DOI:
10.1039/C6RA24215D
(Paper)
RSC Adv., 2017,
7, 2953-2963
SiO2 nanoparticles cause depression and anxiety-like behavior in adult zebrafish†
Received
29th September 2016
, Accepted 26th November 2016
First published on 8th December 2016
Abstract
The extensive employment of engineered nanoparticles (NPs) makes it inevitable that the environment would be exposed to nano-materials. As a result, their biological effects on the ecosystem and living organisms are gaining attention. Here, we report that both nano-silica (SiO2-NPs) and reserpine can elicit depression-like behavior in adult zebrafish in a novel tank test. Nano-silica or reserpine induce the depressive phenotype by decreasing locomotion, inhibiting exploratory behavior, aggravating the depressive phenotype and disturbing swimming patterns. Immunohistochemistry reveals that the dampened locomotion induced by nano-silica and reserpine is associated with the reduced expression of tyrosine hydroxylase. Inhibited exploratory behavior, aggravated depressive phenotypes, and disturbed spatiotemporal swimming path patterns are related to the increased expression of serotonin. These findings imply that the similar behavioral and depression-inducing effect of nano-silica and reserpine may share a common physiological mechanism. In summary, our results suggest that exposure to silica nanoparticles in the nervous system is a possible depression-inducing factor.
Introduction
With the development of nanotechnology, engineered nano-materials such as nano-silica (SiO2-NPs) have been extensively applied in various fields of daily life. Nano-silica is used for biomedical purposes, for example in targeted drug delivery and controlled release,1 bio-imaging,2 and cancer treatment.3 In agriculture and manufacturing, nano-silica also plays an indispensable role in paints and bactericidal agents. Among nanotech-based consumer products, nano-silica is in the top five of nano-materials used.4 Thus, with the expansive application of silica nanomaterials, terrestrial or aquatic organisms and humans are more likely to be exposed. The potential adverse effects of nano-silica used daily on the ecological environment and on human health is an urgent problem which needs to be addressed.5 Fortunately, a multitude of research techniques are relevant for deciphering the side effects of nano-silica.6 Substantial work has already paid attention to evaluate the toxicity in vitro.7–12 Recently, a host of researchers have assessed the toxicity in vivo. Xiang, L. et al. applied AB zebrafish (Danio rerio) as an animal model to find that nanosilica acted on the retina and dopaminergic (DA) neurons to change colour preference and to cause potential Parkinson’s disease-like behavior. Furthermore, Wu, J. et al. used SD rats to propose that SiO2-NPs may enhance the potential risk of neurodegenerative disease by exerting a negative impact on the striatum and DA neurons. Overall, these studies suggest that nano-silica has a hazardous effect on the nervous system and stress the importance of fully investigating the neuro-toxicity of nano-silica.
Because of their genetic and physiological homology with humans,13,14 a large and variable behavioral repertoire,15 and high throughput experimental manipulation,16 zebrafish have emerged as a promising efficient animal model for studying various brain disorders.17–19 In addition, zebrafish have become the ideal candidate for studying CNS disorders in light of the conserved mechanism of neuropsychiatric dysfunction with humans.20,21 Reserpine, clinically used as an early anxiolytic-like antipsychotic, exerts an obvious effect in suppressing bipolar disorder (BP) symptoms. But it can elicit major depressive disorder (MDD) by depleting dopamine.22 Currently, reserpine exposure is the widely accepted pharmacological manipulation method which is reported to induce depression-like phenotypes in zebrafish.23 Zebrafish exposed to reserpine demonstrated long-term depressive symptoms, such as elevated baseline whole-body cortisol, social withdrawal and locomotion retardation.24
This research aims to discover the potential depressive effects caused by chronic exposure to nano-silica by behavioral analysis and immunohistochemistry. Reserpine is used in this study to induce a depression model in zebrafish. Comparative analysis of nano-silica vs. reserpine is made to characterize the potent depression-causing effect of nano-silica. Behavioral tests exhibit a similarity between nano-silica and reserpine in eliciting depression-like behavior such as decreasing locomotion, inhibiting exploratory behavior, aggravating the depressive phenotype and disturbing swimming patterns. Furthermore, immunohistochemistry reveals the comparability of nano-silica with reserpine in decreasing the expression of tyrosine hydroxylase but increasing the expression of serotonin. Thus, these results demonstrate that in causing depression, nano-silica may possibly share a common behavioral and physiological mechanism with reserpine. In addition, further investigations should be carried out to decipher specific common genetic and molecular mechanisms.
Methods and materials
Experiment ethics and animal housing
Zebrafish (12 months old) were maintained in an environmentally controlled room with a 14/10 h light/dark cycle (07:00 AM to 09:00 PM) and a temperature at 25 °C in the State Key Laboratory of Medicinal Chemical Biology, Nankai University. Adult zebrafish (Danio rerio) of the wild type (AB strain) were housed in a recirculating aquatic system at 28.5 °C according to the typical zebrafish housing standards.25 Circulating water in the aquarium (Shanghai Haisheng Biotech Co. LTD) was filtered by reverse osmosis (pH 7.0–7.5). To maintain the conductivity of standard system water to 450–550 nS cm−1 and the pH to 7.0–7.4, Instant Ocean® salt and sodium bicarbonate were added. Fish were fed twice a day with a mixture of frozen brine shrimp (Germurunze bio-product co. LTD) and flakes for aquarium fish (Shanghai min-ang electronic business co. LTD). All the experimental protocols concerning zebrafish were approved by the Committee for Animal Experimentation of the College of Life Science at Nankai University (no. 2008). All the experimental procedures were performed in accordance with the NIH Guide for the Care and Use of Laboratory Animals (no. 8023, revised in 1996).
