DOI:
10.1039/C6FO01436D
(Paper)
Food Funct., 2017,
8, 333-340
Mixing behaviour of WPI–pectin-complexes in meat dispersions: impact of biopolymer ratios
Received
30th September 2016
, Accepted 9th December 2016
First published on 12th December 2016
Abstract
Particulated complexes composed of oppositely charged biopolymers were incorporated into highly concentrated protein matrices as potential fat replacers and structuring agents. A multistep procedure was therefore utilized to generate process-stable complexes, which were subsequently embedded into emulsion-type sausages, whereas macro- and microstructural properties were then assessed. Firstly, stock WPI and sugar beet pectin solutions were mixed under neutral conditions (pH 7) at various biopolymer ratios r (2
:
1, 5
:
1, 8
:
1). Secondly, the pH of the biopolymer mixture was decreased to 3.5 to promote associative complexation. Thirdly, electrostatically attracted biopolymer particles were subjected to a heat treatment (ϑ = 85 °C, 20 min) to enhance their stability against superimposed stresses. Finally, fat-reduced emulsion-type sausages were fabricated containing stable WPI–pectin complexes. The results revealed that the heat treatment increased the pH-stability of the biopolymer complexes. In addition, textural and sensorial analysis demonstrated that the meat products became increasingly soft as the biopolymer ratio r increased. This effect was attributed to thermodynamic incompatibility between meat proteins and beet pectin. The results obtained from this study might have important implications for the fabrication of processed meat products with reduced fat levels.
1. Introduction
In recent years, the demand for functional foods promoting health and wellbeing of consumers has steadily increased.1,2 The consumption of processed fats derived from animals is often associated with an increased risk of health problems and becoming overweight.3 In particular, processed meat products and sausages have fat contents ranging from 30 up to 70%. As such, food scientists and manufacturers have considerable interest in reducing the overall lipid content of foods to combat obesity, coronary diseases or diabetes.4 The design of foods with reduced fat content is however challenging and has already been extensively studied.5,6 In particular, lipids are known to act as reservoirs for lipophilic flavor compounds, thereby contributing to the overall taste and flavor sensation. Moreover, fat has also a pronounced impact on the texture and mouthfeel of the final product since it often acts as an active filler in a proteinaceous meat matrix.7 Therefore, replacements of fat content often lead to undesirable alterations in textural and sensorial attributes of the meat batter and sausage.5,8,9
Various approaches have therefore been studied to reduce the overall fat content in processed meat products. Examples of several fat replacers utilized were water and a wide number of hydrocolloids or proteins including inulin, xanthan, carboxymethyl cellulose, pectin, and soy protein – just to name a few.6,10,11 In general, it was shown that a fat reduction in meat sausages resulted in a rubbery and dry texture, whereas hardness, gumminess, and chewiness were increased.9,12 Moreover, a small reduction of fat in meat formulations caused lower juiciness and higher redness values in comparison with the full-fat counterparts.13 Therefore, novel fat replacers with tailor-made properties in terms of texture and sensory profiles are needed to produce high quality foods which are low in calories.
Recently, the utilization of electrostatic protein–polysaccharide complexes as potential fat replacers, structure modifiers, and emulsifying agents has been introduced.14–16 It was demonstrated that the micro- and macrostructural properties (e.g. morphology, shape, size, and charge) of complexes composed of WPI and pectin can be simply modulated by changing intrinsic (e.g. biopolymer concentration and degree of esterification) and extrinsic parameters (e.g. pH, salt, and temperature).17,18 As such, the results revealed that complex formation occurred at pH values below the proteins’ isoelectric point due to strong electrostatic attraction between oppositely charged biopolymers.16,19 In addition, mixed biopolymer complexes composed of WPI and low-methylated pectins consisted of small and loosely linked particles, whereas a high degree of esterification led to the formation of large and dense aggregates with particle sizes ranging between 1 and 10 μm – a fact that highlights their potential as fat mimetics in processed meat products.16,17,20 In general, biopolymers are capable of binding high quantities of water, which might provide lubricity and melting sensation to meat products.21
The possible interaction scenarios of proteins and polysaccharides have been extensively studied in solutions at low overall biopolymer content.19,22 However, in-depth investigations using biopolymer complexes composed of electrostatically attracted WPI and pectin as fat replacers in highly concentrated protein matrices have been rarely carried out. In an early study, it was demonstrated that WPI–pectin complexes could be embedded into a skim milk yoghurt as fat replacers showing similar texture profiles to their full-fat control.20
As such, the objective of the present study was to establish a better understanding of the mixing behaviour of WPI–pectin complexes in processed meat dispersions. For that purpose, biopolymer complexes at various WPI–pectin ratios r (2
:
1, 5
:
1, 8
:
1) were formed under acidic conditions and subsequently heat treated to enhance their process stability. Heated complexes were then added to emulsion-type sausages to assess their impact on structural and functional properties of the batter and sausage. We hypothesized that biopolymer complexes might be easily incorporated into the coherent protein network, whereas single biopolymers might phase separate with meat proteins due to thermodynamic incompatibility. The information obtained from this study might be useful for designing low-fat meat products enriched with fibers and/or carbohydrates.
