., 2012, 4(3), 115–127 CAS
. - S. H. Eckert, J. Eckmann, K. Renner, G. P. Eckert, K. Leuner and W. E. Muller, J. Alzheimer's Dis., 2012, 31(1), 21–32 CAS
. - M. N. Sabbagh and H. A. Shill, Curr. Opin. Invest. Drugs, 2010, 11(1), 80–91 CAS
. - B. W. Miller, K. C. Willett and A. R. Desilets, Ann. Pharmacother., 2011, 45, 1416–1424 CrossRef CAS PubMed
. - P. A. Novick, D. H. Lopes, K. M. Branson, A. Esteras-Chopo, I. A. Graef, G. Bitan and V. S. Pande, J. Med. Chem., 2012, 55(7), 3002–3010 CrossRef CAS PubMed
. - M. Zhu, A. De Simone, D. Schenk, G. Toth, C. M. Dobson and M. Vendruscolo, J. Chem. Phys., 2013, 139(3), 035101 CrossRef PubMed
. - L. Jiang, C. Liu, D. Leibly, M. Landau, M. Zhao, M. P. Hughes and D. S. Eisenberg, eLife, 2013, 2, e00857 Search PubMed
. - R. Scherzer-Attali, R. Pellarin, M. Convertino, A. Frydman-Marom, N. Egoz-Matia, S. Peled, M. Levy-Sakin, D. E. Shalev, A. Caflisch, E. Gazit and D. Segal, PLoS One, 2010, 5(6), e11101 Search PubMed
. - Y. Chebaro, P. Jiang, T. Zang, Y. Mu, P. H. Nguyen, N. Mousseau and P. Derreumaux, J. Phys. Chem. B, 2012, 116(29), 8412–8422 CrossRef CAS PubMed
. - T. Zhang, W. Xu, Y. Mu and P. Derreumaux, ACS Chem. Neurosci., 2014, 5(2), 148–159 CrossRef CAS PubMed
. - P. Nguyen and P. Derreumaux, Acc. Chem. Res., 2014, 47(2), 603–611 CrossRef CAS PubMed
. - O. Berthoumieu, P. H. Nguyen, M. P. Castillo-Frias, S. Ferre, B. Tarus, J. Nasica-Labouze, S. Noël, O. Saurel, C. Rampon, A. J. Doig, P. Derreumaux and P. Faller, Chemistry, 2015, 21(36), 12657–12666 CrossRef CAS PubMed
. - Z. Yang, C. Ge, J. Liu, Y. Chong, Z. Gu, C. A. Jimenez-Cruz, Z. Chai and R. Zhou, Nanoscale, 2015, 7(44), 18725–18737 RSC
. - T. Zhang, J. Zhang, P. Derreumaux and Y. Mu, J. Phys. Chem. B, 2013, 117(15), 3993–4002 CrossRef CAS PubMed
. - M. Dong, H. Li, D. Hu, W. Zhao, X. Zhu and H. Ai, ACS Chem. Neurosci., 2016, 7(5), 599–614 CrossRef CAS PubMed
. - A. J. Doig and P. Derreumaux, Curr. Opin. Struct. Biol., 2015, 30, 50–56 CrossRef CAS PubMed
. - M. Ray, J. Ruan and W. Zhang, Genome Biol., 2008, 9(10), R148 CrossRef PubMed
. - R. Leon, A. G. Garcia and J. Marco-Contelles, Med. Res. Rev., 2013, 33(1), 139–189 CrossRef CAS PubMed
. - Y. Zhu, K. Xiao, L. Ma, B. Xiong, Y. Fu, H. Yu, W. Wang, X. Wang, D. Hu, H. Peng, J. Li, Q. Gong, Q. Chai, X. Tang, H. Zhang, J. Li and J. Shen, Bioorg. Med. Chem., 2009, 17(4), 1600–1613 CrossRef CAS PubMed
