DOI:
10.1039/C6RA23786J
(Paper)
RSC Adv., 2016,
6, 113315-113321
Protein-induced ultrathin molybdenum disulfide (MoS2) flakes for a water-based lubricating system
Received
24th September 2016
, Accepted 24th November 2016
First published on 25th November 2016
Abstract
Monolayer or ultrathin transition metal dichalcogenides cover a wide range of atomically thin two-dimensional (2D) materials, whose fascinating properties have made them promising candidates for many applications. In this work, ultrathin MoS2 flakes were successfully exfoliated, induced by bovine serum albumin (BSA) or hemoglobin (HB), under vigorous ultra-sonication. They were used as additives for water-based lubricating systems and related investigations indicated that they could improve the friction performance and anti-wear abilities at a relatively low concentration: 0.10 wt% of additive contents can make the average friction coefficient stabilize in the range between 0.06 and 0.08, which results from the formation of a dynamic protective film on the friction surfaces. The protein-induced ultrathin MoS2 flakes are also expected to be useful for other hydrophilic lubrication systems, which possess great scientific value and promising application prospects compared with bulk or microscale lubricating additives.
1. Introduction
Monolayer or few-layer 2-D transition metal dichalcogenides (TMDS, illustrated as NX2, N = Mo, W, etc.; X = S, Se, etc.) have attracted more and more attention due to their special properties, such as their semiconducting effect, sizable band gap and large surface area, and their promising applications in sensing catalysis, hydrogen storage, and lubricating additives.1–5 As a fascinating member of TMDS, MoS2 has elicited numerous studies because of its unique layered structure and interesting functional properties.6–8 Natural MoS2 occurs as a lamellar hexagonal architecture composed of stacked atomic layers (S–Mo–S), which are held together by weak van der Waals interactions.9–11 Interesting properties which are different from bulk MoS2 will appear when it is downsized to single or few layer structures.12,13 For example, exfoliated MoS2 slices possess excellent catalytic properties for the electrochemical hydrogen evolution reaction,14–16 and ultrathin MoS2 exhibits photo-electrochemical and photo-response activity.17 It is believed that 2-D MoS2 with ordered structures will have more promising applications in super lubrication through band gap transformation from an indirect way to a direct way for monolayers.18–21 Obviously, these special properties of 2-D MoS2 are owing to the ultrathin thickness at atomic scale, thus thickness control is a crucial factor for ultrathin MoS2.
There are many ways to achieve ultrathin MoS2 flakes.22,23 Mechanical exfoliation by scotch-tape is just a simple way, but its yield is too low, and the shape and thickness control is difficult. Chemical vapor deposition (CVD) is another important method which has been successfully used to synthesize high-quality MoS2 sheets in a controllable manner with needed compositions and controlled number of layers, although it is not easy to scale-up till now.24 Compared with mechanical exfoliation and CVD, liquid phase exfoliation (LPE) is a convenient and cost-effective way to obtain exfoliated MoS2, which meet the requirements of high yield and large-scale production at the same time.25 Researchers have tried many different ways to develop LPE method. Highly exfoliated MoS2 sheets can be prepared by lithium ion intercalation and subsequent thermal shock or ultrasonication in a liquid phase.26 Liu et al. gave a resolution of solvo-thermal method for producing excellent nanosheets from bulk MoS2, hexagonal boron nitride (h-BN) and graphite,27 while Li et al. prepared 2-D MoS2 from sodium molybdate dehydrate via hydrothermal method and the subsequent water-exfoliation.28 In addition, sonication LPE assisted by proteins is showing a wide, convenient and efficient application in 2-D MoS2 materials. In this case, proteins adsorbed MoS2 nanoparticles slide against the layers below, and the freshly exposed surfaces are covered to prevent the reverse sliding subsequently; finally bulk MoS2 is stripped and ultrathin MoS2 comes into being.1 Furthermore, protein adsorbed MoS2 exhibited excellent dispersion capability in water. The protein molecules possess a variety of functional groups, including benzene rings, carboxyl, hydroxyl and amino group, etc., parts of these groups can stably bind or adsorb on the surface of MoS2 crystals, resulting hydrophilic MoS2 ultrathin flakes.
On the other hand, friction is a general phenomenon in nature and industry, while lubrication is a ways to improve energy efficiency. On the surface of friction pairs, there are “ridges”, “valleys”, “asperities” and “depressions” at micro- or nano- scale. Effective controls or reductions of the friction in mechanical systems are important for a sustainable consideration. Lubricating oils and water-based lubricating systems are widely used in most kinds of machines for friction reduction, and how to enhance their lubricating properties has been an important challenge. The design of new friction modification and lubrication additives based on 2-D materials which offered solutions to lubrication challenges in a variety of economically and technologically critical sectors of society represents some unique and challenging opportunities. From the viewpoint of additives, more close combination of novel 2-D materials and base oil can offer new approaches to enhancing the friction reduction capabilities of liquid lubricants, in which solubility, dispersibility and particle degradation will be improved when compared with other conventional solid additives. Similar solutions may be on the horizon for improving the tribological properties of water based lubricating system using 2-D MoS2 materials. In this work, protein-induced ultrathin MoS2 flakes which possess good hydrophilicity were involved for this attempt.
