DOI:
10.1039/C6RA24573K
(Paper)
RSC Adv., 2016,
6, 105487-105494
Poly(vinyl alcohol)–Gantrez® AN cryogels for wound care applications†
Received
2nd October 2016
, Accepted 27th October 2016
First published on 28th October 2016
Abstract
Cryotropic gelation is a low cost, well-known technique that has been used for decades for the preparation of cryogels based on poly(vinyl alcohol). This technique does not require addition of any cross-linkers or initiators because a physical cross-linking takes place during the cryotropic gelation. Poly(vinyl alcohol)–Gantrez® AN cryogels with highly porous structure were successfully produced from the aqueous polymer mixtures with addition of 0.4, 0.6 and 0.8% w/v NaOH, which were frozen at −18 °C for approximately 9 hours and then thawed at room temperature (∼25 °C). These cryogels exhibited excellent mechanical properties (with 158–218% elongation to break) and high swelling ability in water (∼40 g of deionized water could be absorbed per 1 g of initial gel within 48 h). They exhibited intrinsic antimicrobial properties against Staphylococcus aureus without the addition of any antimicrobial agent due to the acidic nature of Gantrez® AN and related low pH of these cryogels. The in vitro biocompatibility of these cryogels was assessed using human dermal fibroblasts with very encouraging results. These cryogels are promising for applications in wound care.
Introduction
Polymeric gels are widely used in the biomedical sector as drug delivery systems, matrices for cells immobilization, scaffolds for tissue engineering, chromatographic materials and wound care products.1,2 Gels can be generally defined as three-dimensional swollen networks entrapping the solvent, which can be water (hydrogels) or an organic liquid (organogels). What crucially determines their physicochemical properties, and even their appearance, is the nature of their cross-linking and the method used for their synthesis. There are two main types of polymeric gels: chemically cross-linked gels, whose chains are linked with covalent bonds, resulting in irreversibly insoluble networks, and physical gels, which are formed by the physical cross-linking of the polymer chains and could potentially be re-dissolved upon changes in environmental conditions.3 One of the most commonly used techniques for the synthesis of physically cross-linked hydrogels is the so-called ‘cryotropic gelation’ (from the Greek ‘κριóς’, frost and ‘τρóπoς’, cause). In this method, the solution of polymers undergoes one or multiple freeze–thawing cycles, which lead to the formation of an internal structure of interconnected pores.4–6 In fact, after reaching the crystallization temperature of the solvent, the system is represented by a heterogeneous block containing solvent crystals and the unfrozen liquid micro-phase (UFLMP), where the reagents quickly concentrate (this phenomenon is called ‘cryoconcentration’) leading to the gelation.2,7 The solvent here acts as a pore-forming agent: when the solvent crystals melt down they leave a macro and micro-porous network inside the gel formed (cryogel). The size of the macro-pores present in the system as well as its mechanical properties such as the elastic modulus, directly depend on the concentration of the gel-forming reagents used and on the number and conditions of the freeze–thawing cycles.8,9
The most popular cryogels are based on poly(vinyl alcohol) (PVA) and are usually obtained after several (up to 8) repeated thermal cycles using water as a solvent (to produce opaque gels) or dimethyl sulfoxide (DMSO, to form transparent gels).9 They have been proposed as materials promising for cells entrapment and delivery, as well as cartilage and cardiac valves replacement and wound healing.10–12 Wound dressings using PVA-cryogels have been shown to be particularly advantageous. It is well-known that hydrogels could accelerate wound healing thanks to their ability to absorb exudate, preventing the dehydration of the wound bed at the same time, and promoting the re-epithelization process.13,14 Moreover, their cooling effect on the skin can provide some pain relief to the patients. Cryogels are better option to traditional dressings in particular when the injury is located in body parts that can be more frequently subjected to physical stress (such as joints) or compression (for example in the case of bed sores).14,15 PVA is a neutral water-soluble synthetic polymer that has been used alone or in combination with other natural or synthetic polymers. It can form the basis of non-toxic, non-carcinogenic, and biocompatible materials with high water content.16 In the present work, PVA was used in combination with poly(methyl vinyl ether-alt-maleic anhydride), another synthetic polymer used in food, pharmaceutical and oral care industries as a thickener and suspending agent, known by its trade name Gantrez® AN.17–19 The two polymers were physically cross-linked by one freeze–thawing cycle to result in novel cryogels for wound care applications. To the best of our knowledge, this is the first study where Gantrez® AN is combined with PVA for cryogels synthesis. The formation of these materials was found to be highly dependent on the addition of NaOH in their aqueous mixture.
Experimental section
Materials
PVA of 98–99% hydrolysis and of Mw 57–66 kDa molecular weight was purchased from Alfa Aesar (UK). The analysis of PVA using 1H NMR and gel permeation chromatography established the actual degree of deacetylation and Mw to be 98.7% and 51.3 kDa, respectively. Nutrient agar and nutrient broth used to perform the antimicrobial properties test were purchased from Oxoid Ltd UK. Gantrez® AN (Mw ∼ 216 kDa) as well as the other chemicals used for this work were purchased from Sigma-Aldrich (UK). AQUACEL® Ag dressing (used as a control for antimicrobial and cytotoxicity tests) was kindly provided by ConvaTec Ltd.