2. Materials and methods
2.1. Materials
Whey protein isolate (WPI 895, batch no. AX26) was purchased from Fonterra (Auckland, New Zealand). According to the manufacturer's specifications, the WPI sample contained 93.9% protein, 4.7% moisture, 0.3% fat, 0.4% carbohydrates, and 1.5% minerals, respectively. Sugar beet pectin (SBP, Betapec RU 301, batch no. 1 13 12 646) was donated by Herbstreith & Fox KG (Neuenbürg, Germany) and had a degree of esterification of 55%. Pork meat and pork fat were purchased from MEGA eG (Stuttgart, Germany) and standardized to S II and S VIII, according to the GEHA meat classification system.7 Nitrite curing salt (NCS, 0.5% nitrite) was obtained from Zentrag eG (Frankfurt, Germany). Spice mixture (Meisterklasse, Fleischwurst S), sodium di-phosphate (Bullifos LL), and ascorbic acid (E 300) were purchased from Gewürzmüller GmbH (Ditzingen, Germany). Bradford reagent Roti®-Quant (batch no. K015.1), sodium chloride (NaCl, ≥99%), sodium hydroxide (NaOH, ≥99%, p.a., batch no. 6771.5), and hydrochloric acid (HCl, ≥32%, p.a., batch no. P074.4) were obtained from Carl Roth GmbH & Co. KG (Karlsruhe, Germany). All samples prepared are expressed on a weight basis (w/w %).
2.2. Formation and characterization of process-stable WPI–pectin-complexes
2.2.1. Thermal treatment of electrostatic biopolymer complexes.
WPI–pectin complex formation was carried out using an associative complexation approach described in previous studies but with some modifications.17,18,22 Briefly, stock suspensions of WPI and beet pectin were prepared by suspending specified amounts of biopolymers in distilled water at room temperature (Table 1), whereas a high shear homogenizer (Silent Crusher M with dispersing element 22 G, Heidolph Instruments GmbH & Co. KG, Schwabach, Germany) at 20
000 rpm was used to properly mix the suspensions. The final biopolymer concentration in the complex suspensions remained constant at cbiopolymer = 25% ((w/w); equivalent to 3% (w/w) in the sausage formulation). After complete hydration, the pH was adjusted to 7 with NaOH (1 M), whereas stock biopolymer solutions were mixed at various WPI–pectin ratios r (2
:
1, 5
:
1, 8
:
1). The pH was subsequently decreased to induce complexation at pH 3.5 by adding dropwise hydrochloric acid (1 M) to the biopolymer dispersions. All complexes generated were heated at ϑ = 85 °C for 20 min to increase their pH-stability. Before incorporation into the meat batter, the pH of the complex dispersions was re-adjusted to 5.8 to avoid pH-induced structural and sensorial changes in the emulsion-type sausages.
Table 1 Composition (w/w %) of full fat emulsion-type sausages and their fat-reduced counterparts enriched with WPI–pectin complexes
Sample |
Pork meat (%) |
Pork fat (%) |
Water (%) |
Biopolymer |
WPI (%) |
Beet pectin (%) |
Full fat standard |
50 |
25 |
25 |
— |
— |
Water control |
50 |
12.5 |
37.5 |
— |
— |
WPI control |
50 |
12.5 |
34.38 |
3.12 |
— |
Pectin control |
50 |
12.5 |
34.38 |
— |
3.12 |
WPI–pectin 2 : 1 |
50 |
12.5 |
34.38 |
2.08 |
1.04 |
WPI–pectin 5 : 1 |
50 |
12.5 |
34.38 |
2.60 |
0.52 |
WPI–pectin 8 : 1 |
50 |
12.5 |
34.38 |
2.78 |
0.34 |
Nitrate curing salt (%) |
|
|
1.8 |
|
|
Spice blend (%) |
|
|
0.5 |
|
|
Di-phosphate (%) |
|
|
0.1 |
|
|
Ascorbic acid (%) |
|
|
0.05 |
|
|
2.2.2. Surface charge (ζ-potential) measurements.
Single biopolymer solution and complex suspensions were diluted to a concentration of approximately 0.005% with an appropriate buffer. Diluted dispersions were then loaded into a cuvette of a particle electrophoresis instrument (Nano ZS, Malvern Instruments, Malvern, UK), and the ζ-potential was determined by measuring the direction and velocity that the particles moved in the electric field applied. The ζ-potential measurements were reported as the means and standard deviations of measurements made from two freshly prepared samples, with four readings made per sample.