Experimental apparatus
The novel tank, used to assess anxiety and depression behavior of zebrafish, was a 5 L rectangular box (23 cm length × 15 cm width × 15 cm depth), which was made from transparent Plexiglass. The tank was maximally filled with water and divided into two equal virtual horizontal portions, by a line marking the outside walls. The area above this mid-line represented the ‘upper half (top)’ of the novel tank, while the region below represented the ‘lower half (bottom)’ of the novel tank.26–28 The novel tank was placed over a light source, an LED array, with an acrylic diffuser located above (Fig. 1a). The light source was composed of white light arrays and a transparent platform. The light intensity of the white light was 500 lux. Acrylic diffusers here were used to homogenize the illumination intensity and to enhance the contrast between the three zebrafish individuals and chamber background during video tracking. Two CCD cameras (MV-VS078FM, Microvision, 10 frames per s), were fixed to obtain the top (dorsal) view and side (lateral) view of the moving zebrafish (Fig. 1b). A daylight lamp (500 lux) was mounted above the camera to offer photo-stimulation to trigger courtship behavior. Experiments were conducted in a relatively sound-proof room to minimize the effect of noise. A big black cloak covered all the experimental apparatus to eliminate environmental interference. All apparatus rested on a level, stable surface.
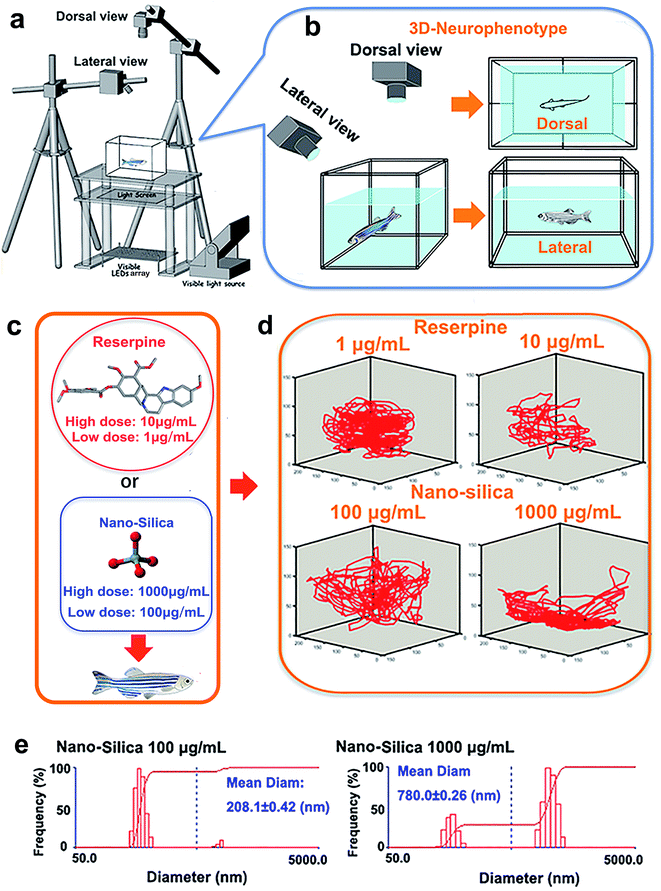 |
| Fig. 1 Experimental paradigm. (a) Experimental setup of three-dimensional behavioral neuro-phenotyping of adult zebrafish in the novel tank test. (b) Two cameras from a dorsal view and lateral view recorded the behavior of zebrafish in the novel tank. (c) Zebrafish were chronically (for 10 days) exposed to 15 nm SiO2-NPs or reserpine solutions. (d) The depression-causing or anxiogenic effect of nanosilica (15 nm) vs. reserpine was compared via behavioral analysis. 3D reconstruction of swimming trajectories demonstrated the distinct swimming patterns of zebrafish exposed to nanosilica vs. reserpine. (e) Characterisation of nanosilica (100 μg mL−1 and 1000 μg mL−1) dispersed in a standard water system using dynamic light scattering (DLS). | |
Pharmacological manipulation
Reserpine (purity ≥ 98.0%) used in this study was purchased from Shanghai Macklin Biomedical Co., Ltd, and SiO2-NPs (15 nm) were purchased from Xuan Cheng Jing Rui New Material Co., Ltd. China. The rationale for the concentration of reserpine was based on previous research concerning the effective doses of reserpine on behavior of zebrafish.23 In this study, mild doses: 1 μg mL−1 (low concentration) and 10 μg mL−1 (high concentration) were chosen (Fig. 1c). Also, the chosen concentration of SiO2 NPs (15 nm) was according to the dose that elicited a behavioral change.6 Subsequently, 100 μg mL−1 (low concentration) and 1000 μg mL−1 (high concentration) were chosen (Fig. 1c). Before being suspended in a 3 L tank, the experimental doses of reserpine (1 μg mL−1, 10 μg mL−1) or SiO2-NPs (100 μg mL−1, 1000 μg mL−1) were prepared by weighing and adding dry powder to the system’s water. To facilitate the suspension of the particles, the solutions were sonicated for 30 min. The diameters and distributions of the 15 nm nano-silica in the system water were then determined using dynamic light scattering (DLS) (Fig. 1e).
A total of 80 AB strain adult zebrafish (12 months old, male
:
female = 1
:
1) that were experimentally naïve were used in this study. All fish were housed in groups of 2 fish per 4 L tank (filled with filtered facility water maintained at 27 °C) on a 14:10 h cycle. Fish were fed with live brine shrimp twice per day. The fish were separated into five groups according to the pharmacological manipulations. The five groups were exposed to tank water (as a control, n = 24), reserpine of low concentration (1 μg mL−1, n = 14), reserpine of high concentration (100 μg mL−1, n = 14), 15 nm nano-silica of low concentration (100 μg mL−1, n = 14), 15 nm nano-silica of high concentration (1000 μg mL−1, n = 14). In order to examine the long term effect of reserpine or 15 nm SiO2-NPs, the exposure duration of the five groups was 10 days. Water in each 4 L tank containing standard tank water, reserpine or nano-silica was refreshed twice a day after feeding with brine shrimp. All the experimental subjects in a 4 L tank were offered the same illumination, temperature and dissolved oxygen conditions identical to those in a standard aquarium. The zebrafish were euthanized with 500 mg L−1 tricaine (Sigma-Aldrich, St. Louis, MO), following behavioral testing. Then the animals were immediately dissected on ice for further analysis. All surgery was performed under cold anesthesia, and all efforts were made to minimize suffering.