Cryogels synthesis
10% w/v aqueous solution of PVA was prepared by dissolving the polymer in water at 92 °C for approximately 7 h. This solution was mixed with 17.4% w/v aqueous solution of Gantrez® AN, which was previously prepared by dissolving the polymer in water overnight at 40 °C. Previously20 we have reported that the two shoulders at 1846 and 1779 cm−1 typical for maleic anhydride cycles observed in the infrared spectrum of dry Gantrez AN disappear completely in the spectrum of the air-dried films prepared by casting its aqueous solutions due to the hydrolysis of anhydride cycles into COOH groups in the presence of water. The conditions used in that study are very similar to those used for dissolving Gantrez AN in this work, so it could be assumed that the hydrolysis of anhydride cycles is complete during the preparation of Gantrez AN solutions.
Three mixtures with 1
:
1 volume ratio of the two polymers were produced with the addition of NaOH at different concentrations (0.4%, 0.6% and 0.8% w/v), and poured into sealed glass Petri-dishes (8 cm diameter). These were frozen at −18 °C overnight (approximately 9 hours) and then thawed at room temperature (∼25 °C). An opaque, flat, stretchable and very easy to handle gels were formed as a result of this cryogelation.
Scanning electron microscopy (SEM)
Scanning electron microscopy (1000× magnification) was carried out to investigate the porous structure of the materials produced. An FEI Quanta FEG 600 Environmental Scanning Electron Microscope (ESEM) was used to study gold sputter coated, freeze–dried PVA–Gantrez® AN cryogels, produced after one freeze–thawing cycle.
Sol–gel fraction analysis
Insoluble (gel) and soluble (sol) fractions (%) of PVA–Gantrez® AN cryogels were determined using a gravimetric method. The samples were immersed for 48 h in 12 mL of deionized water, which was then freeze–dried (sol 1) and then replaced with fresh water for another 48 h. The swollen gel and the soluble extract (sol 2) were also freeze–dried. Control samples were directly freeze–dried after their synthesis (100% gel fraction). All analyses were conducted in triplicate. Gel and sol fractions were calculated using the following formulae: |
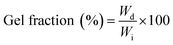 | (1) |
|
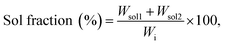 | (2) |
where Wd is the swollen dry gel, Wi is the control (a sample which was not immersed in water but directly freeze–dried), and Wsol1 and Wsol2 represent soluble fractions.
Swelling behaviour of PVA–Gantrez® AN cryogels
The swelling properties of the cryogels were studied in deionized water and in a saline solution simulating the ionic strength of wound exudate (8.298 g NaCl and 0.368 g CaCl2 dissolved in 1 L of deionized water). The test was performed using metallic mesh baskets (2 cm diameter, 3 cm height) placed inside plastic vials (3.5 cm in diameter and 6 cm in height). The materials produced were cut into 3 mm diameter disc shaped flat samples and placed into the metallic baskets. The initial weight of the samples and the weight of each basket used (after being immersed into the medium used for the test and wiped always in the same manner) were accurately recorded.21 The cryogels were kept in excess of medium for 8 days and weighed regularly, by weighing the baskets with the cryogel samples inside. The weights of the baskets were taken into consideration to calculate the weight of the swollen samples. The swelling ratio (SR) of the PVA–Gantrez® AN cryogels was calculated using the following formula:where Wi is the initial gel weight and Ws is the weight of the sample after additional swelling, respectively.
Mechanical properties
A Texture Analyser XT Plus (T.A., by Stable Micro System Ltd, UK) was used to perform the tensile test on the PVA–Gantrez® AN cryogels to assess their mechanical properties. The materials were cut into 20 × 3 mm samples. A ‘grip-to-grip’ separation of 30 mm was used and each sample was stretched, undergoing an extension of 100 mm min−1 to break. Strength–strain curves were recorded during the test.
Cryogels adhesion to porcine skin model
The adhesion of the cryogels to porcine skin was tested using Texture Analyser (Stable Micro System Ltd, UK) in a different set up: cryogel discs (20 mm in diameter) were fixed to the probe of the T.A. and shaved porcine skin (from the back of the animal, provided by P. & D. Jennings Butchers, Hurst, UK) 5 cm × 5 cm specimens were placed on the area below. The following settings were used for the test: pre-speed test 1.0 mm s−1; test-speed 0.5 mm s−1; post-test speed 1.0 mm s−1; applied force 0.5 N; return distance 10.0 mm; contact time 60.0 s; trigger type auto; trigger force 0.1 N; and return distance of 10.0 mm. The adhesive strength, the total work of adhesion and the cohesiveness of the materials produced were calculated from the data collected during this test by the T.A. software (T.A. Exponent) according to the methodology described by Boateng et al.22
Antimicrobial properties
The disk diffusion test23 was used to investigate the antimicrobial properties of PVA–Gantrez® AN cryogels against Staphylococcus aureus NCTC 8532 (National Collection of Type Cultures). Staphylococcus aureus was grown overnight at 37 °C (shaking at 200 rpm) in nutrient broth. Sterilized nutrient agar was prepared as indicated by the manufacturer and used to produce the plates to be inoculated with two bacterial suspensions (108 CFU mL−1 and 104 CFU mL−1, respectively). Cryogel discs (1 cm in diameter) were placed in the centre of the agar plates and incubated for 24 hours at 37 °C. The clear zone around the samples was measured using a ruler to assess bacteria growth inhibition and proliferation.
