DOI:
10.1039/C6RA25805K
(Paper)
RSC Adv., 2017,
7, 11394-11402
Use of fermented glutinous rice as a natural enzyme cocktail for improving dough quality and bread staling
Received
25th October 2016
, Accepted 30th January 2017
First published on 14th February 2017
Abstract
Solid-state fermentation of glutinous rice by Rhizopus oryzae (rice wine or Jiu-niang in Chinese) is a traditional Chinese food as well as a natural enzyme cocktail with the potential to be used in bread making. The objective of this work was for the first time to study the enzyme activities of Jiu-niang and its effect on dough quality and bread staling. The results showed that the principal enzymes in Jiu-niang were amylase, protease and lipase. The Jiu-niang enzyme cocktail significantly improved the volume, texture, and shelf life of wheat breads. The use of an optimal enzyme concentration (addition of Jiu-niang at 10% on flour basis) increased specific volume (19%), reduced crumb hardness (50%), and reduced moisture migration rate and bread staling rate. Using low-field nuclear magnetic resonance (LF-NMR) analysis, proton mobility and starch retrogradation of Jiu-niang bread during storage were determined and discussed. These results indicated that Jiu-niang could be used as a natural quality improver for bread making.
1. Introduction
Bread staling is a very complex physico-chemical phenomenon which has been studied for years and still needs to be resolved. During the storage time, bread gradually becomes harder, loses its aroma and moisture. The mechanisms of staling have been excessively investigated by many researchers.1–3 Many of them considered that starch retrogradation is only part of the reason for the changes in bread properties during storage. Water plays an important role in the staling of bread, both at a macroscopic level, migrating from bread crumb to crust but also at a molecular level, influencing the redistribution of protons and affecting the molecular dynamics of water.4,5 When retrogradation of amylopectin occurs, water changes the original location and is incorporated in crystals of retrograded amylopectin or migrates from gluten to starch causing the loss of plasticity of bread crumb and finally reduces molecular mobility.6–8 Water is redistributed among bread domains, leading to crumb hardening during storage.9 Nowadays, some additives such as enzymes and emulsifiers are applied in bread baking to improve the quality of bread. Previous studies have reported that application of amylase in bread making has a significant effect on bread anti-staling and improves the elasticity of bread crumb.10,11 Moreover, bread supplemented with amylase decreased the firmness of bread, increased fragrant aroma owing to Maillard reactions, improved bread loaf volume and retarded starch retrogradation.3,11,12 The anti-staling mechanisms or modes of action of amylases were different. The efficiency of anti-staling of endo-acting amylases can be attributed to their effects on weakening the starch networks by hydrolyzing the internal bonds of starch polymers.3 For exo-acting amylases, the mechanism of action is due to the degradation of the outer amylopectin branches so that amylopectin recrystallisation can be controlled. Addition of amylase in bread production is quite popular since the enzyme activity is regularly very low in wheat flour.13
Sweet fermented glutinous rice (Jiu-niang) is a traditional Chinese food prepared by fermentation of steamed glutinous rice with the starter which is composed of moulds.14,15 Jiu-niang has sweet flavor and rich nutrition which is widely accepted and is recognized as one of the most famous traditional solid-state fermentation foods in China. The principal strain in the starter is Rhizopus oryzae (determined by strain identification). Rhizopus oryzae has the ability to produce different enzymes in large quantities such as amylase, protease and lipase. The strain secretes amylase to degrade the starch molecule into fermentable sugars and non fermentable sugars. Sugars that microorganism cannot use are remained in the system to form the typical sweet aroma. Meanwhile, proteins are hydrolyzed into amino acids by protease which contributes to nutrients of Jiu-niang. Furthermore, Jiu-niang is widely known by Chinese people for promoting digestion, relieving fatigue, stimulating blood circulation and reducing fat accumulation in blood vessels. Traditional Chinese medicinal herbs are often soaked in Jiu-niang in order to improve their efficacy. Jiu-niang is rich in amino acids, oligosaccharides and microelements which will enhance nutrition values and sweet flavor of bakery products.16
Steamed bread is a kind of steamed wheat-based food which represents 40% of the wheat consumption in China.17 Jiu-niang has been used as a quality improver in steamed bread making mainly for textural and sensory properties amelioration. Dough treated by Jiu-niang generates special flavor substances and offers sweet sensations of steamed bread. However, to the best of our knowledge, the application of Jiu-niang in bakery products has not been reported. The enzyme cocktail produced by Rhizopus oryzae is served as natural additive in dough fermentation which is beneficial to health. Jiu-niang is an extremely rich system including various kinds of enzymes which may improve bread quality and retard the staling during the storage. In this study, the effect of enzymes of Jiu-niang on bread quality will be investigated. Changes of carbohydrates and proteins during dough fermentation, as well as moisture migration, bread texture and proton distribution of bread with and without Jiu-niang supplemented during storage will be discussed.
2. Materials and methods
2.1 Materials
Wheat flour was obtained from Dachengliangyou Co., LTD (Shanghai, China). The wheat flour (given by the company) contains: 18% proteins, 2% lipids, 24% moisture and 17% energy. The starter was purchased from cereals, oil and food Co., LTD (Suzhou, China). The starter was a kind of Chinese traditional starter which was composed of Rhizopus oryzae.
2.2 Preparation of Jiu-niang
Jiu-niang preparation was started by washing and soaking of 1000 g glutinous rice in water for 10 h. Then, the glutinous rice was steamed in the electric steamer (SUPOR Z12YN6-G2, SUPOR Electrical Equipments, Zhejiang, China) for 30 min. The fermentation starter was subsequently added with glutinous rice
:
starter ratio of 99
:
1 (w/w) after the glutinous rice cooled down to 30 to 35 °C. The glutinous rice was stored in a container and fermented at 30 °C, 90% relative humidity for 36 h. The fermented glutinous rice was filtrated to obtain the filtrate.