2.2.3. Optical microscopy.
All samples were gently mixed prior to analysis to ensure homogeneity. 10 μL of each sample were placed on a microslide and a glass coverslip was carefully slid on top. Light microscopy images were taken with an axial mounted Canon Powershot G10 digital camera (Canon, Tokyo, Japan) mounted on an Axio Scope optical microscope (A1, Carl Zeiss Microimaging GmbH, Göttingen, Germany).
2.3. Preparation of processed meat dispersions – formation and analysis of fat-reduced emulsion-type sausages
A standard sausage formulation (50% pork meat, 25% pork fat, and 25% water) was utilized to prepare full-fat emulsion-type sausages, whereas 50% of the fat was replaced by incorporating complex dispersions with various biopolymer ratios r (2
:
1, 5
:
1, and 8
:
1) to obtain fat-reduced sausages. Additional formulations were prepared with solutions containing either the single biopolymers (WPI, beet pectin) or water and were used as control samples. Initially, the meat and fat were separately passed through a meat grinder (3 mm endplate, WD-114, Seydelmann KG, Stuttgart, Germany) and half of the water (as ice), 1.8% nitrite-enriched curing salt, and 0.1% sodium di-phosphate were added. The mixture was chopped until a temperature of 3.5 °C was reached. Minced fat, 0.05% ascorbic acid, and 0.5% of the spice blend were added, whereas the meat batter was further processed until a temperature of 10 °C was reached. Subsequently, the ice water remaining was added to achieve a temperature of 6 °C. Biopolymer complexes as well as control dispersions (WPI, pectin, and water) were then incorporated and mixed for 105 s to obtain fat-reduced batters. The full-fat and fat-reduced meat batters were filled in plastic casings (inline coated cellulose casing, Nano Top, 60 mm diameter, Kalle, Wiesbaden, Germany) and thermally processed for 90 min at 76 °C. After heating, the sausages were subsequently stored at 2 °C prior to analysis. The final biopolymer concentration in each fat-reduced emulsion-type sausage was cbiopolymer = 3% (w/w).
2.3.1. Textural analysis.
A modified procedure was utilized to measure the firmness of the emulsion-type sausages using an Instron texture analyzer (model 1011, Instron Corporation Ltd, Canton, MA, USA).25 The measuring device was equipped with a Kramer shear cell (ten blades; each of 7.0 × 0.3 cm). The sausage samples were initially sliced (0.5 cm height and 4.5 cm diameter) and subsequently packed into plastic films to avoid any drying. The slices were then placed into the Kramer shear cell and compressed, penetrated, and sheared by the blades at a speed of 50 mm min−1. The force measured as a function of deformation obtained the maximum yield force F (N per 100 g), which was used as a firmness indicator. 10 slices were analyzed per sample.
2.3.2. Sensory evaluation.
The firmness of the emulsion-type sausages was evaluated by 20 trained panellists using a rank sum test. The ranks varied from 1 for the firmest sausages to 7 for the softest sausages. Sensory evaluation was performed using a standardized sensory setup according to DIN 10950:2012-10.26
2.3.3. Confocal scanning microscopy.
The microstructural properties of the emulsion-type sausages were characterized using a confocal laser scanning microscope (Nikon D-Eclipse C1, pinhole 30 μm, Nikon GmbH, Düsseldorf, Germany) equipped with a red diode laser (excitation wavelength: 638 nm, 25 mV, Melles Griot GmbH, Didam, Netherlands) and a blue argon-ion laser (excitation wavelength: 488 nm, 10 mV, Melles Griot GmbH, Didam, Netherlands). Sausage slices (0.01 cm height and 1.0 cm diameter) were stained using Calcofluor white (Sigma Aldrich, Steinheim, Germany) for protein visualization, whereas Nile red (Sigma Aldrich, Steinheim, Germany) was utilized to highlight the fat particles. All stained samples were covered with aluminium foil to avoid light-induced bleaching, whereas 10 images were taken per sample at 100-fold magnification (TU Plan Fluor Nikon, 100×, 1.30). The images were then analyzed using Nikon's software (EZ-C1, Gold version 3.70).