Biocompatibility of PVA–Gantrez® AN cryogels
The biocompatibility of the cryogels was evaluated using an MTT (3-(4,5-dimethylthiazol-2-yl)-2,5-diphenyl tetrazolium bromide) assay.21,24,25 The extracts of the cryogels and control (AQUACEL® Ag, ConvaTec Ltd) were used for the test. 5 mg of each sample were incubated at 37 °C in 8 mL of Dulbecco's Modified Eagle's Medium (DMEM) containing 2% v/v L-glutamine and 50 μg mL−1 gentamicin. The medium was collected after 1, 2 and 7 days and used to perform the MTT assay. Human dermal fibroblasts were seeded at a density of 8 × 104 cells per mL in complete medium (containing 10% Fetal bovine serum (FBS), 2% L-glutamine and 50 μg mL−1 of gentamicin) in a sterile 96-wells culture plate and cultured to reach confluence. The extracts collected from the materials produced were used to replace the medium. The plates were incubated at 37 °C and 5% CO2 for 24 h. MTT solution was prepared in warm PBS (0.5 mg mL−1) following the manufacturers specification. 100 μL per well of this solution was added to the plates, which were then incubated at 37 °C for 3 h. After removal of MTT and medium, DMSO (100 μL per well) was used to solubilize the formazan present in the cells. A Biotek Synergy HT detector was used to read the absorbance at 570/630 nm.
Sterilization of cryogels by gamma irradiation
The materials produced were sterilized using gamma irradiation26 by Bradford Synergy Health PLC (UK) in accordance with EN ISO 11137-1:2015 requirements for health care products, EN ISO 9001:2008 for quality management system, and EN ISO 13485:2012 for quality system-medical devices. The absorbed radiation dose was 33.3 kGy. The influence of the gamma irradiation on their swelling ability in deionized water and mechanical properties were assessed as described above.
Statistical analysis
The statistical analysis was performed using one-way ANOVA with Bonferroni post-hoc test on Prism (Graphpad software, USA) comparing different groups of data.
Results and discussion
Formation of PVA–Gantrez® AN cryogels
Flat cryogel samples were successfully produced from mixtures of aqueous solutions of PVA and Gantrez® AN (with the addition of NaOH) using the freeze–thawing technique, which involves the cryotropic gelation process and leads to inter- and intra-chains physical cross-linking. Only one thermal cycle is required to form the materials presented in this work. The proposed method is less-time consuming and more cost-effective compared to many techniques commonly used to produce physical hydrogels.3,27,28 It should be noted that PVA–Gantrez® AN cryogels did not form in the absence of NaOH within solutions under the freeze–thaw conditions used in this work. However, an aqueous solution of PVA alone did form cryogels in the absence of NaOH. The synthesis of PVA-based cryogels with the addition of strong bases such as NaOH and KOH has previously been reported; it was demonstrated that PVA-based gels prepared with the addition of NaOH have improved mechanical properties due to increased sample crystallinity.29–32
It could be hypothesized that the addition of NaOH to PVA–Gantrez® AN aqueous mixtures prevents the formation of weak intermolecular hydrogen bonds between –COOH of Gantrez® AN and –OH groups of PVA due to dissociation of carboxylic groups. The formation of more regular –OH⋯HO– hydrogen bonds between PVA chains is then facilitated under these conditions. Additionally, the effect of NaOH on PVA reported in ref. 29–32 could also not be fully ruled out. Moreover, the addition of NaOH to polymer solutions gives a more desirable pH range, 4.4–5.3, for wound care applications.33–35
In the conditions described above, the intra- and inter-chain hydrogen bonding is promoted and takes place more rapidly in the unfrozen liquid micro-phases. In these areas the reagents mixture is highly concentrated because of the formation and expansion of solvent crystals during the freezing step at −18 °C. These expand in the mixture forming a structure that will generate the network of interconnected pores once the crystals melt down during the thawing step at room temperature.4,11 This characteristic internal structure significantly influences the physical and mechanical properties of cryogels, which became very popular materials for different biomedical applications.1,36 SEM analysis allowed us to observe pores morphology and distribution of the PVA–Gantrez® AN cryogels (Fig. 1).
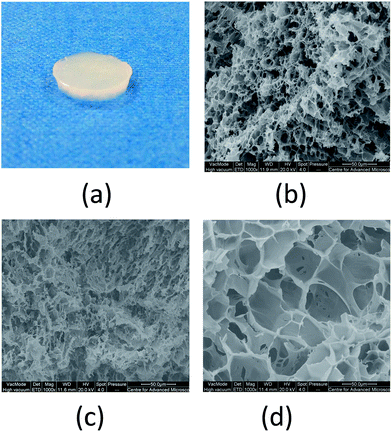 |
| Fig. 1 Macroscopic image of the swollen cryogel with 0.8% NaOH (a) and SEM images of freeze–dried, gold sputter coated samples of PVA–Gantrez® AN formed in the presence of 0.4% (b), 0.6% (c) and 0.8% NaOH (d). | |
All the PVA–Gantrez® AN cryogels present a highly porous structure with different pore sizes and thin gel walls throughout the matrix. Although the pore distribution of the cryogels is very inhomogeneous, it could be noticed that an increase in the concentration of NaOH in the liquid mixture of two polymers generally leads to the formation of larger pores. The pores observed in these samples are also interconnected, and can be then attributed to the formation of large ice crystals and to the consequent water flow through the cryogel matrix during thawing.