2.3 Preparation of dough and bread
The basic dough recipe was composed of 500 g of wheat flour, 220 g of water for the control sample, 40 g of egg, 90 g of sugar, 5 g of salt and 6 g of yeast. For the Jiu-niang dough preparation, the addition of Jiu-niang varied from 5% to 20% on flour basis. Sugar, salt and egg were firstly added and mixed 2 min using a mixer (B25, HENGLIAN Mechanical Equipments, Guangdong, China), and followed by the addition of the rest of ingredients and mixed for 30 min. The formed dough was then divided into pieces (60 g), molded and rounded before the fermentation was carried out at 32 °C for 2 h with a relative humidity of 82%. Baking was conducted in an automatic thermostatic oven (YXD-60, XINNANG Electric Equipments, Guangzhou, China) with the condition as follows: top temperature of 190 °C, bottom temperature of 200 °C, 12 min. Prior to further analyses, the breads were cooled down to room temperature and then packaged in hermetic plastic bags and stored in a temperature controlled incubator (LHS-150SC, YIHENG Scientific Instruments, Shanghai, China) at 25 °C for 7 days.
2.4 Enzyme assays
Amylase activity in Jiu-niang was determined according to the method of Tribst & Cristianini (2012) with slight modifications.18 For the determination of α-amylase activity, 1 mL Jiu-niang and 1 mL soluble starch solution (1%) was mixed and incubated at 40 °C for 10 min. The reaction was stopped by adding 2 mL 3,5-dinitrosalicylic acid solution and heating in boiling water for 10 min. The mixture was diluted with distilled water to 25 mL. One unit of enzyme activity was defined as the amount of enzyme catalyzing to the release of 1 mg maltose per minute at pH 5.6 at 40 °C.
Protease activity of Jiu-niang was conducted by the hydrolysis of 1% casein at 40 °C for 10 min with the Folin method.19 1 mL Jiu-niang was incubated with 1 mL substrate solution for 10 min. 2 mL trichloroacetic acid was added in order to stop the reaction. The mixture was centrifuged for 8 min at 5000 rpm. After the centrifugation, 5 mL NaCO3 (0.5 M) and 1 mL Folin–Ciocalteu reagent were added into 1 mL supernatant. The enzyme activity was defined as the amount of enzyme catalyzing the release of 1 μg tyrosine at 40 °C, pH 3.0, for 1 min.
Lipase activity was measured by the method of olive oil hydrolysis.20 The amount of fatty acids was determinate by titration with 0.05 M NaOH. The enzyme activity was defined as the amount of enzyme catalyzing the release of 1 μmol fatty acids at 40 °C.
2.5 Changes of carbohydrates and proteins
Agilent 1100 high performance liquid chromatography (HPLC) equipped with the COSMOSIL Sugar-D Packed Column (Tosoh Corporation, Tokyo, Japan) was used to detect the changes of carbohydrates during the fermentation of dough. 1 g of dough was mixed with 20 mL distilled water and the mixture was agitated on a magnetic stirrer for 25 min at room temperature and centrifuged at 10
000 rpm for 10 min. The supernatant was eluted using 75% acetonitrile aqueous solution at a flow rate of 0.7 mL min−1 and then monitored by an evaporative light-scattering detector.
The protein changes of doughs were analyzed by sodium dodecyl sulfate polyacrylamide gel electrophoresis (SDS-PAGE), using 4% stacking gel and 12% separating gel.21 The gels were run in a Miniprotein 3 unit (Bio-Rad Laboratories, Hercules, CA, USA) at 60 V for 30 min, and followed by 120 V for 90 min. Gels were then scanned by Image Scanner III (GE Healthcare Biosciences, Uppsala, Sweden).
2.6 Physical properties of bread
Specific volume of bread was determined by rapeseed displacement method.22 Crust color was measured at the surface of bread by a chroma meter (CR-400, Konica Minolta, Osaka, Japan). Reducing sugar content of bread was measured using 3,5-dinitrosalicylic acid. 2.5 g of bread was weighed and 150 mL distilled water was added. The mixture was incubated at 50 °C for 20 min. The filtrate was then collected and diluted with water to 250 mL. The solution of reducing sugar was reacted with 3,5-dinitrosalicylic acid in boiling water for 5 min. The absorbance of mixture was then measured at 540 nm by a spectrophotometer (Alpha-1101m, PUYUAN Instruments, Shanghai, China).
2.7 Moisture migration rate
2 g of bread crumb (crust) was weighed and placed in the oven (BAO-80A, SRIK Instruments, Shanghai, China) for drying at 101 °C until constant weight. Moisture migration rate was defined as the moisture of crumb (crust) compared to the interval days between two measurements.
2.8 Crumb texture analysis
Texture profiles were measured by an Analyzer TA.XT Plus (Stable Micro Systems, Surrey, UK) with a cylindrical probe of 50 mm diameter (P50).23 The bread crumb cube was cut into a cube with 2 cm × 2 cm × 2 cm. The rate of the probe was set at 1 mm s−1 and the sample bread was then compressed to 50% of the original height.
2.9 Low-field nuclear magnetic resonance (LF-NMR)
A low-field nuclear magnetic resonance (21.3 MHz) spectrometer (NMI20, NIUMAG corporation, Shanghai, China) was used for determination of the proton distribution in the bread crumb. A magnetic field strength of 0.5 T was applied. T2 relaxation times were detected with the Carr–Purcell–Meiboom–Gill (CPMG) pulse sequence. The 90° pulse length was set as 7.50 μs whereas the 180° pulse length was set as 14.80 μs. Time between 90° and 180° pulse was 100 μs. Measurements were performed under a recycle delay of 2 s and 5000 data points. For the CPMG sequence, 32 scans were used to improve the signal-to-noise ratio. MultiExp Inv Analysis software (provided by NIUMAG corporation) was used to transform the LF-NMR data to different distributions of relaxation times. For each test, the bread crumb (2 g) was placed in the NMR tube (25 mm inner diameter) and compressed using a glass plunger. The bread crumb was measured with LF-NMR during the storage time.