Behavioral testing
All fish were given at least 10 days to acclimate to the laboratory environment. After 10 days of chronic reserpine or SiO2-NPs treatment, fish were placed into a 3 L beaker with tank water for 2 h. Before the behavioral test, fish were given 1 h to acclimate to the environment. Behavioral testing was performed between 11.00 am and 15.00 pm with tanks filled with water at a temperature from 25 °C to 27 °C. Zebrafish behavior was recorded by two cameras that were fixed vertically and horizontally. Zebrafish behavior was evaluated by recording and analyzing the following behavioral endpoints: total distance traveled (m), average velocity (m s−1), turn angle (°), angular velocity (° s−1), meandering (° m−1), average entry duration in the top (s), distance traveled in the top (m), time spent in the top (s), latency to enter the top (s), number of entries to the top, time spent ratio of top
:
bottom, distance travel ratio of top
:
bottom, entries ratio of top
:
bottom, tail drop angle (°), freezing bouts (frequency), freezing duration (s).
Behavioral parameters
The behavioral parameters briefly referred to the definition by Allan V. Kalueff, in which all the behavioral parameters in the novel tank test were specified.29,30 The specific definitions of behavioral parameters that describes depression and anxiety profiles are demonstrated as follows:30
(1) Total distance traveled (m) was defined as the total distance in the novel tank.30
(2) Average velocity (cm s−1) was defined as the direction and magnitude of zebrafish speed in the novel tank.30
(3) Turn angle (°) was defined as the total turning angle of zebrafish in the novel tank.30
(4) Angular velocity (° s−1) was defined as the direction and magnitude of zebrafish angular speed in the novel tank.30
(5) Meandering (° s−1) was defined as the degree of turning vs. travel distance.30
(6) Average entry duration in the top (s) was defined as the amount of time spent at the top of the novel tank during each crossing.30
(7) Distance traveled in the top (m) was defined as the total distance moved in the defined top part in the novel tank.30
(8) Time spent in the top (s) was defined as the total time spent in the top part of the novel tank.30
(9) Latency to enter the top (s) was the amount of time to first cross from the bottom part to the top of the novel tank.30
(10) Number of entries to the top was defined as the number of crosses from the bottom part to the top of the novel tank.30
(11) Time spent ratio of top
:
bottom was the ratio of the time spent on top over bottom.30
(12) Distance traveled ratio of top
:
bottom was defined as the ratio of the total distance moved in the top part versus the bottom.30
(13) Entries ratio of top
:
bottom was defined as the number of crosses from the bottom part to the top of the novel tank.30
(14) Freezing bouts (frequency) was defined as the total number of instances of immobility (>1 s) during the 5 minutes test in the novel tank.30
(15) Freezing duration (s) was defined as the duration of all freezing bouts in the novel tank.30
(16) Tail drop angle (°) was defined as the angle of the body against the horizontal plane (Fig. 5a).15,23,30
Immunohistochemistry
The brains were harvested after the silica and RSP treatment for 10 days, and immediately fixed in 4% paraformaldehyde, equilibrated in 30% sucrose/PBS overnight and embedded in OCT. Sections of 10 μm thickness were mounted on gelatin-coated slides and air dried at 37 °C for at least 2 h. The tissue sections were rehydrated with PBS, blocked with 20% NGS and 2% BSA in 0.3% PBS/Triton X-100 (PBST) for 1 h and incubated with primary antibodies overnight at 4 °C. The following primary antibodies and concentrations were used: mouse monoclonal antibody 5-HT (1
:
4000, Sigma) for labelling cones and mouse monoclonal anti-tyrosine hydroxylase (1
:
400, Millipore, Billerica, MA) for labelling DA cells. The interpretation of the neuroanatomy follows the adult zebrafish brain atlas. Immunoreactions were detected using Cy3-labelled goat anti-mouse IgG diluted to 1
:
400 (Millipore). The sections were counterstained in a 1
:
1000 dilution of 4′,6-diamidino-2-phenylindole (DAPI) (Sigma) to label the nuclei. The slides were viewed with an Olympus BX51 light microscope (Olympus, Tokyo, Japan). The images were captured by an Olympus CCD DP71 (Olympus) and processed using Adobe Photoshop CS (Adobe Systems, San Jose, CA).
Western blotting
The levels of tyrosine hydroxylase in the brain were measured by western blot analysis. After the treatment, the brains were harvested and immediately lysed in a tissue protein extraction reagent (CWBIO, Beijing, China) with PMSF (Sigma-Aldrich). A BCA Protein Assay Kit (CWBIO) was used to quantify the protein concentrations. Then the proteins were subjected to SDS-PAGE and transferred onto a nitrocellulose membrane blocked with 5% non-fat dry milk in Tris-buffered saline with 0.05% Tween-20. The membrane was incubated with the following primary antibodies: mouse anti-TH (1
:
1000; Millipore) and rabbit anti-actin (1
:
1000; CWBIO). After being washed with Tris-buffered saline containing 0.05% Tween-20, the membrane was incubated with an anti-mouse or an anti-rabbit peroxidase-conjugated secondary antibody (1
:
3000; CWBIO). Then the membrane was washed with Tris-buffered saline with 0.05% Tween-20, and Super Signal West Pico chemiluminescent substrate (Thermo Scientific) was used for detection.