. - M. Rosini, V. Andrisano, M. Bartolini, M. L. Bolognesi, P. Hrelia, A. Minarini, A. Tarozzi and C. Melchiorre, J. Med. Chem., 2005, 48(2), 360–363 CrossRef CAS PubMed
. - H. Liu, F. Liang, W. Su, N. Wang, M. Lv, P. Li, Z. Pei, Y. Zhang, Q. Xie, L. Wang and Y. Wang, J. Ethnopharmacol., 2013, 147(2), 366–372 CrossRef CAS PubMed
. - H. Chu, A. H. Zhang, Y. Han, S. Lu, L. Kong, J. Han, Z. D Liu, H. Sun and X. J. Wang, J. Chromatogr. B: Anal. Technol. Biomed. Life Sci., 2016, 1015–1016, 50–61 CrossRef CAS PubMed
. - A. Zhang, H. Sun and X. Wang, Mass Spectrom. Rev., 2016, 9999, 1–14 Search PubMed
. - L. Wang, C. Ma, P. Wipf, H. Liu, W. Su and X. Q. Xie, AAPS J., 2013, 15(2), 395–406 CrossRef CAS PubMed
. - H. Liu, L. Wang, M. Lv, R. Pei, P. Li, Z. Pei, Y. Wang, W. Su and X. Q. Xie, J. Cheminf., 2014, 54(4), 1050–1060 CrossRef CAS PubMed
. - H. Chu, A. Zhang, Y. Han and X. Wang, World J. Tradit. Chin. Med., 2015, 1, 26–32 CrossRef
. - A. Zhang, H. Sun, S. Qiu and X. Wang, J. Evidence-Based Complementary Altern. Med., 2013, 2013, 257909 Search PubMed
. - X. Wang, A. Zhang, H. Sun, Y. Han and G. Yan, TrAC, Trends Anal. Chem., 2016, 76, 86–94 CrossRef CAS
. - A. Zhang, H. Sun and X. Wang, Phytother. Res., 2014, 28(4), 526–533 CrossRef PubMed
. - X. Wang, A. Zhang, G. Yan, Y. Han and H. Sun, TrAC, Trends Anal. Chem., 2014, 63, 180–187 CrossRef CAS
. - H. Chu, H. Sun, G. Yan, A. Zhang, C. Liu, H. Dong, X. Meng and X. Wang, World Journal of Traditional Chinese Medicine, 2015, 1(3), 1–12 CrossRef
. - Q. Liu, A. Zhang, L. Wang, G. Yan, H. Zhao, H. Sun, S. Zou, J. Han, C. W. Ma, L. Kong, X. Zhou, Y. Nan and X. Wang, Sci. Rep., 2016, 6, 38437 CrossRef CAS PubMed
. - X. Wang, A. Zhang, X. Zhou, Q. Liu, Y. Nan, Y. Guan, L. Kong, Y. Han, H. Sun and G. Yan, Sci. Rep., 2016, 6, 18997 CrossRef CAS PubMed
. - X. H. Zhou, A. H. Zhang, L. Wang, Y. L. Tan, Y. Guan, Y. Han, H. Sun and X. J. Wang, Chin. J. Nat. Med., 2016, 14(8), 561–581 Search PubMed
. - A. H. Zhang, H. Sun, G. L. Yan, P. Wang, Y. Han and X. J. Wang, China J. Chin. Mater. Med., 2015, 40(4), 569–576 Search PubMed
. - X. J. Wang, A. H. Zhang, H. Sun and G. L. Yan, Chin. Herb. Med., 2016, 8(4), 299–307 CrossRef
. - A. Zhang, Q. Liu, H. Zhao, X. Zhou, H. Sun, Y. Nan, S. Zou, C. W. Ma and X. Wang, Sci. Rep., 2016, 6, 19333 CrossRef CAS PubMed
.
Footnote |
† Electronic supplementary information (ESI) available. See DOI: 10.1039/c6ra26737h |
|
This journal is © The Royal Society of Chemistry 2017 |