2.10 Statistical analysis
Statistical analysis was carried out with SPSS version 21.0. A one-way-analysis of variance (ANOVA) and Duncan's multiple comparison tests were used to determine the significant differences at a level of 0.05. The main and interactive effects of Jiu-niang concentration and storage time on hardness, springiness and cohesiveness were evaluated using a mixed-model ANOVA, where the measured variables were set as dependent variables, Jiu-niang concentration, storage time and Jiu-niang concentration × storage time as fixed effects, and replicate as random effect.
3. Results and discussion
3.1 Changes of carbohydrates and proteins during dough fermentation
Fig. 1 contains the changes of protein profiles during the fermentation process by using SDS-PAGE. Wheat gluten is a main element which will determine water absorption capacity and viscoelasticity of dough. It is composed of gliadins (50–60%) and glutenins (40–50%). The protein bands obtained ranged from molecular weight of 30 kDa to 80 kDa were probably corresponded to gliadins.24 Glutenins confer strength and elasticity of dough and possess a higher molecular weight (>100 kDa). For the control dough, the bands have no obvious changes before and after fermentation. In case of the fermented Jiu-niang dough, the intensity of some large-sized proteins bands were reduced (>130 kDa) and a new protein band was observed (∼120 kDa). Degradation of large-sized proteins during dough fermentation might be due to the action of protease. Therefore, the addition of Jiu-niang should not be excessive, otherwise the network of wheat gluten will be weakened.
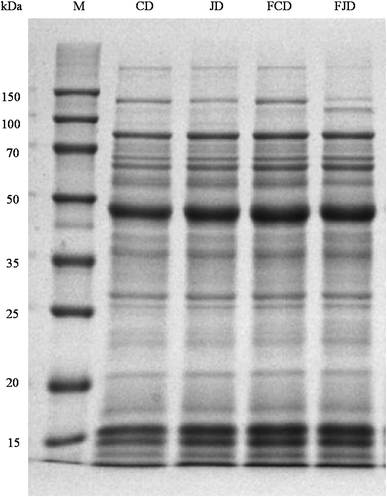 |
| Fig. 1 SDS-PAGE electropherogram of the control and Jiu-niang doughs before and after fermentation. Lane 1: molecular weight maker (M), lane 2: control dough (CD), lane 3: Jiu-niang dough (JD), lane 4: fermented control dough (FCD), lane 5: fermented Jiu-niang dough (FJD). | |
HPLC was used for the analysis of carbohydrates changes of dough during fermentation. One principal peak with the retention time of 10.0 min (data not shown) was observed for the dough without Jiu-niang added (Fig. 2a). For the Jiu-niang dough, there was another major peak appearing with the retention time of 7.8 min (Fig. 2c). According to the curves of the standards, the sugars peaking at 7.8 min and 10.0 min were referred to as monosaccharides and disaccharides, respectively. Disaccharides content of the control dough sharply reduced after fermentation probably due to the consumption by yeasts. Compared with the control, Jiu-niang dough showed a peak containing a large number of monosaccharides which was from the addition of Jiu-niang. However, the dough treated with Jiu-niang showed a decrease of monosaccharides and an increase of disaccharides. Utilization of monosaccharides in preference by yeasts resulted in the decrease of monosaccharides. On the contrary, peak area of disaccharides for the Jiu-niang dough largely increased, from 13
896.1 to 42
171.5 (data not shown). The results showed that the increase of disaccharides was probably attributed to the hydrolysis of polysaccharides by the action of amylase in Jiu-niang. Monosaccharides and disaccharides released could be further used for the growth of yeasts, leading to the expansion of dough and the capacity to hold gas and the improvement of the quality of bakery products.
 |
| Fig. 2 HPLC chromatogram of monosaccharides and oligosaccharides of (a) control dough (CD), (b) fermented control dough (FCD), (c) Jiu-niang dough (JD) and (d) fermented Jiu-niang dough (FJD). | |
3.2 Fresh bread characterization
Jiu-niang fermented for 36 h was added at different amounts (5%, 10%, 15% and 20%) into the dough for the further incorporation in bread making. Reducing sugar contents of different bread samples are given in Table 1. The addition of Jiu-niang significantly increased the reducing sugar content of bread in a concentration-dependent manner. This was probably due to amylase produced by Rhizopus oryzae during Jiu-niang fermentation. The presence of amylase hydrolyzed starch molecule into monosaccharide, leading to augmentation of reducing sugar content.
Table 1 Physical properties of bread containing different amounts of Jiu-nianga
Bread sample |
Reducing sugar (g/100 g bread) |
Specific volume (mL g−1) |
Crust color |
L |
a |
b |
Results are presented as the mean ± standard deviation. Different small letters within a column represent that the values are significantly different at P < 0.05. |
Control |
9.5 ± 0.3a |
4.0 ± 0.1a |
67.7 ± 1.2c |
8.3 ± 1.1a |
27.0 ± 1.5b |
5% |
10.0 ± 0.2b |
4.0 ± 0.0a |
64.3 ± 0.9bc |
10.7 ± 1.5ab |
26.5 ± 0.8b |
10% |
11.7 ± 0.2c |
4.7 ± 0.0c |
61.3 ± 5.4b |
12.7 ± 3.5bc |
23.1 ± 3.7b |
15% |
13.6 ± 0.0d |
4.7 ± 0.1c |
59.3 ± 3.9b |
13.2 ± 2.3bc |
23.5 ± 2.7b |
20% |
14.8 ± 0.2e |
4.3 ± 0.0b |
52.4 ± 0.9a |
16.0 ± 0.4c |
16.9 ± 2.5a |
Specific volume is an important indicator to measure the quality of bread which reflects dough expansion and its capacity to hold gas. The results (Table 1) indicated that addition of Jiu-niang significantly (P < 0.05) increased the value of specific volume except the sample added with 5% Jiu-niang. Jiu-niang is rich in reducing sugar, and can promote yeast growth during dough fermentation to generate a high amount of gas. It was also worth noting that the specific volume suddenly decreased when a large amount (20%) of Jiu-niang was added into dough. This was because excessive amounts of protease resulted in hydrolysis of gluten, therefore destroying dough structure and decreasing gas holding capacity.