Data analysis
Histograms were presented as mean + standard error of the mean (S.E.M.). All the plots were generated by GraphPad Prism 6 for Mac (GraphPad Software, Inc.). P < 0.05 was considered to indicate a significant difference. One-way ANOVAs were performed to compare the difference among variances, followed by post hoc Turkey HSD tests using SPSS Statistics 22 for Mac (IBM). Cluster 3.0 (Stanford University, USA) was used to perform hierarchical clustering ordered by Euclidean distances31 to link the nano-silica or reserpine treatment to the alteration of all behaviors. Java Tree View (University of Glasgow, UK) was utilized to visualize the clustering result. In the clustergram, each cell represented the average relative value and the standard deviation with blue lower than the control, red higher than the control, and black invariable. Analysis of the droopy tail angle was performed using MATLAB R2012b (MathWorks) with the CircStat toolbox.32 Circular significance was conducted using the Watson–William test.33 3D-Reconstruction and 3D spatiotemporal reconstruction of the swim path were performed in accordance with the method described before.6,34,35
Result and discussion
Nano-silica or reserpine dampened the locomotion of zebrafish
The locomotion behavior of zebrafish, which was measured by the total distance traveled, averaged velocity, turn angle and angular velocity, has been suppressed by nano-silica or reserpine compared with the control. Both nano-silica and reserpine significantly decrease the total distance traveled (Fig. 2b) (F4,70 = 4.452, P = 0.0026). In addition, the averaged velocity (Fig. 2c) has also been suppressed by treatment with both reserpine and nano-silica (F4,70 = 4.475, P = 0.0026). Besides, both the reserpine and silica treated manipulations affected the swimming flexibility of adult zebrafish by decreasing the turn angle (Fig. 2d) (F4,70 = 5.821, P = 0.0004). The value of angular velocity in both reserpine and nano-silica treated fish also declined dramatically (Fig. 2e) (F4,70 = 5.742, P = 0.0005). Moreover the erratic movements measured by meandering in the novel tank test (Fig. 2f) revealed a significant reduction in meandering (F4,70 = 6.345, P = 0.0002). General results have indicated that nano-silica suppressed the locomotion profiles of zebrafish. Locomotion behavior reflects the general motor aspects of swimming zebrafish and may be decreased depending on the anxiogenic effect of certain chemicals.30 Results from the nano-silica data suggested a potential locomotion-dampened effect of nano-silica, which is comparable with that of reserpine.23
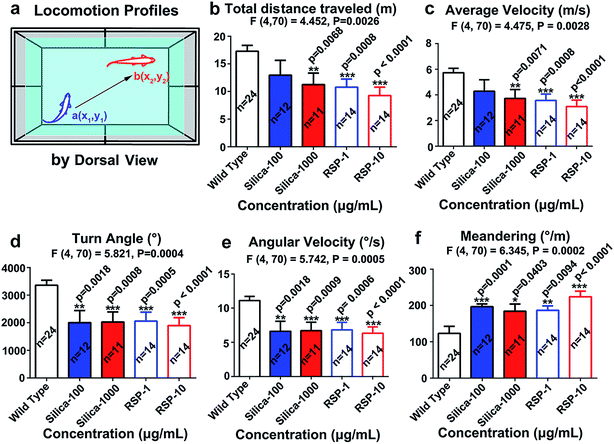 |
| Fig. 2 Locomotion profiles of zebrafish exposed to nano-silica or reserpine in the novel tank test. (a) Diagram of locomotion behavior recorded by a camera with a dorsal view. A histogram revealed that both reserpine and nano-silica dampened locomotion of adult zebrafish by decreasing the (b) total distance traveled, (c) averaged velocity, (d) turn angle and (e) angular velocity. Reserpine and nano-silica caused the erratic movements measured by (f) meandering in the novel tank test. The data are expressed as the mean + S.E.M. and were analyzed by one-way ANOVA followed by the Turkey post hoc test. Significance was defined as *p < 0.05, **p < 0.01, and ***p < 0.001. | |
Both nano-silica and reserpine treatment were associated with inhibited exploratory behavior and disturbed spatiotemporal swimming patterns of zebrafish
The average entry duration in the top (Fig. 3b) (F4,70 = 3.080, P = 0.0214), distance traveled in the top (Fig. 3c) (F4,70 = 4.596, P = 0.0024), time spent in the top (Fig. 3d) (F4,70 = 4.635, P = 0.0022), latency to enter the top (Fig. 3e) (F4,39 = 2.236, P = 0.0427), number of entries to the top (Fig. 3f) (F4,70 = 5.293, P = 0.0009), time spent ratio of top
:
bottom (Fig. 3g) (F4,70 = 2.101, P = 0.0498) and distance traveled in the top
:
bottom (Fig. 3h) (F4,70 = 1.783, P = 0.1420) are all shown in Fig. 3. However, there is a notable trend in that the ratios of the distance traveled in the top
:
bottom and the number of entries in the top
:
bottom (Fig. 3i) (F4,70 = 4.464, P = 0.0020) are all significantly decreased by nano-silica. It has been reported that a high anxiety level in zebrafish is directly related to a decreased value of the exploratory behavioral parameters mentioned above. Therefore, the behavioral result demonstrated the anxiogenic effect of silica.
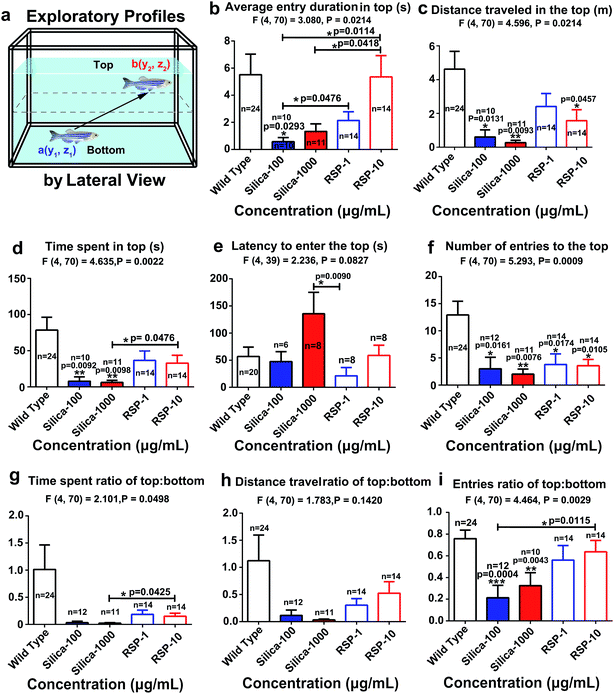 |
| Fig. 3 Exploratory profiles of zebrafish exposed to nano-silica or reserpine in the novel tank test. (a) Diagram of the exploratory behaviors recorded by camera with a lateral view. The histograms demonstrated that both reserpine and nano-silica inhibited exploratory behaviors of adult zebrafish by decreasing the (b) average entry duration to the top, (c) distance traveled in the top, (d) time spent in the top, (e) latency to enter the top, (f) number of entries to the top, (g) time spent ratio of top : bottom, (h) distance traveled of top : bottom and (i) entries ratio of top : bottom. The data are expressed as the mean + S.E.M. and were analyzed by one-way ANOVA followed by the Turkey post hoc test. Significance was defined as *p < 0.05, **p < 0.01, and ***p < 0.001. | |
The effect of different concentrations of nano-silica or reserpine on the spatiotemporal swimming patterns are further investigated by three-dimensional swimming path reconstruction and spatiotemporal swimming trajectory reconstruction (Fig. 4a). Wild type zebrafish demonstrate normal locomotion patterns: the spatial scope of wild type zebrafish is wide and trajectories of wild-type zebrafish are intensive. However, both nano-silica and reserpine disturb their swimming path patterns, resulting in sparse trajectories and decreased locomotive activity. Furthermore, nano-silica and reserpine inhibit the exploratory behavior of zebrafish in the novel tank, decreasing their swimming path in the upper part. To explore the precise effects of silica on instantaneous locomotive activities at each location in the swimming path over the course of 5 min, locomotive activities were colour-coded (Fig. 4a–c); the legend’s colour scales represent the proportional spectrum across the minimum/maximum ranges of the locomotive parameter values (ESI Fig. S1–S5†). Interestingly, 100 μg mL−1 nano-silica and 1 μg mL−1 reserpine exhibited similar changes in the swimming path patterns and locomotive activities of the zebrafish.