It is well known that a second polymer added to PVA solutions in a common solvent may lead to a liquid–liquid phase separation due to the thermodynamic incompatibility between these materials, which affects the formation of pores.37–39 It should be noted that PVA–Gantrez® AN forms completely transparent aqueous mixtures without addition of NaOH (Fig. S1, ESI†). However, the addition of NaOH to PVA–Gantrez® AN mixtures results in formation of slightly cloudy solutions, indicating phase separation. It is likely that the increase in pH of solution causes dissociation of COOH groups of Gantrez® AN, which prevents weak intermolecular –COOH⋯HO– bonds formation and reduces its thermodynamic compatibility with PVA. It should also be noted that these cloudy solutions are colloidally stable and even centrifugation at 60
000 rpm for 1 h did not result in any liquid–liquid separation. Perhaps, a phase separation in PVA–Gantrez® AN mixtures in the presence of NaOH may affect the formation and morphology of pores; however, more detailed studies of this phenomenon are outside of the scope of this work.
Sol–gel fraction analysis
Formation of hydrogels from ready-made polymers often results in materials containing large quantities of loosely bound, non-cross-linked macromolecules. To quantify the efficiency of cross-linking and the content of loosely bound macromolecules a sol–gel analysis is usually performed.40 This involves the extraction of a soluble fraction and gravimetric determination of soluble/insoluble fractions. In fact, gel content significantly influences the mechanical properties and the swelling behaviour of these materials.12 Gel and sol fractions of the cryogels produced were measured by gravimetric analysis of freeze–dried samples previously immersed in deionized water and their soluble residues (Table 1). Control samples directly freeze–dried after production were used to establish the average total weight of dry gel.
Table 1 Gel and sol fractions (%) for PVA–Gantrez® AN cryogels. Data is shown as mean ± standard deviation. Statistical significance (p < 0.05) was calculated for all data (a–c). Letters after each value indicate which other composition the sample is significantly different from
NaOH added to polymer mixture |
Gel fraction (%) |
Sol fraction (%) |
0.4a% |
21 ± 1b,c |
79 ± 1 |
0.6b% |
40 ± 3 |
60 ± 14 |
0.8c% |
44 ± 4 |
56 ± 5 |
A significant increase in gel fraction of the materials is observed when the percentage of NaOH added to the liquid mixture of the two polymers is raised from 0.4% to 0.6% w/v. Higher concentrations of NaOH during the synthesis of cryogels are likely to lead to higher degrees of PVA –OH⋯HO– inter- and intra-chain hydrogen bonding, resulting in more cross-linked cryogels with a lower soluble fraction, and ultimately in mechanically stronger gel.12 In fact at higher pH values, when the –COOH groups of Gantrez® AN are ionized, the formation of weak hydrogen bonding between the two polymers chains (–COOH⋯HO–) is prevented and OH– groups in PVA macromolecules will have better opportunities to form –OH⋯HO– bonds.37 As a result, the sol fraction is more likely to consist of hydrolysed Gantrez® AN, and the gel is enriched with PVA.
Swelling studies
The swelling behaviour of the cryogels in deionized water and in an ion-containing solution (simulating the wound fluid) were monitored for 8 days (Fig. 2a and b). In fact, moist dressings, such as hydrocolloids are usually left on the wound site from 3 up to a maximum of 7 days.41 Due to the high degree of physical cross-linking achieved using the freeze–thawing technique, their swelling ratio (SR) values are substantially lower than reported for chemically-crosslinked autoclaved hydrogels made from the same combination of polymers.42 In fact, these hydrogels can absorb more than 40 g of deionized water per 1 g of initial gel within 48 h. However, the water uptake of the cryogels is reduced in the ionic solution for the presence of mobile ions in the medium. This reduction in the cryogels swelling is related to their polyelectrolyte nature and the charge screening effects in the presence of inorganic ions.43,44
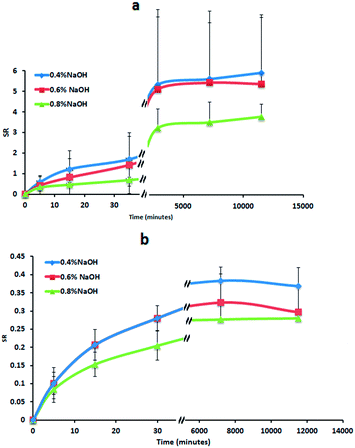 |
| Fig. 2 Swelling kinetics of PVA–Gantrez® AN cryogels in water (a) and simulated wound fluid (b). | |
The swelling ratios in both media are not affected by the amount of NaOH added to the liquid mixture of the two polymers to produce the cryogels (no statistical difference was found at any time point comparing all the samples). The samples slowly swell during the first 48 h, without the initial fast swelling exhibited for instance by the cryogels synthesised by Ahuja et al.45 from the combination of PVA and carboxymethyl tamarind kernel polysaccharide aqueous solutions. They tend to reach the equilibrium after approximately 4 days, similarly to what reported in the literature for example for cryogels prepared from mixtures of PVA with silk.46 In simulated wound fluid the swelling is decreased by the presence of Cl− which act as kosmotropes (defined as ions able to cause changes in the structure of bound and free water present in polymeric solutions), destabilizing the hydrogen-bonding hydration of PVA and Gantrez® AN through ionic hydration.47–49 The swelling of cryogels is also reduced by Ca2+ cations, which interacting with negatively charged oxygens of –OH groups and –COO− groups, form complexes increasing the cross-linking density. At the end of the experiment (after 8 days) the cryogels seem to start degrading and losing weight in the ion-containing solution, whereas in water they continue to absorb small volumes of medium.