Chemical reactions that occur during baking, such as Maillard reactions and caramelization, cause browning and color differences of baked products. The color and flavor formed during baking are related to the reactions between reducing sugars and amino acids.25 The crust of Jiu-niang bread had significantly lower L values and the values of L decreased along with the rising addition of Jiu-niang (Table 1). Increase in redness and decrease in yellowness were found for bread containing Jiu-niang. The darker color of Jiu-niang bread was attributed to its high content of reducing sugar which caused a greater extent of Maillard reactions, thereby intensifying the crust color of final products.
3.3 Texture profile analysis of bread during storage
Hardness, springiness and cohesiveness are the three TPA attributes selected to evaluate the texture of bread in this experiment. Table 2 demonstrates the effects of Jiu-niang concentration and storage time and their interaction on the texture of bread. During 7 days of storage, the hardness gradually increased with increasing storage time both in the Jiu-niang treated bread and the control bread. As was shown in Table 2, the effects of Jiu-niang concentration, storage time and their interaction on the value of hardness were significant (P < 0.0001, P < 0.0001 and P < 0.0001, respectively). Jiu-niang bread showed a lower hardness value and softer texture compared with the control at any time of the storage. The difference of hardness value was not significant of all bread samples at early stage of storage. However, the hardness value of Jiu-niang treated samples was significantly (P < 0.05) lower than that of the control as a function of time. The control sample had the highest hardness value which reached the maximum of 1317.58 g on the seventh day of storage. In addition, the value of hardness decreased at first and then increased as Jiu-niang level increased. Too much addition (20%) could lead to a harder texture of bread which was probably due to large quantities of protease in Jiu-niang. Proper protease content was beneficial to dough preparation as proteins would be degraded into amino acids which were important precursors to form the flavor of bread. When the dough was added with 20% Jiu-niang, large amounts of protease would hydrolyze gluten, resulting to the collapse of dough and deteriorating the appearance and texture of final products.26 10% was an appropriate addition level of Jiu-niang since the bread had the lowest hardness and the highest specific volume (Table 1) values. Addition of 10% Jiu-niang reduced 50% crumb hardness compared with the control on the first day. In general, springiness and cohesiveness decreased as a function of time (Table 2).
Table 2 Effects of Jiu-niang concentration, storage time and their interaction on the texture of different bread samplesa
TPA attributes |
A: Jiu-niang concentration (%) |
B: storage time (d) |
SE |
SL |
1 |
3 |
5 |
7 |
A |
B |
A × B |
A |
B |
A × B |
Results are presented as the mean ± standard deviation. For the same TPA attribute, values with the same small letter at the same storage time for different bread samples and with the same capital letter at different storage times of one bread are not significantly different at P < 0.05. For the same TPA attribute, values with the same small letter at the same storage time for different bread samples and with the same capital letter at different storage times of one bread are not significantly different at P < 0.05. SE: standard error of the mean. SL: least significant difference. |
Hardness |
0 |
343.46aA |
579.73aA |
1139.56bB |
1317.58bB |
26.699 |
23.880 |
53.397 |
<0.0001 |
<0.0001 |
<0.0001 |
5 |
248.45aA |
486.82aAB |
631.48aB |
711.55aB |
10 |
171.08aA |
358.99aAB |
427.79aAB |
541.17aB |
15 |
200.37aA |
421.82aAB |
537.08aB |
706.70aB |
20 |
263.19aA |
541.83aAB |
663.98aB |
1055.46bC |
Springiness |
0 |
0.76aA |
0.75aA |
0.75aA |
0.72aA |
0.006 |
0.006 |
0.013 |
<0.0001 |
0.4610 |
0.7068 |
5 |
0.80aA |
0.80aA |
0.79aA |
0.79bA |
10 |
0.79aA |
0.81aA |
0.80aA |
0.80bA |
15 |
0.79aA |
0.78aA |
0.79aA |
0.80bA |
20 |
0.79aA |
0.79aA |
0.77aA |
0.77abA |
Cohesiveness |
0 |
0.59aA |
0.46A |
0.45aA |
0.42aB |
0.006 |
0.005 |
0.012 |
<0.0001 |
<0.0001 |
0.3408 |
5 |
0.61aA |
0.50aA |
0.46aA |
0.49aB |
10 |
0.63aA |
0.51aA |
0.50aA |
0.51bB |
15 |
0.62aA |
0.48aA |
0.48aA |
0.45aB |
20 |
0.61aA |
0.47aA |
0.46aA |
0.46aB |
The effects of Jiu-niang concentration and storage time on the value of springiness were significant (P < 0.0001, P < 0.0001) whereas their interaction on springiness and cohesiveness were not. For Jiu-niang bread, the change of springiness during storage was not obvious. All bread samples displayed similar springiness and cohesiveness on the first day of storage. However, the results represented a sharp decrease in springiness and cohesiveness for the control sample at the end of storage which would influence consumer's satisfaction of bread. The lower hardness and higher cohesiveness and springiness leaded to a softer texture of bread.