2.4. Preparation and analysis of model meat dispersions
The mixing behaviour of meat proteins and heat-treated biopolymer complexes was investigated in model meat dispersions (pH 5.8, 2% NaCl). Therefore, meat proteins were initially extracted with an isoionic sodium chloride solution (2%) to obtain a solubilized fraction using a high shear blender (Stephan Cutter UMC 5 electronic, Stephan und Söhne GmbH & Co., Hameln, Germany). The suspension was then centrifuged at 24
040g and 4 °C for 15 min.23 The supernatant was subsequently filtered, whereas the solubilized protein content was determined by the Bradford assay.24
Stock meat protein and biopolymer complex solutions were then mixed and incubated for 24 h at 5 °C to investigate their phase separation behaviour in model meat dispersions (pH 5.8, 2% NaCl). The final complex concentration was cbiopolymer = 1.0% (w/w).
2.5. Statistical analysis
All experiments were repeated at least twice using freshly prepared samples and the means and standard deviations were calculated from these data using Excel (Microsoft, Redmond, VA, USA).
3. Results and discussion
3.1. Impact of pH and heat on the formation of process-stable WPI–pectin complexes
The purpose of this set of experiments was to generate process-stable biopolymer complexes which could be incorporated into emulsion-type sausages (pH 5.8, 2% NaCl) as potential fat replacers or texturing agents. Our results indicated that both pH and heat had a clear impact on the microstructural and electrical properties of the biopolymer dispersions (Fig. 1 and 2). Under neutral pH conditions, no complexation was observed due to strong electrostatic repulsion between similarly charged WPI and beet pectin molecules – a phenomenon that is well known in mixed biopolymer systems and referred to as co-solubility.27,28 Moreover, the miscibility of biopolymers is also known to depend on the molecular characteristics (molecular weight, conformation, flexibility, and charge) of the two species present. However, associative complexation was induced as the pH decreased from 7 to 3.5 regardless of the biopolymer ratio (Fig. 2). In our previous study, we could demonstrate that the electrical charge of the complexes became increasingly positive as the biopolymer ratio r (2
:
1 < 5
:
1 < 8
:
1) increased, suggesting that the pectin backbone became saturated with WPI molecules.22 These results are in line with the previously published literature.16,19,22,29 Moreover, a heat treatment (ϑ = 85 °C, 20 min) above the proteins’ denaturation temperature enhanced the pH-stability of the WPI–pectin complexes (Fig. 2). As such, heated biopolymer complexes kept their particulate matter after increasing the pH from 3.5 to 5.8, whereas non-heated complexes dissociated under the given conditions – an important prerequisite to incorporate complexes into emulsion-type sausages with pH values typically ranging between 5.8 and 6.0.7,30 It was already indicated that thermally treated biopolymer particles composed of β-lactoglobulin and pectin were stable to aggregation over a broad pH range.17,18 Jones et al. (2009)18 showed that heated biopolymer particles remained intact from pH 3 to 7 at sufficiently high polysaccharide concentrations. In addition, thermally concentrated complex dispersions could be generated and easily re-dispersed while maintaining their particulate structures.22 It was assumed that reactive sulfhydryl groups and hydrophobic patches were exposed leading to the formation of stable WPI–pectin complexes.
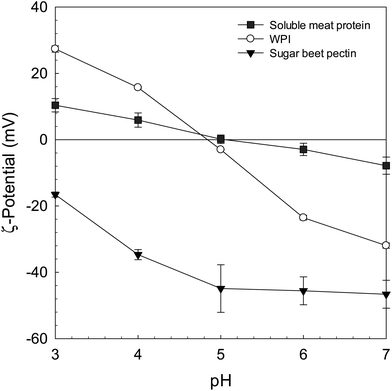 |
| Fig. 1 Impact of pH (3–7) on the electrical properties (ζ-potential) of WPI, sugar beet pectin, and meat proteins (cbiopolymer = 0.5 w/w %). | |
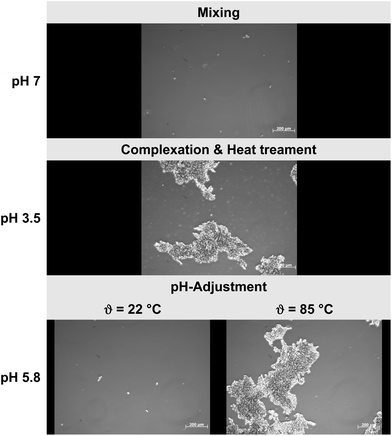 |
| Fig. 2 Microstructural changes of heated (ϑ = 85 °C, 20 min) and non-heated WPI–pectin complexes (pH 3.5, biopolymer ratio r 2 : 1, cbiopolymer = 0.5 w/w %) as a function of pH (scale bar = 200 μm). | |
3.2. Mixing behaviour in processed meat products
In this series of experiments the impact of the addition of biopolymer complexes as potential fat replacers and structuring agents on the textural and sensorial properties of processed meat products was investigated. We therefore fabricated fat-reduced emulsion-type sausages (12.5% fat) rich in stable biopolymer complexes (pH 5.8, cbiopolymer = 25% (w/w)) with various WPI–pectin ratios r (2
:
1, 5
:
1, and 8
:
1), which were subsequently subjected to texture, sensory, and pH determinations. Single biopolymers (WPI, beet pectin), water and a full fat standard were used as control samples. The final biopolymer concentration in the meat products was cbiopolymer = 3% (w/w).