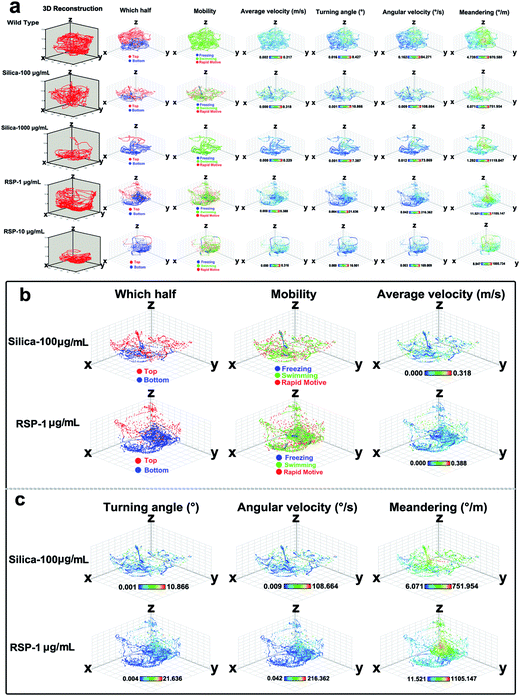 |
| Fig. 4 Three-dimensional-spatial and 3D-spatiotemporal reconstructions of adult zebrafish swim trajectories exposed to nano-silica or reserpine. (a) “3D reconstruction” indicates the spatial swimming path changes induced by nano-silica or reserpine in the whole novel tank. “Which half” represents the spatiotemporal swimming path in red indicating trajectories in the top part and in blue indicating trajectories in the bottom part. The instantaneous locomotion at every point in the trajectories was color-coded according to the value; the legend colour scales represent the proportional spectrum across the minimum/maximum ranges of the locomotive parameter values. Similar effect of 100 μg mL−1 nano-silica and 1 μg mL−1 reserpine in altering the spatiotemporal swimming path (b) and (c) the instantaneous locomotion on trajectories of zebrafish in the novel tank. | |
Both reserpine and nano-silica altered the expression of serotonin and decreased the level of tyrosine hydroxylase (TH)
The increased anxious behavior and decreased locomotive activity were detected in the reserpine and nano-silica treated groups. It is well known that serotonergic systems are involved in the modulation of fear and/or anxiety.36 The decreased locomotive activity is a characteristic of Parkinson’s disease in zebrafish.37 Thus the expression of serotonin and tyrosine hydroxylase were examined in brain tissues after 10 days treatment.
As shown in Fig. 6a, reserpine can promote the level of serotonin in white matter, but the number of the neurons containing serotonin decreased. In the RSP-10 group, neurons containing serotonin were hardly detected. In either of the nano-silica groups, the serotonin disseminated in the white matter, no 5-HT neurons were found. The expression of tyrosine hydroxylase (TH) was used to evaluate the number of dopaminergic (DA) neurons. The expression of TH in the posterior tuberculum (TP), which is the substantia nigra of the zebrafish, decreased in the reserpine groups (Fig. 6b). The decrease of TH in RSP-1 is much more than in RSP-10. The expression of TH also decreased in the silicon groups, and the decrease of TH in nano-silica-100 was smaller than it was in the nano-silica-1000 group.
Depressive phenotype induced by nano-silica or reserpine
Circular statistics indicate that the droopy tail angle increased by nano-silica and reserpine treatment. The wind rose map (Fig. 5c) reveals the significance of the droopy tail angle of the nano-silica and reserpine groups compared with the control group [(silica-100 vs. wild type): P = 0.036; (silica-1000 vs. wild type): P < 0.001; (RSP-1 vs. wild type): P < 0.001; (RSP-10 vs. wild type): P < 0.001]. Both nano-silica and reserpine increase the tail drop angle compared with the wild type (Fig. 5i), which are measured by one-way ANOVA (F4,70 = 13.94, P < 0.0001). A multiple T post hoc test reveals that both nano-silica and reserpine significantly increase the tail drop angle [(silica-100 vs. wild type): t = 2.18, df = 34, P = 0.0360; (silica-1000 vs. wild type): t = 7.22, df = 33, p < 0.0001; (RSP-1 vs. wild type): t = 7.16, df = 36, p < 0.0001; (RSP-10 vs. wild type): t = 7.68, df = 36, p < 0.0001].