Hardness is a negative indicator of bread textural changes during storage.27 In contrast, cohesiveness, and springiness are normally seen as positive characters to evaluate bread quality. During storage time, these two attributes decreased because of the retrogradation of starch. Currently, amylases are frequently used in bread making. The activity of amylases in dough during fermentation and bread baking impacts several characteristics of bread, including specific volume, firmness and firming rate.28 The hardness of bread is one of the measures of the degree of bread staling. The increased bread softness due to addition of Jiu-niang may be attributed to the presence of amylase in the system. A part of starch molecules could be converted into monosaccharide and dextrin by the action of amylase that is present in Jiu-niang, and thus the long chain and molecular weight of starch was reduced. In such a pathway, the weakness of starch networks particularly the recrystallized amylopectin network was occurring, resulting in the reduction of bread hardness.3 Furthermore, the value of crumb hardness is generally linked to porosity.29 The reducing sugar produced through hydrolyzation was used by yeasts to generate a large amount of gas, which leaded to the high porosity and aeration of Jiu-niang bread and thus decreased the crumb hardness.30
3.4 Moisture migration of bread during storage
After baking, bread crumb showed a higher moisture content than crust which would lead to the redistribution and migration of water between bread crumb and crust. During the time of storage, moisture migrated from crumb to crust, resulted in the decrease of moisture content in bread crumb and the increase in bread crust. Fast moisture migration resulted in dehydration of the bread and leaded to the hardness of bread crumb.31 The results showed that the moisture migration rate of crumb augmented in the first few days and then decreased (Fig. 3). The moisture migration rate of bread crust displayed the similar change tendency. It was possible that water migrated quickly in the first few days and resulted in high values of moisture migration rate. The rate of crumb moisture reduction was relatively lower in Jiu-niang bread, especially the bread supplemented with 10% Jiu-niang. The results demonstrated that the rate of moisture migration (both crumb and crust) of the control bread was much faster. The addition of Jiu-niang could reduce the moisture migration from crumb to crust.
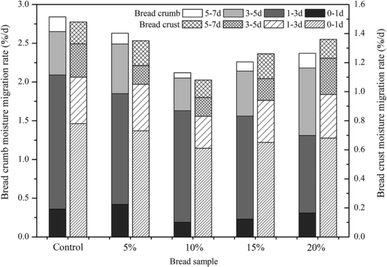 |
| Fig. 3 Moisture migration of bread crumb and crust during storage of 7 days. | |
Moisture migration is one of the most important parameters to measure the degree of bread staling.32 It has been reported by Baik & Chinachoti (2000) that water migrated between crumb and crust was due to the moisture gradient.4 During storage time of bread, there was a redistribution of water when retrogradation of amylopectin occurred. Water migrated from crumb to crust and became immobilized, as more and more water moved into amylopectin crystallites. Amylases hydrolyzed starch granules into smaller molecules, dextrins for example. During bread baking, amylases had effects on starch to form branched chain polymer of dextrins to hold water and then decreased retrogradation of bread.1 Bread with Jiu-niang supplemented had less moisture loss in crumb and low moisture migration rate between crumb and crust, to a certain degree, decrease crystallization of starch.
3.5 Proton distribution of bread during storage
LF-NMR measurement is an effective method to show molecular mobility of small and dynamics molecules and crumb firming in bread during storage time.33 T2 relaxation time obtained by CPMG pulse sequence is a good representative of protons mobility.34 A longer T2 relaxation time indicates a more freedom kind of proton. Three different proton populations (peaking at ∼0.5–0.8 ms, ∼5–6 ms and ∼27–30 ms) were obtained by CPMG pulse sequence (Table 3). Population B was regarded as the major fraction and accounted for 82% of total signal (data not shown). Population A and C encompassed approximately 12% and 6%. The three peaks represented three diverse degrees of proton mobility. Population B was the most abundant which was probably referred to protons of free water in bread crumb. Li et al., (2015) also attributed this part to weakly bound or free water.35 However, besides the mobile OH protons of water, the exchangeable protons of starch and gluten constituted population B as well.36 The determination of different T2 relaxation time was reported in similar products. Curti et al. (2014) investigated the molecular mobility of three gluten enriched breads and found three proton distributions (T2A ∼ 0.2 ms, T2B ∼ 11 ms and T2C > 20 ms) which were attributed to rigid and exchanging protons of starch and gluten interacting with water molecules, more mobile and exchanging protons of starch, gluten and water and mobile protons of lipids, respectively.9 Bosmans et al., (2013) investigated water dynamics and proton mobility in starch and gluten of bread crumb.37 However, four T2 relaxation times (peaking at ∼0.5 ms, ∼2 ms, ∼15 ms and ∼100 ms) were observed by CPMG sequence. The T2 relaxation times at 0.5 ms and 2 ms were represented rigid protons from starch and gluten in little contact with water and exchanging protons of confined water, starch and gluten. The two more mobile populations were attributed to exchanging protons of mobile water, starch and gluten and lipid protons. On the basis of these reports above, the three populations in this experiment were reasonably proposed. Population B was assigned to mobile and exchangeable protons of water, starch and gluten. Population C, the most mobile proton, was assumed to be protons of lipid in bread. The population A had a very short T2 relaxation time could be considered as both rigid and exchangeable protons of starch and gluten in contact with water molecules.