Mechanical properties of cryogels
The mechanical properties of the PVA–Gantrez® AN cryogels produced were tested using a texture analyzer in the ‘film stretching’ mode and compared with those of PVA-only cryogels synthesised using the same cryotropic gelation method (Table 2). Some exemplary strength–strain curves are presented in Fig. S2 (ESI).†
Table 2 Mechanical properties of PVA–Gantrez® AN and PVA-only cryogel samples. PVA–Gantrez® AN cryogels (a), (b) and (c) were produced adding 0.4, 0.6 and 0.8% NaOH to the polymers mixture before freezing. Data is shown as mean ± standard deviation. Statistical significance (p < 0.05) was calculated for the data related to tensile stress at break, maximum strength, elongation to break and Young's modulus of the samples (a–d). Letters after each value indicate which other composition the sample is significantly different from
Cryogels |
Tensile stress at break (N mm−2) |
Maximum strength (N mm−1) |
Elongation to break (%) |
Young's modulus (N mm−2) |
PVA–Gant a |
(2.3 ± 1.0) × 10−4 d |
0.14 ± 0.04 |
158 ± 7c,d |
(4.7 ± 3.2) × 10−5 d |
PVA–Gant b |
(1.2 ± 0.7) × 10−4 d |
0.24 ± 0.04 |
190 ± 2d |
(8.3 ± 1.2) × 10−5 d |
PVA–Gant c |
(3.8 ± 1.0) × 10−5 d |
0.29 ± 0.04 |
218 ± 13d |
(7.6 ± 3.3) × 10−5 d |
PVA only d |
(3.4 ± 0.4) × 10−2 a,b,c |
0.53 ± 0.31 |
304 ± 21a,b,c |
(6.7 ± 0) × 10−4 a,b,c |
The presence of Gantrez® AN in the polymer mixture leads to the formation of more flexible materials compared to PVA-only cryogels. PVA macromolecules have a stronger tendency to form inter-chain hydrogen bonds compared to Gantrez® AN resulting in denser cross-linking, higher PVA crystallization and stiffer gels in case of pure PVA system. The addition of NaOH does not significantly influence the flexibility of the cryogels produced. Only the elongation to break, which is the deformation or strain that the material undergoes at fracture, increases with NaOH addition to the reaction mixture from 0.4% to 0.8% (Table 1). Note that strict comparison between the mechanical properties of PVA–Gantrez® AN cryogels prepared in the presence of NaOH and the properties of PVA only cryogel should be conducted when PVA only sample was also prepared with addition of NaOH. This was not done in the present work as PVA is not able to neutralize NaOH and would result in basic and biologically-incompatible samples.
Adhesion to skin
The adhesion to skin is an important aspect when considering new biomaterials as wound dressing candidates. During the adhesion tests, PVA–Gantrez® AN cryogel samples were applied to sections of porcine skin and then detached. The force of detachment, work of adhesion and cohesiveness values for the cryogels tested were collected and evaluated (Fig. 3).
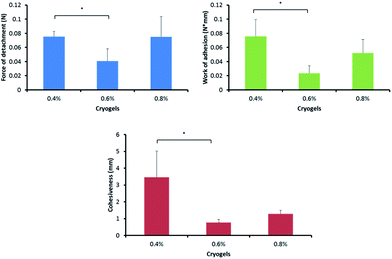 |
| Fig. 3 Force of detachment, work of adhesion and cohesiveness data recorded for PVA–Gantrez® AN cryogels during their application to porcine skin and detachment using a T.A. Asterisk (*) indicates significant statistical difference (p < 0.05). | |
The cryogels become less adhesive when the percentage of NaOH added for cryogels formation is increased from 0.4% to 0.6%. The effect of NaOH on the adhesiveness of the cryogels to skin may be related to several factors. First, the increase in the pH disrupts the hydrogen bonding between –COOH groups of Gantrez® AN and –OH groups of PVA, promoting the formation of inter-chain –OH⋯HO– hydrogen bonds. This leads to greater physical cross-linking and fewer loosely bound macromolecules present in the cryogel. This could reduce the contribution of their diffusion into the crevices of the skin lowering the adhesion. On the contrary, according to the adsorption theory, two materials can adhere to each other due to the formation of non-covalent bonds, such as hydrogen bonds, between the two surfaces in contact.50 The cryogels formed in the presence of greater quantities of NaOH will have more carboxylic groups ionized, making them less able to form hydrogen bonds with –OH groups present on the skin surface.