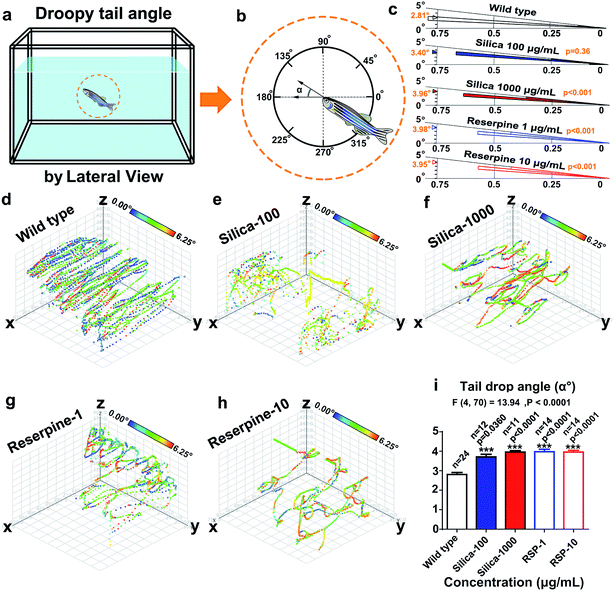 |
| Fig. 5 The similar effect of nano-silica and reserpine on increasing depression behavior in the novel tank test. Diagram of the swimming pattern (a) and (b) definition of the “droopy tail” angle. (c) Polar directional histograms (wind direction rose map) demonstrated the value and directional distribution of the droop tail angle. The radial length of sector i represented the proportion of the droopy tail angle (i°, ∼i + 1°) out of the total angle. The numerical value of the radial lengths of all sectors in each rose map adds up to 1. The small triangle indicates the mean value. The instantaneous droopy tail angles at every point in the trajectories were color-coded according to the value (d–h); the legend colour scales represent the proportional spectrum across the minimum/maximum ranges of the droopy tail angle. (i) Histogram of the droopy tail angle for each treatment. Both nano-silica and reserpine increase the tail drop angle compared with the wild type. | |
Furthermore, the instantaneous “droop tail angle” dynamics are demonstrated by the color-coded angle values in each location in the swimming path (Fig. 5d–h). The color scales in the legend represent the proportional spectrum across the minimum/maximum ranges of the “droop tail angle” values. Both nano-silica and reserpine inhibit the spatiotemporal swimming paths of zebrafish. High values of “droopy tail angle” (color-coded by yellow or red) usually occur in the upper part of the swimming path (ESI Fig. S6–S10†).
Depressive and anxiety behavior such as (Fig. 6c) freezing bouts have been enhanced by silica or reserpine treatment, which are revealed by one-way ANOVA (F4,70 = 18.67, P < 0.0001). Multiple T post hoc tests demonstrate that both nano-silica and reserpine significantly increase the tail drop angle [(silica-100 vs. wild type): t = 2.20, df = 34, P = 0.0348; (RSP-1 vs. wild type): t = 5.17, df = 36, p < 0.0001; (RSP-10 vs. wild type): t = 8.30, df = 36, p < 0.0001; (silica-100 vs. RSP-1): t = 3.53, df = 24, P = 0.0017; (silica-1000 vs. RSP-1): t = 5.67, df = 24, p < 0.0001; (silica-100 vs. RSP-10): t = 5.67, df = 24, p < 0.0001; (silica-1000 vs. RSP-10): t = 5.39, df = 23, P < 0.0001].
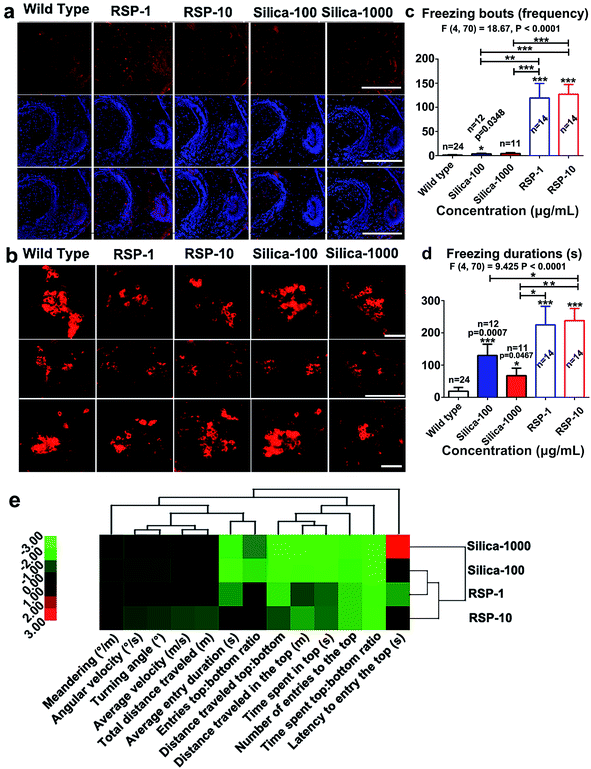 |
| Fig. 6 Depressive phenotype caused by nano-silica or reserpine. Examination of expression of (a) serotonin in the hypothalamus and (b) tyrosine hydroxylase in brain tissues treated by nano-silica or reserpine. In (b), the upper and lower panels showed the left and right populations of TH positive cells in the middle panel. The depressive behavior such as (c) freezing bouts and (d) freezing duration have been enhanced by both reserpine and nano-silica. (e) Hierarchical clustering revealed the similarity between nano-silica and reserpine on altering the whole behavioral profiles in the novel tank test. The data are expressed as the mean + S.E.M. and were analyzed by one-way ANOVA followed by a Turkey post hoc test. Significance was defined as *p < 0.05, **p < 0.01, and ***p < 0.001. Scale bars, 400 μm. | |
One-way ANOVA demonstrates the significant effect of nano-silica or reserpine on the freezing duration (Fig. 6d) (F4,70 = 9.425, P < 0.0001). The freezing duration has been enhanced by both reserpine and nano-silica, which is revealed by multiple T post hoc tests [(silica-100 vs. wild type): t = 3.75, df = 34, p = 0.0007; (silica-1000 vs. wild type): t = 2.07, df = 33, p = 0.0467; (RSP-1 vs. wild type): t = 4.47, df = 36, P < 0.0001; (RSP-10 vs. wild type): t = 6.70, df = 36, P < 0.0001; (silica-100 vs. RSP-10): t = 2.07, df = 24, P = 0.0496; (silica-1000 vs. RSP-1): t = 2.31, df = 23, p = 0.0301; (silica-1000 vs. RSP-10): t = 3.58, df = 23, P = 0.0016].