Table 3 T2 relaxation times and areas of proton distributions of bread crumb stored at 25 °C for 7 daysa
Bread sample |
Storage time (d) |
Population A |
Population B |
Population C |
T2 (ms) |
Area |
T2 (ms) |
Area |
T2 (ms) |
Area |
Results are presented as the mean ± standard deviation. Values with the same small letter at the same storage time for different bread samples and with the same capital letter at different storage times of one bread are not significantly different at P < 0.05. |
Control |
1 |
0.6 ± 0.0aA |
1672.6 ± 29.9dB |
6.3 ± 0.1dB |
10 113.3 ± 56.2aB |
27.6 ± 0.9aA |
833.6 ± 56.5aB |
3 |
0.7 ± 0.0aA |
1458.0 ± 31.3bA |
6.1 ± 0.1dA |
10 071.5 ± 35.5aB |
30.6 ± 0.8aB |
639.5 ± 44.4aA |
5 |
0.6 ± 0.1aA |
1491.9 ± 125.5aA |
6.1 ± 0.1dA |
9954.9 ± 105.7aB |
29.1 ± 1.7aAB |
710.9 ± 93.4aAB |
7 |
0.8 ± 0.1abB |
1411.0 ± 20.7bcA |
6.2 ± 0.1dA |
8921.5 ± 829.1aA |
30.23 ± 1.1aB |
756.0 ± 68.7bAB |
5% |
1 |
0.6 ± 0.0bA |
1594.7 ± 42.1cB |
5.9 ± 0.0cB |
10 016.0 ± 187.4aA |
27.7 ± 0.9aA |
840.6 ± 26.8aB |
3 |
0.7 ± 0.1abA |
1489.2 ± 133.3bAB |
5.9 ± 0.2cAB |
9782.6 ± 382.0aA |
30.4 ± 2.8aA |
709.3 ± 70.1abA |
5 |
0.7 ± 0.0abA |
1422.1 ± 5.6aA |
5.8 ± 0.0cAB |
9998.5 ± 35.0aA |
29.8 ± 0.7aA |
672.7 ± 30.1aA |
7 |
0.8 ± 0.1aA |
1449.2 ± 65.5cAB |
5.7 ± 0.1cA |
9784.5 ± 109.2bA |
30.1 ± 0.6aA |
655.3 ± 9.7abA |
10% |
1 |
0.7 ± 0.0cA |
1487.2 ± 44.5bA |
5.6 ± 0.1bA |
9915.7 ± 57.6aA |
28.1 ± 0.9aA |
812.5 ± 28.3aB |
3 |
0.8 ± 0.0bcAB |
1454.1 ± 112.8bA |
5.6 ± 0.1bA |
9996.0 ± 261.8aA |
29.0 ± 0.2aA |
773.4 ± 82.2bAB |
5 |
0.8 ± 0.0bcB |
1370.8 ± 61.0aA |
5.5 ± 0.1bA |
9807.1 ± 217.0aA |
29.7 ± 2.5aA |
687.5 ± 49.9aA |
7 |
0.8 ± 0.0abB |
1375.4 ± 56.0bcA |
5.4 ± 0.1bA |
9804.9 ± 50.0bA |
29.7 ± 2.6aA |
700.7 ± 47.9abA |
15% |
1 |
0.8 ± 0.0dA |
1429.9 ± 39.1abA |
5.5 ± 0.1abB |
9938.0 ± 160.9aA |
27.9 ± 0.4aA |
816.7 ± 40.6aB |
3 |
0.9 ± 0.0cdA |
1295.3 ± 1.9aA |
5.4 ± 0.0abAB |
9799.4 ± 104.0aA |
28.7 ± 0.8aA |
677.1 ± 51.1abA |
5 |
0.9 ± 0.1cA |
1351.4 ± 152.2aA |
5.3 ± 0.1abA |
9695.1 ± 236.3aA |
28.5 ± 1.3aA |
627.7 ± 25.2aA |
7 |
0.8 ± 0.1abA |
1311.7 ± 85.7abA |
5.3 ± 0.1bA |
9781.4 ± 326.0bA |
27.8 ± 0.8aA |
682.4 ± 106.9abA |
20% |
1 |
0.8 ± 0.0dA |
1411.9 ± 12.5aB |
5.4 ± 0.0aD |
9948.0 ± 19.6aB |
27.6 ± 0.2aA |
785.9 ± 0.8aB |
3 |
0.9 ± 0.0dB |
1267.3 ± 1.1aA |
5.3 ± 0.0aC |
9712.0 ± 25.9aA |
28.6 ± 0.3aA |
663.5 ± 2.1abAB |
5 |
0.8 ± 0.1bcAB |
1327.6 ± 117.9aAB |
5.3 ± 0.1aB |
9930.2 ± 110.7aB |
27.0 ± 1.5aA |
741.2 ± 156.8aAB |
7 |
1.0 ± 0.1bB |
1251.0 ± 14.9aA |
5.2 ± 0.0aA |
9721.3 ± 113.1bA |
29.5 ± 3.0aA |
610.1 ± 66.5aA |
The data for T2 relaxation times of protons distributions stored at 25 °C of bread crumb are shown in Table 3. During the storage, both the peak area and the average peak position of population B is dramatically reduced, suggesting that the reduction of mobile and exchangeable protons of starch, gluten and water, as well as the declination of water mobility. Bread with Jiu-niang added had a relatively shorter relaxation time of peak B and the peak time became shorter as the addition of Jiu-niang increased. The high soluble solids content of Jiu-niang increased the viscosity of dough and then decreased the mobility of water, leading to the shorter T2 relaxation time of bread. Moreover, the area of population B decreased much faster in control bread than Jiu-niang treated bread. The peak strength of population B changed during storage period as the protons with a large degree of mobility could move to places where they fixed more tightly. Bread added with 10% Jiu-niang had minimal changes in population B. On the contrary, after 7 days of storage, the peak area of the control was down to 8921.5, which was the lowest among the other samples.