Antimicrobial activity
A clear S. aureus growth inhibition zone was observed around the PVA–Gantrez® AN cryogel samples that had been placed on the agar plates inoculated with the bacteria (Fig. 4). The antimicrobial activity is higher when the bacteria concentration is lower (104 CFU mL−1), as expected, and comparable to the control containing silver and other silver loaded PVA-based cryogels reported in the literature.51
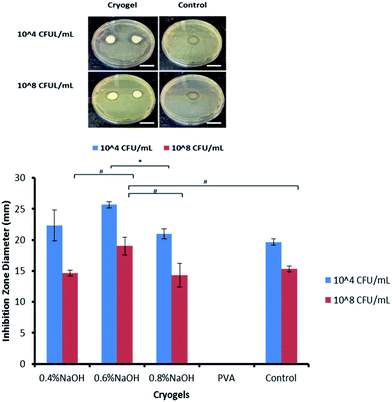 |
| Fig. 4 Antimicrobial activity of PVA–Gantrez® AN cryogels evaluated using a disk diffusion method performed with S. aureus. PVA-only cryogels and Aquacel® Ag were used as a negative and positive controls, respectively. Asterisk (*) and hash mark (#) indicate significant statistical difference (p < 0.05). | |
No bacteria growth inhibition was found for the PVA-only cryogels, whose presence did not affect S. aureus proliferation at all. The results obtained for the cryogels containing Gantrez® AN can be explained by the presence of this polymer, which has shown an intrinsic antimicrobial activity,26 as well as with the low pH (from 4.3 to 5.2) of the materials produced. A leaching of Gantrez® AN or H+ ions from the cryogels could be the reason for their antimicrobial properties. Mild acidic pH (∼5.5) prevents S. aureus proliferation, interfering with its gene expression and creating a hostile environment for its growth.52
In vitro cytotoxicity studies
The biocompatibility of the materials produced was assessed in vitro performing an MTT assay using human dermal fibroblasts (Fig. 5). This well-known colorimetric test allows a rapid and clear cytotoxicity evaluation of drugs and biomaterials.53 The positively charged MTT reagent is promptly internalised by viable cells and converted into a coloured product (called ‘formazan’), which exhibits a maximum absorbance at 570 nm.24 PVA–Gantrez® AN cryogel extracts collected in sterile conditions were used for the test.
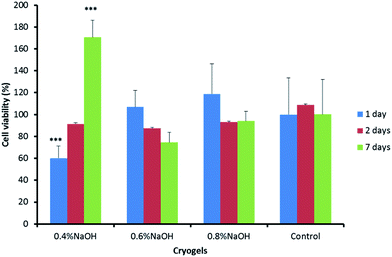 |
| Fig. 5 Cell viability assessment by MTT assay at 1, 2 and 7 days post-seeding for PVA–Gantrez® AN cryogels and control (AQUACEL® Ag) extracts. Asterisk (*) indicates significant statistical difference (p < 0.05). | |
The cryogel compositions, with the only exception of the samples where 0.4% of NaOH was initially added for the 1 day extract, showed good cell viability (above 70%). Thus, it is possible to confirm the biocompatibility of the materials produced. No difference was found in the viability of cell cultures placed in contact with extracts collected from the cryogel samples (and control) after 2 days. The gel formed with the addition of 0.4% of NaOH to the PVA–Gantrez® AN mixture, which showed the highest cytotoxicity for the 1 day gel extracts, exhibited the highest viability for the 7 days extracts. The pH plays a very important role in maintaining a favourable environment for the cells to grow and proliferate. In fact, the samples formed in the presence of 0.6% and 0.8% NaOH having the highest pH (∼5), showed more desirable cell viability for the extracts collected after 1 day, with no difference compared to the control.
The sterility is necessary for most wound management products. The PVA–Gantrez® AN cryogels developed in this work were sterilized using gamma irradiation, which is commonly employed for the sterilisation of medical devices.25 The swelling of the gamma-sterilized samples in deionized water is slightly reduced due to additional cross-linking of the polymers induced by the gamma irradiation (Fig. S3, ESI†). PVA and its blends with other polymers are known to undergo cross-linking reactions upon their radiation treatment.39,41,54 However, the mechanical properties of PVA–Gantrez® AN hydrogels do not seem to be affected by the radiation treatment (Table S1, ESI,† no statistical difference was found among the data analysed).
Conclusions
Highly porous PVA–Gantrez® AN cryogels were successfully prepared using a freeze–thawing method. The cryogels presented in this work were produced from mixtures of aqueous solutions of PVA and Gantrez® AN, after only one freeze–thawing cycle with freezing at −18 °C and thawing at ∼25 °C, with the addition of small quantities NaOH to adjust their final pH. The materials described in this study have shown good swelling capacity, as well as excellent mechanical properties and level of adhesion to skin. Their soft consistency and high moisture content could provide a comfortable feeling on traumatized and inflamed skin, which may improve patient compliance with the treatment. An intrinsic antimicrobial activity was confirmed due to the presence of Gantrez® AN, and the low pH, which is a significant advantage of these cryogels over PVA-only gels and many commercial products that require the addition of antimicrobial agents, such as iodine or silver.42,43 The cryogels also demonstrated very good biocompatibility when tested using human dermal fibroblasts which make them promising materials for wound dressings.