Hierarchical clustering revealed the comparability of the depressive effect of nano-silica or reserpine on the whole behavioral profiles of zebrafish in the novel tank test (Fig. 6e). The locomotive and exploratory behaviors are suppressed by both nano-silica and reserpine with low or high concentrations. The degree of similarity between the depressive effects of the four pharmacological manipulations suggests that the low dose of nano-silica (100 μg mL−1) and the low dose of reserpine (1 μg mL−1) produced similar degrees of alteration in all of the behavioral parameters.
Conclusion
The novel tank diving test in this experiment compared the similar anxiogenic effects induced by chronic nano-silica versus chronic reserpine exposure. The anxiogenic effects of nano-silica and reserpine have been demonstrated to increase anxiety behavior, decreasing locomotion (total distance traveled, average velocity, turn angle and angular velocity); increasing erratic movements (meandering); reducing exploratory behavior to the top (average entry duration in the top, distance traveled in the top, time spent in the top, latency to enter the top, number of entries to the top, time spent ratio of top
:
bottom, distance traveled ratio of top
:
bottom and entries ratio of top
:
bottom); and enhancing depressive phenotype (freezing bouts, freezing durations and droopy tail angle). Further, 3D-reconstruction demonstrates that nano-silica or reserpine narrow the swimming path of zebrafish by confining their area of movement to the bottom of the novel tank, while spatiotemporal 3D-reconstruction revealed the similar effects between nano-silica and reserpine on instantaneous locomotive activities at each location in the swimming path. Together, the novel tank test indicates the similar effects of nano-silica and reserpine on decreasing locomotion, inhibiting exploratory behaviors and enhancing anxiety phenotype.
In this study, we found that nano-silica, similar to reserpine, could increase anxious behavior and decrease locomotive activity in adult zebrafish. Deficiency in the 5-HT system is accompanied by increased depression and anxiety-like behaviors in adult mice.38 In zebrafish, Buspirone (5HT 1A receptor agonist), a serotonergic anxiolytic drug can decrease the depression and anxiety-like behavior during the novel tank diving response.28 Thus the effect of anxiogenic or anxiolytic drugs affecting the 5-HT system in zebrafish behavior also can be a read-out for anxiety or fear.36 The serotonergic system in zebrafish is also involved in depression and anxiety behavior. Here the number of serotonin-containing neurons decreased in the brain tissues of the RSP and nano-silica groups, which is in accordance with the depression behavior detected in our behavior test. This result suggested that the reserpine and nano-silica can induce depression by decreasing the serotonin in adult zebrafish brain tissues.
Decreased locomotive activity was found in the RSP and nano-silica treated groups. Decreased locomotive activity is a characteristic of Parkinson’s disease which occurs as a result of the progressive loss of DA cells in the substantia nigra. Here, tyrosine hydroxylase expression in the posterior tuberculum was decreased in the reserpine and nano-silica groups, which is also consistent with the behavior test. This finding demonstrated that RSP and nano-silica could induce Parkinson’s disease by reducing the number of DA cells.
In summary, this research provides a description of the similar effect between nano-silica and reserpine on the locomotive, exploratory, and anxious behaviors of adult zebrafish in the novel tank test. The behavior test demonstrates that nano-silica is as anxiogenic as reserpine. Both nano-silica and reserpine have an anxiogenic effect by decreasing locomotion, inhibiting exploratory behaviors and aggravating the depressive phenotype. Furthermore, immunohistochemistry reveals that the similar behavioral and anxiogenic effects of nano-silica and reserpine may share a common physiological mechanism. Dampened locomotion induced by nano-silica and reserpine is associated with the decreased expression of tyrosine hydroxylase, and inhibited exploratory behavior, aggravated anxiety phenotypes, and disturbed spatiotemporal swimming path patterns are related to the increased expression of serotonin. Further investigation should be conducted to decipher the common genetic and molecular mechanisms of the behavioural and physiological changes induced by nano-silica and reserpine.
Conflict of interests
The authors declare no conflict of interests.
Acknowledgements
We thank the Special Fund for Basic Research on Scientific Instruments from the Chinese National Natural Science Foundation (grant no. 61327802) and National Basic Research Program of China (2015CB856500) for their support of this work. D. F. F. acknowledges support from the National Natural Science Foundation of China (Grant No. 81501589). D. Y. C. also acknowledges support from the Tianjin Science and Technology Research Funds of China (grant no. 14JCQNJC09600). X. Z. F. acknowledges support from the State Key Laboratory of Medicinal Chemical Biology, Nankai University, China (grant no. 201501019).
References