The changes in firmness upon addition of biopolymer complexes to the emulsion-type sausages are shown in Fig. 3. In general, the results indicate that all fat-reduced samples were less firm than the full fat standard regardless of the fat replacer. It was previously shown that the replacement of fat by proteins or hydrocolloids might lead to differences in texture and mouthfeel depending on the substitution level.12,31,32 Moreover, the biopolymer ratio r had a clear impact on the texture of the fat-reduced sausages. Texture profile analysis revealed a decrease of the maximum yield force F from 205.7 N per 100 g to 156.7 N per 100 g as the WPI–pectin ratio r increased from 2
:
1 to 8
:
1. As such, the control sample containing beet pectin as a structuring agent had the lowest firmness value measured leading to a pronounced smooth and soft texture (Fig. 3). These results were supported by a sensorial evaluation. The trained panellists confirmed that emulsion-type sausages became increasingly soft as the biopolymer ratio r decreased, whereas the pectin sample had noticeably the highest rank sum – a fact that highlights its smooth texture (Fig. 3). However, pH-measurements revealed that the incorporation of biopolymer complexes did not change their pH values. All samples tested showed a pH range between 5.8 and 6.0, suggesting that the softening effect of the protein meat matrix was primarily induced by the presence of the biopolymer complexes. These results are in line with previously published studies. Halloran et al. (1997) fabricated a low-fat frankfurter using a high methoxylated pectin as the fat replacer and the final product had an extremely soft texture.6 Furthermore, the addition of carboxymethyl cellulose (CMC) led to a destabilization of the meat batter as indicated by texture measurements.11
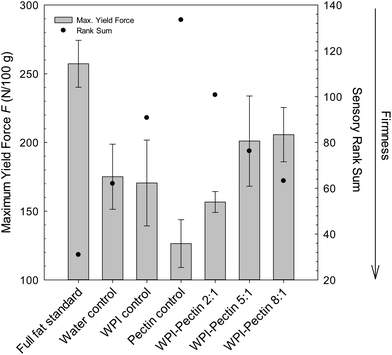 |
| Fig. 3 Textural (maximum yield force F) and sensorial (rank sum) evaluation of fat-reduced emulsion-type sausages (50 w/w % pork meat, 12.5 w/w % pork fat, 12.5 w/w % fat replacer, 25 w/w % water) containing WPI–pectin complexes in various biopolymer ratios r (2 : 1, 5 : 1, and 8 : 1). In addition to a full fat standard, water control, WPI control, and pectin control were used as references. | |
CLSM analysis was further utilized to determine microstructural changes in the meat batter upon complex addition which might help to explain the macroscopic alterations obtained by texture and sensory evaluation. Fig. 4 shows CLSM images of the full fat standard as well as the samples containing WPI–pectin complexes or single biopolymers stained with two different fluorescence dyes, namely Calcofluor white and Nile red. In general, a coherent protein network with finely dispersed fat particles was formed after heating the full fat standard above 72 °C. Upon heating, the meat proteins undergo structural changes affecting the quality parameters of the final product.33 However, the microscopy images taken showed that the microstructure vastly changed when biopolymer complexes were incorporated into the meat batter. As such, the protein network tended to become gradually more disrupted and loose as the biopolymer ratio r increased from 2
:
1 to 8
:
1. In addition, this effect was most pronounced when beet pectin was used, whereas WPI alone seemed to improve the integrity of the protein gel network.6,11 Schuh et al. (2013) also figured out that the proteins were no longer interconnected when CMC was added to the meat batter, whereas the network attained an increasingly fractal dimension.11
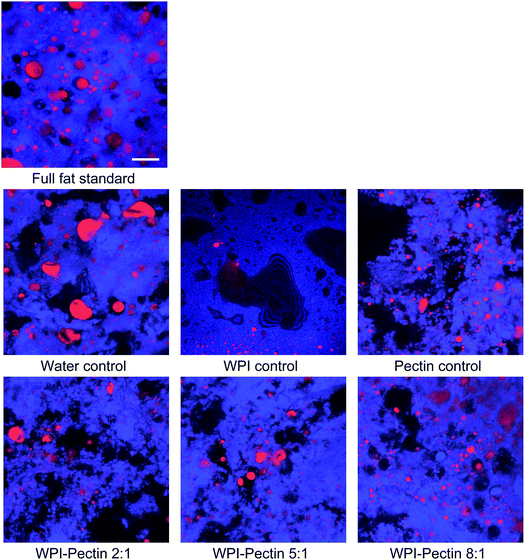 |
| Fig. 4 CLSM images (magnification = 100×) of full fat and fat-reduced emulsion-type sausages (50 w/w % pork meat, 12.5 w/w % pork fat, 12.5 w/w % fat replacer, and 25 w/w % water). The protein network is highlighted in blue, whereas the fat particles are stained in red (scale bar = 50 μm). | |
3.2. Mixing behaviour in model meat dispersions – mechanistic insights
We then examined the mixing behaviour of WPI–pectin complexes with various biopolymer ratios r (2
:
1, 5
:
1, and 8
:
1) and meat proteins in model dispersions (pH 5.8, 2% NaCl) to further gain insights into the unexpected textural and sensorial properties of the processed sausages. WPI and sugar beet pectin were used as control samples. All mixtures prepared were incubated for 24 h at 5 °C before being analyzed. It should be mentioned that the biopolymer concentrations in the processed meat sausages and in the model dispersions differ from each other; however, a rather general assumption of the experimental outcome might be drawn to highlight the miscibility behaviour.