Acknowledgements
The authors would like to acknowledge the University of Reading and ConvaTec Ltd for funding the doctoral studies of EC and the Chemical Analysis Facility (University of Reading) for access to SEM.
References
- N. A. Peppas, P. Bures, W. Leobandung and H. Ichikawa, Eur. J. Pharm. Biopharm., 2000, 50, 27–46 CrossRef CAS PubMed.
- V. I. Lozinsky, I. Y. Galaev, F. M. Plieva, I. N. Savina, H. Jungvid and B. Mattiasson, Trends Biotechnol., 2003, 21, 445–451 CrossRef CAS PubMed.
- V. I. Lozinsky and O. Okay, Basic Principles of Cryotropic Gelation–Polymeric Cryogels, ed. O. Okay, Adv. Polym. Sci., Springer International Publishing, Switzerland, 2014, vol. 263, pp. 49–101 Search PubMed.
- C. M. Hassan and N. A. Peppas, J. Appl. Polym. Sci., 2000, 76, 2075–2079 CrossRef CAS.
- S. K. H. Gulrez, S. Al-Assaf and G. O. Phillips, Hydrogels: Methods of Preparation, Characterisation and Applications, Progress in Molecular and Environmental Bioengineering – From Analysis and Modeling to Technology Applications, ed. A. Carpi, InTech, 2011, pp. 117–150 Search PubMed.
- V. I. Lozinsky, A Brief History of Polymeric Cryogels–Polymeric Cryogels, ed. O. Okay, Adv. Polym. Sci., Springer International Publishing, Switzerland, 2014, vol. 263, pp. 1–48 Search PubMed.
- A. Kumar, R. Mishra, Y. Reinwald and S. Bhat, Mater. Today, 2010, 13, 42–44 CrossRef CAS.
- N. A. Peppas and S. R. Stauffer, J. Controlled Release, 1991, 16, 305–310 CrossRef CAS.
- A. S. Hickey and N. A. Peppas, J. Membr. Sci., 1995, 107, 229–237 CrossRef CAS.
- V. Pazos, R. Mongrain and J. C. Tardif, J. Mech. Behav. Biomed. Mater., 2009, 2, 542–549 CrossRef CAS PubMed.
- V. I. Lozinsky, A. L. Zubov and E. F. Titova, Enzyme Microb. Technol., 1997, 20, 182–190 CrossRef CAS.
- E.-R. Kenawy, E. A. Kamoun, M. S. Mohy Eldin and M. A. El-Meligy, Arabian J. Chem., 2014, 7, 372–380 CrossRef CAS.
- E. A. Kamoun, X. Chen, M. S. Mohy Eldin and E.-R. S. Kenawy, Arabian J. Chem., 2014, 8, 1–14 CrossRef.
- E. Caló and V. V. Khutoryanskiy, Eur. Polym. J., 2014, 65, 252–267 CrossRef.
- C.-D. Varganici, O. M. Paduraru, L. Rosu, D. Rosu and B. C. Simionescu, J. Anal. Appl. Pyrolysis, 2013, 104, 77–83 CrossRef CAS.
- X. Qi, X. Hu, W. Wei, H. Yu, J. Li, J. Zhang and W. Dong, Carbohydr. Polym., 2015, 118, 60–69 CrossRef CAS PubMed.
- P. Arbós, M. Wirth, M. Arangoa, F. Gabor and J. Irache, J. Controlled Release, 2002, 83, 321–330 CrossRef.
- M. Agüeros, M. A. Campanero and J. M. lrache, J. Pharm. Biomed. Anal., 2005, 39, 495–502 CrossRef PubMed.
- V. V. Khutoryanskiy, Int. J. Pharm., 2007, 334, 15–26 CrossRef CAS PubMed.
- O. V. Khutoryanskaya, V. V. Khutoryanskiy and R. A. Pethrick, Macromol. Chem. Phys., 2005, 206, 1497–1510 CrossRef CAS.
- M. Fernandez, J. Parra, B. Vazquez, A. Lopez-Bravo and J. S. Román, Biomaterials, 2005, 26, 3311–3318 CrossRef CAS PubMed.
- J. S. Boateng, H. V Pawar and J. Tetteh, Int. J. Pharm., 2013, 441, 181–191 CrossRef CAS PubMed.
- J. J. Castellano, S. M. Shafii, F. Ko, G. Donate, T. E. Wright, R. J. Mannari, W. G. Payne, D. J. Smith and M. C. Robson, Int. Wound J., 2007, 4, 114–122 CrossRef PubMed.
- R. Scherliess, Int. J. Pharm., 2011, 411, 98–105 CrossRef CAS PubMed.
- M. Cheddadi, E. López-Cabarcos, K. Slowing, E. Barcia and A. Fernández-Carballido, Int. J. Pharm., 2011, 413, 126–133 CrossRef CAS PubMed.
- J. M. Rosiak and J. Olejniczak, Radiat. Phys. Chem., 1993, 42, 903–906 CrossRef CAS.