- I. I. Slowing, B. G. Trewyn and V. S.-Y. Lin, J. Am. Chem. Soc., 2007, 129, 8845–8849 CrossRef CAS PubMed
. - M. Bottini, F. D. Annibale, A. Magrini, F. Cerignoli, Y. Arimura, M. I. Dawson, E. Bergamaschi, N. Rosato, A. Bergamaschi and T. Mustelin, Int. J. Nanomed., 2007, 2, 227 CAS
. - L. R. Hirsch, R. Stafford, J. Bankson, S. Sershen, B. Rivera, R. Price, J. Hazle, N. J. Halas and J. West, Proc. Natl. Acad. Sci. U. S. A., 2003, 100, 13549–13554 CrossRef CAS PubMed
. - M. V. Park, H. W. Verharen, E. Zwart, L. G. Hernandez, J. van Benthem, A. Elsaesser, C. Barnes, G. McKerr, C. V. Howard and A. Salvati, Nanotoxicology, 2011, 5, 168–181 CrossRef CAS PubMed
. - P. C. Ray, H. Yu and P. P. Fu, J. Environ. Sci. Health, Part C: Environ. Carcinog. Ecotoxicol. Rev., 2009, 27, 1–35 CrossRef CAS PubMed
. - X. Li, B. Liu, X.-L. Li, Y.-X. Li, M.-Z. Sun, D.-Y. Chen, X. Zhao and X.-Z. Feng, Sci. Rep., 2014, 4, 3810 Search PubMed
. - J. Marks, Br. J. Ind. Med., 1957, 14, 81 CAS
. - W. Lin, Y.-W. Huang, X.-D. Zhou and Y. Ma, Toxicol. Appl. Pharmacol., 2006, 217, 252–259 CrossRef CAS PubMed
. - A. Allison, J. Harington and M. Birbeck, J. Exp. Med., 1966, 124, 141–154 CrossRef CAS PubMed
. - E. J. Park and K. Park, Toxicol. Lett., 2009, 184, 18–25 CrossRef CAS PubMed
. - X. Cao, W. W. Deng, M. Fu, L. Wang, S. S. Tong, Y. W. Wei, Y. Xu, W. Y. Su, X. Xu and J. N. Yu, Int. J. Nanomed., 2012, 7, 753–762 CAS
. - F. Koch, A. M. Möller, M. Frenz, U. Pieles, K. Kuehni-Boghenbor and M. Mevissen, Toxicol. in Vitro, 2014, 28, 990–998 CrossRef CAS PubMed
. - R. Sood, M. A. English, M. P. Jones, J. Mullikin, D. M. Wang, M. Anderson, D. Wu, S. C. Chandrasekharappa, J. Yu and J. Zhang, Methods, 2006, 39, 220–227 CrossRef CAS PubMed
. - D. Chatterjee and R. Gerlai, Behav. Brain Res., 2009, 200, 208–213 CrossRef CAS PubMed
. - A. V. Kalueff, M. Gebhardt, A. M. Stewart, J. M. Cachat, M. Brimmer, J. S. Chawla, C. Craddock, E. J. Kyzar, A. Roth and S. Landsman, Zebrafish, 2013, 10, 70–86 CrossRef PubMed
. - B. D. Robison, M. J. Benner, M. L. Singer and M. E. Oswald, A High-Throughput and Inexpensive Assay for Anxiety-Related Behaviors, 2012 Search PubMed
. - S. Jesuthasan, Dev. Neurobiol., 2012, 72, 395–403 CrossRef PubMed
. - M. Nguyen, E. Yang, N. Neelkantan, A. Mikhaylova, R. Arnold, M. K. Poudel, A. M. Stewart and A. V. Kalueff, Behav. Brain Res., 2013, 256, 172–187 CrossRef PubMed
. - J. Cachat, A. Stewart, L. Grossman, S. Gaikwad, F. Kadri, K. M. Chung, N. Wu, K. Wong, S. Roy and C. Suciu, Nat. Protoc., 2010, 5, 1786–1799 CrossRef CAS PubMed
. - A. V. Kalueff, D. J. Echevarria and A. M. Stewart, Prog. Neuro-Psychopharmacol. Biol. Psychiatry, 2014, 55, 1–6 CrossRef PubMed
. - A. Stewart, F. Kadri, J. DiLeo, K. Min Chung, J. Cachat, J. Goodspeed, C. Suciu, S. Roy, S. Gaikwad and K. Wong, Int. J. Comp. Psychol., 2010, 23, 104–121 Search PubMed
. - E. D. Freis, N. Engl. J. Med., 1954, 251, 1006–1008 CrossRef CAS PubMed
. - E. Kyzar, A. M. Stewart, S. Landsman, C. Collins, M. Gebhardt, K. Robinson and A. V. Kalueff, Brain Res., 2013, 1527, 108–116 CrossRef CAS PubMed
. - M. Nguyen, A. M. Stewart and A. V. Kalueff, Prog. Neuro-Psychopharmacol. Biol. Psychiatry, 2014, 55, 26–39 CrossRef CAS PubMed
. - M. A. Akimenko, S. L. Johnson and M. Westerfield, Development, 1995, 121(2), 347–357 CAS
. - R. J. Egan, C. L. Bergner, P. C. Hart, J. M. Cachat, P. R. Canavello, M. F. Elegante, S. I. Elkhayat, B. K. Bartels, A. K. Tien and D. H. Tien, Behav. Brain Res., 2009, 205, 38–44 CrossRef CAS PubMed
. - E. D. Levin, Z. Bencan and D. T. Cerutti, Physiol. Behav., 2007, 90, 54–58 CrossRef CAS PubMed
. - Z. Bencan, D. Sledge and E. D. Levin, Pharmacol., Biochem. Behav., 2009, 94, 75–80 CrossRef CAS PubMed
. - A. V. Kalueff and J. M. Cachat, Zebrafish neurobehavioral protocols, Springer, 2011 Search PubMed
. - J. M. Cachat, P. R. Canavello, S. I. Elkhayat, B. K. Bartels, P. C. Hart, M. F. Elegante, E. C. Beeson, A. L. Laffoon, W. A. Haymore and D. H. Tien, Zebrafish neurobehavioral protocols, 2011, pp. 1–14 Search PubMed
. - W. Shannon, R. Culverhouse and J. Duncan, Pharmacogenomics, 2003, 4, 41–52 CrossRef CAS PubMed
. - P. Berens, J. Stat. Software, 2009, 31, 1–21 Search PubMed
. - R. Muheim, S. Sjöberg and A. Pinzon-Rodriguez, Proc. Natl. Acad. Sci. U. S. A., 2016, 113, 1654–1659 CrossRef CAS PubMed
. - A. M. Stewart, F. Grieco, R. A. Tegelenbosch, E. J. Kyzar, M. Nguyen, A. Kaluyeva, C. Song, L. P. Noldus and A. V. Kalueff, J. Neurosci. Methods, 2015, 255, 66–74 CrossRef PubMed
. - J. Cachat, A. Stewart, E. Utterback, P. Hart, S. Gaikwad, K. Wong, E. Kyzar, N. Wu and A. V. Kalueff, PLoS One, 2011, 6, e17597 CAS
. - C. Lillesaar, J. Chem. Neuroanat., 2011, 41, 294–308 CrossRef CAS PubMed
. - S. Bretaud, S. Lee and S. Guo, Neurotoxicol. Teratol., 2004, 26, 857–864 CrossRef CAS PubMed
. - T. J. Hendricks, D. V. Fyodorov, L. J. Wegman, N. B. Lelutiu, E. A. Pehek, B. Yamamoto, J. Silver, E. J. Weeber, J. D. Sweatt and E. S. Deneris, Neuron, 2003, 37, 233–247 CrossRef CAS PubMed
.
Footnotes |
† Electronic supplementary information (ESI) available. See DOI: 10.1039/c6ra24215d |
‡ These authors contributed equally to this work. |
|
This journal is © The Royal Society of Chemistry 2017 |
Click here to see how this site uses Cookies. View our privacy policy here.