The visual changes in phase separation of model meat dispersions are shown in Fig. 5, whereas the molecular characteristics of the single biopolymers used are presented in Table 2. In general, our results indicated that the phase separation was dominated by the biopolymer type added to the model meat dispersions (3% protein, pH 5.8, 2% NaCl). As such, no phase separation was observed when WPI was added to the mixture, indicating that both, milk and meat proteins were co-soluble under the given conditions (pH 5.8, 2% NaCl). In contrast, a distinct two phase system was formed when only sugar beet pectin was present in the mixture – a phenomenon that is typically referred to as thermodynamic incompatibility (Fig. 5). Thereby, one phase is rich in hydrocolloids and the other one is rich in proteins.11,15 In particular, the Bradford assay revealed a higher protein content in the lower phase (25–30 mg ml−1). Moreover, an increase in phase separation was observed for WPI–pectin complexes as the biopolymer ratio r increased from 2
:
1 < 5
:
1 < 8
:
1, indicating the dominating impact of sugar beet pectin. Previous studies have suggested that the molecular characteristics (molecular weight, conformation, flexibility, and charge) of the two species involved are of key importance to induce such a thermodynamically driven process.34,35 As such, when we analyzed the electrical properties of the mixed dispersions under prevalent conditions (pH 5.8, 2% NaCl), all biopolymers tested were negatively charged, indicating a strong repulsive interaction force (Fig. 1 and Table 2). Moreover, previous studies conducted in our lab have shown that biopolymer complexes composed of WPI and beet pectin were negatively charged at a pH range between 5 and 7.22,36 Moreover, phase separation is typically favoured when the restrictions on the freedom of orientation of the biopolymer molecules reduce the entropy contribution to the overall free energy of the system – a fact that could help explain the incompatibility of beet pectin and meat proteins.37 In particular, excluded volume effects are known to become more pronounced for larger molecules as in the case of beet pectin due to its highly branched nature (Table 2).14,28
 |
| Fig. 5 Mixing behavior of the meat proteins (3 w/w % meat protein, 2 w/w % sodium chloride, pH 5.8) and biopolymers added (1 w/w %): WPI–pectin complexes (ratio r 2 : 1, 5 : 1, and 8 : 1), WPI control, and pectin control. | |
Table 2 Important characteristics (subunits, molecular weight, configuration, charge) of biopolymers added to emulsion-type sausages7,16
Biopolymer |
Subunits (%) |
Configuration |
ζ-Potential at pH 5.8 (mV) |
Molecular weight (kDa) |
Meat protein |
Myosin (50–55) |
Fibrillar |
−2.4 |
400–800 |
Actin (15–20) |
|
|
|
Tropomyosin (4–6) |
|
|
|
|
Whey protein isolate |
β-Lactoglobulin (45) |
Globular |
−19.6 |
18 |
α-Lactalbumin (20) |
|
|
|
Serum albumin (5) |
|
|
|
|
Sugar beet pectin |
|
Linear, branched |
−45.5 |
45 |
4. Conclusion
The present study has shown that the addition of biopolymer particles composed of WPI and pectin clearly impacts textural and sensorial attributes of processed meat products. The results presented in this study, however, highlight the importance of assessing the incorporation of novel engineered particles into real food matrices under the specific conditions (e.g. pH or in the presence of other components) as these can clearly impact their performance. As such, a high pectin content reduced the firmness of the sausages, which was attributed to thermodynamic incompatibility between the meat proteins and the hydrocolloid added. It is clear that the interactions which occur between molecular structures forming the final food matrix need also to be fully understood to achieve their final purpose within the micro- and macro-structure. As such, further studies on this subject should be carried out to gain a better understanding of how the overall complex concentration as well as the fat substitution level might impact the emulsion properties.