The area of population A, containing both rigid and exchangeable protons of starch and gluten in contact with water molecules, was initially lower in Jiu-niang treated bread. In addition, the area of population A decreased as the concentration of Jiu-niang augmented. These differences showed that the addition of Jiu-niang resulted in hydrolysis of starch molecules and finally reduced signal intensity of population A. There was a significant decrease in the area of population A of the control at the end of the storage. Meanwhile, Jiu-niang bread, especially the dough treated with 10% Jiu-niang, showed no obvious changes in population A. The retrogradation of amylopectin resulted in a shift of the protons of this part to form crystalline structures with shorter relaxation time, shown as a decrease area of population A.37 Jiu-niang effectively reduced the shift in protons to lower mobility and the formation of amylopectin crystals. During the storage, Jiu-niang bread showed a lower moisture migration rate and less water loss (Fig. 3) in crumb owing to the capacity to hold water of starch network. At a molecular level, a smaller decrease in population A indicated that only a small amount of protons shifted to population with shorter relaxation time and formed amylopectin crystals during the storage. The prevention of amylopectin crystal formation leaded to a less rigid, continuous and crystalline starch network with water included.38 These differences were possible due to the action of amylase. The anti-staling efficiency of amylases in bread can be correlated to the limitation degrees of the formation and the strength of the permanent amylopectin network, as well as the water immobilization, thus, controlled starch retrogradation and bread staling.3
3.6 Physico-chemical properties of Jiu-niang
The changes of pH and soluble solids content (SSC) as a function of fermentation time are shown in Fig. 4a. The value of pH gradually reduced from 3.7 to 3.3 during fermentation. The production of acids such as lactic acid by Rhizopus oryzae caused the acidification of the medium. The SSC quickly increased in early fermentation period and then trended to be stable from 18 to 54 h. The value of SSC reached the maximum of 39.8% at 36 h. The soluble solids in Jiu-niang were mainly composed of reducing sugars and some other ingredients such as acids, vitamins and minerals. In the fermentation process of Jiu-niang, Rhizopus oryzae plays an important role in the saccharification of starch. The production of reducing sugars is consumed by yeasts for their growth. This consumption will lead to release of gas.39
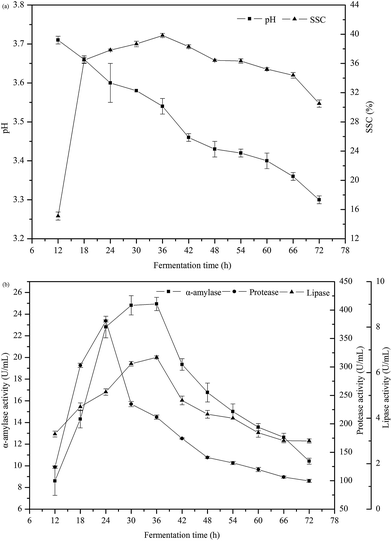 |
| Fig. 4 (a) Changes of pH and soluble solids content (SSC) in Jiu-niang as a function of fermentation time. (b) Principal enzymes activities in Jiu-niang as a function of fermentation time. | |
Bread quality was improved probably due to enzymes produced during solid-state fermentation using glutinous rice as a nutrient source. α-Amylase, protease and lipase were three principal enzymes produced by Rhizopus oryzae. Results of these enzymes activities can be found in Fig. 4b. The activity of α-amylase increased quickly from 12 to 36 h and then decreased. The value reached the maximum at 36 h of 24.9 U mL−1. The α-amylase positively acted on bread texture by hydrolyzing starch into low molecular weight dextrins and then interfered with the amylopectin retrogradation occurring during bread storage. The α-amylase in Jiu-niang had an effect on the development of bread structure, leading to a softer, more springy and more cohesive product. Similar to α-amylase, the maximum value of lipase activity reached at 36 h of 6.7 U mL−1. For the protease, the activity rose sharply until reached the highest value of 380.9 U mL−1 and then substantially decreased. Enzyme activity was closely related to the growth situation of mould. Rhizopus oryzae grew rapidly at the beginning of the fermentation, resulting in large production of enzymes. There was then a decline in enzyme activity which indicated that the amount of moulds began to decrease. High enzyme activity of protease and too much acidification of dough caused degradation of gluten and hindered the formation of gluten network.40 According to the results above, the optimum addition amount found was 10%.
4. Conclusions
Jiu-niang could be applied as a natural enzyme cocktail in bread baking which influenced several characteristics and offered a good overall quality of bread. Addition of Jiu-niang obviously increased specific volume of bread, reduced bread hardness and moisture migration from crumb to crust. At a molecular level, small decreases in population A (both rigid and exchanging protons of starch and gluten in contact with water molecules) and B (mobile and exchangeable protons of water, starch and gluten) of Jiu-niang bread were observed by LF-NMR, which indicated that only a small amount of protons shifted to population with shorter relaxation time and formed amylopectin crystals during the storage. In addition, the optimum addition amount of Jiu-niang was 10% as highest specific volume, lowest hardness and minimum changes in moisture migration and proton mobility were detected for bread supplemented with 10% Jiu-niang.
Funding information
This project was funded by the National High Technology Research and Development Program of China (863 Program, 2011AA100903), Key Projects in the National Science & Technology Pillar Program (2013BAD18B01-4) and National Undergraduates Innovating Experimentation Project (201410307054).
Conflict of interest
The authors declare that they have no competing interests.
Abbreviations
LF-NMR | Low-field nuclear magnetic resonance |
HPLC | High performance liquid chromatography |
SDS-PAGE | Sodium dodecyl sulfate polyacrylamide gel electrophoresis |
TPA | Texture profile analysis |
SSC | Soluble solids content |
Acknowledgements
The authors would like to thank Jiangsu Collaborative Innovation Center of Meat Production and Processing, Quality and Safety Control for some technical supports.
References
- W. Lin and D. R. Lineback, Starch/Staerke, 1990, 42, 385–394 CrossRef CAS.
- L. M. Hallberg and P. Chinachoti, J. Food Sci., 2002, 67, 1092–1096 CrossRef CAS.
- H. Goesaert, L. Slade, H. Levine and J. A. Delcour, J. Cereal Sci., 2009, 50, 345–352 CrossRef CAS.