- O. Okay and V. I. Lozinsky, Synthesis and Structure – Property Relationships of Cryogels –Polymeric Cryogels, ed. O. Okay, Adv. Polym. Sci., Springer International Publishing, Switzerland, 2014, vol. 263, pp. 103–157 Search PubMed.
- M. Watase and K. Nishinari, Carbohydr. Polym., 1989, 11, 55–66 CrossRef CAS.
- W. Wan, A. D. Bannerman, L. Yang and H. Mak, Poly(Vinyl Alcohol) Cryogels for Biomedical Applications – Polymeric Cryogels, ed. O. Okay, Adv. Polym. Sci., Springer International Publishing, Switzerland, 2014, vol. 263, pp. 283–321 Search PubMed.
- C. Chang, A. Lue and L. Zhang, Macromol. Chem. Phys., 2008, 209, 1266–1273 CrossRef CAS.
- M. J. D. Nugent, A. Hanley, P. T. Tomkins and C. L. Higginbotham, J. Mater. Sci.: Mater. Med., 2005, 16, 1149–1158 CrossRef CAS PubMed.
- M. J. D. Nugent and C. L. Higginbotham, Eur. J. Pharm. Biopharm., 2007, 67, 377–386 CrossRef CAS PubMed.
- J. Boateng and O. Catanzano, J. Pharm. Sci., 2015, 1–28 Search PubMed.
- A. Jones and D. Vaughan, J. Orthop. Nurs., 2005, 9, S1–S11 CrossRef.
- M. Flanagan, Wound Healing and Skin Integrity: Principles and Practice, John Wiley & Sons, Oxford, UK, 2013 Search PubMed.
- R. Mishra, S. K. Goel, K. C. Gupta and A. Kumar, Tissue Eng., Part A, 2014, 20, 751–762 CAS.
- V. I. Lozinsky, L. G. Damshkaln, M. G. Ezernitskaya, Y. K. Glotova and Y. A. Antonov, Soft Matter, 2012, 8, 8493 RSC.
- E. A. Podorozhko, G. R. Ul'yabaeva, N. R. Kil'deeva, V. E. Tikhonov, Y. A. Antonov, I. L. Zhuravleva and V. I. Lozinsky, Colloid J., 2016, 78, 90–101 CrossRef CAS.
- R. Koningsveld and L. A. Kleintjens, J. Polym. Sci., Polym. Symp., 1977, 61, 221–249 CrossRef CAS.
- J. M. Rosiak, Radiat. Phys. Chem., 1995, 46, 161–168 CrossRef CAS.
- S. Baranoski and E. A. Ayello, Wound Care Essentials: Practice Principles, Lippincott Williams & Wilkins, Philadelphia, USA, 2004 Search PubMed.
- E. Caló, J. M. S. de Barros, M. Fernández-Gutiérrez, J. San Román, L. Ballamy and V. V. Khutoryanskiy, RSC Adv., 2016, 6, 55211–55219 RSC.
- G. Stell and C. G. Joslin, Biophys. J., 1986, 50, 855–859 CrossRef CAS PubMed.
- S. M. M. Quintero, R. V. Ponce, F. M. Cremona, A. L. C. Triques, A. R. d'Almeida and A. M. B. Braga, Polymer, 2010, 51, 953–958 CrossRef CAS.
- M. Ahuja, Int. J. Biol. Macromol., 2015, 72, 931–938 CrossRef PubMed.
- P. Y. Neo, P. Shi, J. C.-H. Goh and S. L. Toh, Biomed. Mater., 2014, 9, 065002 CrossRef PubMed.
- S. Patachia, C. Florea, C. Friedrich and Y. Thomann, eXPRESS Polym. Lett., 2009, 3, 320–331 CrossRef CAS.
- H. Muta, M. Miwa and M. Satoh, Polymer, 2001, 42, 6313–6316 CrossRef CAS.
- L. Xu, X. Li, M. Zhai, L. Huang, J. Peng, J. Li and G. Wei, J. Phys. Chem. B, 2007, 111, 3391–3397 CrossRef CAS PubMed.
- N. A. Peppas and J. J. Sahlin, Biomaterials, 1996, 17, 1553–1561 CrossRef CAS PubMed.
- A. Chaturvedi, A. K. Bajpai and J. Bajpai, Polym. Compos., 2015, 36, 1983–1997 CrossRef CAS.
- B. Weinrick, P. M. Dunman, F. McAleese, E. Murphy, S. J. Projan, Y. Fang and R. P. Novick, J. Bacteriol., 2004, 186, 8407–8423 CrossRef CAS PubMed.
- G. Ciapetti, E. Cenni, L. Pratelli and A. Pizzoferrato, Biomaterials, 1993, 14, 359–364 CrossRef CAS PubMed.
- Z. S. Nurkeeva, G. A. Mun, A. V. Dubolazov and V. V. Khutoryanskiy, Macromol. Biosci., 2005, 5, 424–432 CrossRef CAS PubMed.
Footnote |
† Electronic supplementary information (ESI) available: Images of polymer mixtures prepared at different NaOH concentrations, swelling and mechanical properties of cryogels sterilized using gamma-irradiation. See DOI: 10.1039/c6ra24573k |
|
This journal is © The Royal Society of Chemistry 2016 |
Click here to see how this site uses Cookies. View our privacy policy here.