Acknowledgements
This research project was supported by the German Ministry of Economics and Energy (via AiF) and the FEI (Forschungskreis der Ernährungsindustrie e.V., Bonn): Project AiF 17876 N.
Notes and references
- B. Zeeb, L. Fischer and J. Weiss, Stabilization of food dispersions by enzymes, Food Funct., 2014, 5, 198–213 CAS.
- D. J. McClements, E. A. Decker, Y. Park and J. Weiss, Structural design principles for delivery of bioactive components in nutraceuticals and functional foods, Crit. Rev. Food Sci. Nutr., 2009, 49, 577–606 CrossRef CAS PubMed.
- F. Jiménez Colmenero, Relevant factors in strategies for fat reduction in meat products, Trends Food Sci. Technol., 2000, 11, 56–66 CrossRef.
- B. Zeeb, C. L. Lopez-Pena, J. Weiss and D. J. McClements, Controlling lipid digestion using enzyme-induced crosslinking of biopolymer interfacial layers in multilayer emulsions, Food Hydrocolloids, 2015, 46, 125–133 CrossRef CAS.
- C. Chung, B. Degner and D. J. McClements, Reduced calorie emulsion-based foods: Protein microparticles and dietary fiber as fat replacers, Food Res. Int., 2014, 64, 664–676 CrossRef CAS.
- J. D. Halloran, R. W. Rogers, S. A. Dyer and D. Ellis, Effectiveness of selected adjuncts on processing and sensory characteristics of extra lean frankfurters, J. Muscle Foods, 1997, 8, 329–346 CrossRef.
-
O. Prändl, Fleisch: Technologie und Hygiene der Gewinnung und Verarbeitung, Ulmer, 1988 Search PubMed.
- E. Cengiz and N. Gokoglu, Effects of fat reduction and fat replacer addition on some quality characteristics of frankfurter-type sausages, Int. J. Food Sci. Technol., 2007, 42, 366–372 CrossRef CAS.
- B. D. Tobin, M. G. O'Sullivan, R. M. Hamill and J. P. Kerry, The impact of salt and fat level variation on the physiochemical properties and sensory quality of pork breakfast sausages, Meat Sci., 2013, 93, 145–152 CrossRef CAS PubMed.
- E. Mendoza, M. L. García, C. Casas and M. D. Selgas, Inulin as fat substitute in low fat, dry fermented sausages, Meat Sci., 2001, 57, 387–393 CrossRef CAS PubMed.
- V. Schuh, K. Allard, K. Herrmann, M. Gibis, R. Kohlus and J. Weiss, Impact of carboxymethyl cellulose (CMC) and microcrystalline cellulose (MCC) on functional characteristics of emulsified sausages, Meat Sci., 2013, 93, 240–247 CrossRef CAS PubMed.
- J. H. Choe, H. Y. Kim, J. M. Lee, Y. J. Kim and C. J. Kim, Quality of frankfurter-type sausages with added pig skin and wheat fiber mixture as fat replacers, Meat Sci., 2013, 93, 849–854 CrossRef PubMed.
- J. Weiss, M. Gibis, V. Schuh and H. Salminen, Advances in ingredient and processing systems for meat and meat products, Meat Sci., 2010, 86, 196–213 CrossRef PubMed.
- A. Matalanis, U. Lesmes, E. A. Decker and D. J. McClements, Fabrication and characterization of filled hydrogel particles based on sequential segregative and aggregative biopolymer phase separation, Food Hydrocolloids, 2010, 24, 689–701 CrossRef CAS.
- E. Scholten, T. Moschakis and C. G. Biliaderis, Biopolymer composites for engineering food structures to control product functionality, Food Struct., 2014, 1, 39–54 CrossRef.
- H. Salminen and J. Weiss, Effect of pectin type on association and pH stability of whey protein—pectin complexes, Food Biophys., 2013, 1–10 Search PubMed.
- K. Protte, C. Bollow, A. Sonne, O. Menéndez-Aguirre, J. Weiss and J. Hinrichs, Impacts on micro- and macro-structure of thermally stabilised whey protein-pectin complexes: A fluorescence approach, Food Biophys., 2016, 1–9 Search PubMed.
- O. G. Jones, E. A. Decker and D. J. McClements, Formation of biopolymer particles by thermal treatment of beta-lactoglobulin-pectin complexes, Food Hydrocolloids, 2009, 23, 1312–1321 CrossRef CAS.