- M. Y. Baik and P. Chinachoti, Cereal Chem., 2000, 77, 484–488 CrossRef CAS.
- G. M. Bosmans, B. Lagrain, N. Ooms, E. Fierens and J. A. Delcour, Food Chem., 2014, 165, 149–156 CrossRef CAS PubMed.
- L. Slade and H. Levine, Crit. Rev. Food Sci. Nutr., 1991, 30, 115–360 CrossRef CAS PubMed.
- A. Imberty and S. Perez, Biopolymers, 1988, 27, 1205–1221 CrossRef CAS.
- E. Vittadini and Y. Vodovotz, J. Food Sci., 2003, 68, 2022–2027 CrossRef CAS.
- E. Curti, E. Carini, G. Tribuzio and E. Vittadini, LWT--Food Sci. Technol., 2014, 59, 418–425 CrossRef CAS.
- M. L. Martin and R. C. Hoseney, Cereal Chem., 1991, 68, 503–507 CAS.
- J. H. Kim, T. Maeda and N. Morita, Food Res. Int., 2006, 39, 117–126 CrossRef CAS.
- J. K. Purhagen, M. E. Sjöö and A. C. Eliasson, Food Hydrocolloids, 2011, 25, 1656–1666 CrossRef CAS.
- S. P. Cauvain and N. Chamberlain, J. Cereal Sci., 1988, 8, 239–248 CrossRef CAS.
- T. Li, C. Tu, X. Rui, Y. Gao, W. Li, K. Wang, Y. Xiao and M. Dong, J. Agric. Food Chem., 2015, 63, 3261–3270 CrossRef CAS PubMed.
- B. Chen, Q. Wu and Y. Xu, Int. J. Food Microbiol., 2014, 179, 80–84 CrossRef CAS PubMed.
- J. Zhong, X. Ye, Z. Fang, G. Xie, N. Liao, J. Shu and D. Liu, Food Control, 2012, 28, 151–156 CrossRef CAS.
- F. Zhu, R. Sakulnak and S. N. Wang, Food Chem., 2016, 194, 1217–1223 CrossRef CAS PubMed.
- A. A. L. Tribst and M. Cristianini, Innovative Food Sci. Emerging Technol., 2012, 13, 107–111 CrossRef CAS.
- D. Senyay-Oncel, A. Kazan and O. Yesil-Celiktas, Biochem. Eng. J., 2014, 92, 83–89 CrossRef CAS.
- J. Yang, X. Ma, Z. Zhang, B. Chen, S. Li and G. Wang, Biotechnol. Adv., 2010, 28, 644–650 CrossRef CAS PubMed.
- J. Y. Lim, J. J. Kim, D. S. Lee, G. H. Kim and J. Y. Shim, Food Chem., 2010, 120, 255–260 CrossRef CAS.
- C. Wu, R. Liu, W. Huang, P. Rayas-Duarte, F. Wang and Y. Yao, J. Cereal Sci., 2012, 56, 127–133 CrossRef CAS.
- H. Tang, S. He, F. Peng, R. Wang, Q. Li and Y. Ma, RSC Adv., 2016, 6, 102617–102625 RSC.
- P. Wang, Z. Jin and X. Xu, Trends Food Sci. Technol., 2015, 46, 189–198 CrossRef CAS.
- M. Heitmann, E. Zannini and E. K. Arendt, J. Cereal Sci., 2015, 63, 49–56 CrossRef CAS.
- A. Matsuo, K. Sato, E. Y. Park, Y. Nakamura and K. Ohtsuki, J. Funct. Foods, 2012, 4, 513–519 CrossRef CAS.
- A. S. Szczesniak, J. Food Sci., 1963, 28, 385–389 CrossRef.
- B. Lagrain, P. Leman, H. Goesaert and J. A. Delcour, Food Res. Int., 2008, 41, 819–827 CrossRef CAS.
- H. Chanvrier, E. Jakubczyk, E. Gondek and J. Gumy, Innovative Food Sci. Emerging Technol., 2014, 24, 61–68 CrossRef.
- S. Hug-Iten, F. Escher and B. Conde-Petit, Cereal Chem., 2003, 80, 654–661 CrossRef CAS.
- L. Piazza and P. Masi, Cereal Chem., 1995, 72, 320–325 CAS.
- D. E. Rogers, K. J. Zeleznak, C. S. Lai and R. C. Hoseney, Cereal Chem., 1988, 65, 398–401 CAS.
- G. M. Bosmans, B. Lagrain, J. L. Deleu, E. Fierens, B. P. Hills and J. A. Delcour, J. Agric. Food Chem., 2012, 60, 5461–5470 CrossRef CAS PubMed.
- S. G. Choi and W. L. Kerr, LWT--Food Sci. Technol., 2003, 36, 105–112 CrossRef CAS.
- Z. Li, C. Deng, H. Li, C. Liu and K. Bian, Food Chem., 2015, 179, 257–262 CrossRef CAS PubMed.
- A. S. Hager, G. M. Bosmans and J. A. Delcour, J. Agric. Food Chem., 2014, 62, 5682–5689 CrossRef CAS PubMed.
- G. M. Bosmans, B. Lagrain, N. Ooms, E. Fierens and J. A. Delcour, J. Agric. Food Chem., 2013, 61, 4646–4654 CrossRef CAS PubMed.
- G. M. Bosmans, B. Lagrain, E. Fierens and J. A. Delcour, Food Chem., 2013, 61, 6525–6532 CrossRef CAS PubMed.
- A. E. León, E. Durán and C. Benedito de Barber, J. Agric. Food Chem., 2002, 50, 1416–1419 CrossRef.
- C. Thiele, S. Grass and M. Ganzle, J. Agric. Food Chem., 2004, 52, 1307–1314 CrossRef CAS PubMed.
|
This journal is © The Royal Society of Chemistry 2017 |