- C. Schmitt and S. L. Turgeon, Protein/polysaccharide complexes and coacervates in food systems, Adv. Colloid Interface Sci., 2011, 167, 63–70 CrossRef CAS PubMed.
- A. Krzeminski, K. A. Prell, M. Busch-Stockfisch, J. Weiss and J. Hinrichs, Whey protein-pectin complexes as new texturising elements in fat-reduced
yoghurt systems, Int. Dairy J., 2014, 36, 118–127 CrossRef CAS.
- S. I. Laneuville, P. Paquin and S. L. Turgeon, Effect of preparation conditions on the characteristics of whey protein - xanthan gum complexes, Food Hydrocolloids, 2000, 14, 305–314 CrossRef CAS.
- C. Stenger, B. Zeeb, J. Hinrichs and J. Weiss, Formation of concentrated biopolymer particles composed of oppositely charged WPI and pectin for food applications, J. Dispersion Sci. Technol., 2016 DOI:10.1080/01932691.2016.1234381.
-
J. Hermanianto, Untersuchungen von Proteinlöslichkeit, Mikrostruktur und Stabilität bei Brühwurstbrät in Abhängigkeit von Salz- und Phosphatkonzentration bzw. Verwendung von Kochsalzersatzmitteln und Flüssigstickstoff, PhD thesis, Universität Hohenheim, 1995 Search PubMed.
- M. M. Bradford, A rapid and sensitive method for the quantitation of microgram quantities of protein utilizing the principle of protein-dye binding, Anal. Biochem., 1976, 72, 248–254 CrossRef CAS PubMed.
- S. Irmscher, S. Rühl, K. Herrmann, M. Gibis, R. Kohlus and J. Weiss, Determination of process-structure relationship in the manufacturing of meat batter using vane pump-grinder systems, Food Bioprocess Technol., 2015, 8, 1512–1523 CrossRef CAS.
- DIN, Sensory analysis - basic principles. 2012. DIN 10950:2012-10. Berlin: Beuth.
- G. Davidov-Pardo, I. J. Joye and D. J. McClements, Food-grade protein-based nanoparticles and microparticles for bioactive delivery: Fabrication, characterization, and utilization, Adv. Protein Chem. Struct. Biol., 2015, 98, 293–325 CAS.
- O. G. Jones and D. J. McClements, Functional biopolymer particles: Design, fabrication, and applications, Compr. Rev. Food Sci. Food Saf., 2010, 9, 374–397 CrossRef CAS.
- O. G. Jones and D. J. McClements, Recent progress in biopolymer nanoparticle and microparticle formation by heat-treating electrostatic protein-polysaccharide complexes, Adv. Colloid Interface Sci., 2011, 167, 49–62 CrossRef CAS PubMed.
- F. Reimold, C. Hildebrand, A. K. Erdös, T. Bantleon and F. Thiemig, Modifizierung von Bindegewebe zur Herstellung energiereduzierter Rohwurst, Fleischwirtschaft, 2015, 3, 134–139 Search PubMed.
- C. M. Pereira, M. F. Marques, M. K. Hatano and I. A. Castro, Effect of the partial substitution of soy proteins by highly methyl-esterified pectin on chemical and sensory characteristics of sausages, Food Sci. Technol. Int., 2010, 16, 401–407 CrossRef CAS PubMed.
- D. F. Keenan, V. C. Resconi, J. P. Kerry and R. M. Hamill, Modelling the influence of inulin as a fat substitute in comminuted meat products on their physico-chemical characteristics and eating quality using a mixture design approach, Meat Sci., 2014, 96, 1384–1394 CrossRef CAS PubMed.
- E. Tornberg, Effects of heat on meat proteins - Implications on structure and quality of meat products, Meat Sci., 2005, 70, 493–508 CrossRef CAS PubMed.
- C. Thongkaew, J. Hinrichs, M. Gibis and J. Weiss, Sequential modulation of pH and ionic strength in phase separated whey protein isolate - Pectin dispersions: Effect on structural organization, Food Hydrocolloids, 2015, 47, 21–31 CrossRef CAS.
- J. L. Doublier, C. Garnier, D. Renard and C. Sanchez, Protein-polysaccharide interactions, Curr. Opin. Colloid Interface Sci., 2000, 5, 202–214 CrossRef CAS.
- B. Zeeb, C. Stenger, J. Hinrichs and J. Weiss, Formation of concentrated particles composed of oppositely charged biopolymers for food applications - impact of processing conditions, Food Struct., 2016, 10, 10–20 CrossRef.
- C. G. De Kruif, F. Weinbreck and R. De Vries, Complex coacervation of proteins and anionic polysaccharides, Curr. Opin. Colloid Interface Sci., 2004, 9, 340–349 CrossRef CAS.
|
This journal is © The Royal Society of Chemistry 2017 |