2. Experimental
2.1 Materials
BSA and HB from bovine blood were provided by Shanghai Shi fang biological technology Co. LTD. Bulk MoS2 was purchased from Sinopharm Chemical Reagent Company (Shanghai, China). All chemicals were used as received and the aqueous solution was prepared by deionized water.
2.2 Preparations
The protein-induced exfoliation of ultrathin MoS2 was carried out via an ultrasonic way: certain amount of bulk MoS2 powders were added into 20 mL deionized water containing 20 mg BSA, followed by ultra-sonication for 48 hour under controlled temperature at 25 °C. After ultra-sonication, the suspension was centrifuged at 7000 rpm for 40 minutes; the collected precipitants were re-dispersed into water under ultra-sonication for 20 minutes. The resulting solution was then centrifuged for 40 minutes to remove unexfoliated MoS2 residues, and the obtained suspension was denoted as BSA–MoS2. Similarly, HB–MoS2 can be obtained when BSA was replaced by HB in the above procedure. For comparison, bulk MoS2 was also dealt with under similar ultra-sonication and centrifugation procedure without protein addition.
2.3 Techniques
X-ray diffraction (XRD) patterns were obtained by using a Rigaku K/max-γA X-ray diffractometer with a Cu Kα (λ = 1.5415 Å) at the scanning rate of 0.2° s−1; thermogravimetric analysis (TGA) was performed on a Netzsch STA-409c Thermal Analyzer under a 50 × 103 mm3 min−1 nitrogen flow with the heating rate of 10 °C min−1; UV-Vis absorption spectra was recorded on a UV-3600 spectrometer; scanning electron microscopy (SEM) images were obtained by using the FEI Inspect F50 instrument. Transmission electron microscopy (TEM) images were performed on a JEM-2100 transmission electron microscope. Optical photographs were taken by a digital camera. The tribological properties were tested on an MSR-10A four-ball test machine. The friction and wear tests were conducted at a rotating speed of 1200 rpm under the applied loads for 147 N for duration of 15 min.
3. Results and discussion
3.1 Characterizations of ultrathin MoS2
Ultrathin MoS2 can be obtained by protein-induced exfoliation of bulk MoS2 powders under ultra-sonication, as illustrated in Fig. 1. Firstly, protein molecules are strongly adsorbed on MoS2 surface via binding energy. During ultra-sonication process, layer-by-layer exfoliation and fracture of MoS2 flakes take place gradually. The obtained protein-induced MoS2 were characterized and analyzed by several ways, which can give confirmation of its structure and morphology.
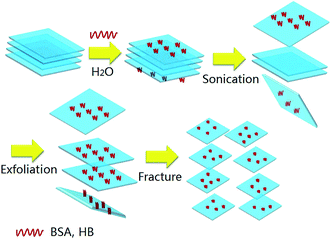 |
| Fig. 1 Ultrathin MoS2 sheets obtained by protein-induced exfoliation under ultra-sonication. | |
UV-Vis absorption spectra of protein-induced ultrathin MoS2 and bulk MoS2 are shown in Fig. 2. The characteristic absorption peaks for HB (at 413 nm) and BSA (at 291 nm) in the visible radiation region are clearly observed. After ultra-sonication, two absorptive peaks at 618 nm and 678 nm can be observed in the curves for BSA–MoS2 and HB–MoS2, which are attributed to the direct-gap absorptions and excitonic transitions in MoS2 sheets generated from energy split from valence band spin–orbital coupling.29–31 In comparison to bulk MoS2, the wide peak between 400 nm and 600 nm is owing to the direct transition from the deep valence band to the conduction band.
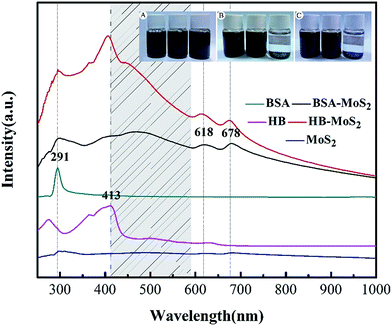 |
| Fig. 2 UV-Vis spectra of the protein-induced ultrathin MoS2 nanosheets and bulk MoS2 dispersed in deionized water. Inserted picture: digital camera images of as-prepared MoS2 nanosheets dispersed in deionized water after sonication (A), 1 day later (B), 7 days later (C), in each image from left to right is bottle of BSA–MoS2, HB–MoS2 and bulk MoS2 suspensions, respectively. | |
The dispersion stability and durability of BSA–MoS2 and HB–MoS2 in water can be improved after protein modification. These dispersion samples have been examined in a simple way: the bulk MoS2, BSA–MoS2 and HB–MoS2 suspensions after 48 h bath ultra-sonication are allowed to settle for several days. It is found that there is no obvious precipitation in the colloid suspensions of as-prepared MoS2 after 7 days (as shown in Fig. 2Cins). According to previous studies,1 protein molecules are strongly adsorbed on MoS2 surface through its functional group, including carboxyl, hydroxyl and amino group, etc. When protein modified MoS2 is dispersed in water, these hydrophilic functional groups play a very important role in improving its dispersion stability and durability. Due to the existing of hydrophilic functional group adsorbed on the MoS2 flakes, the modified MoS2 becomes more hydrophilic, thus better dispersion stability. However, bulk MoS2 suspension exhibited only short-term stability and precipitated completely after one day (as shown in Fig. 2Bins). This indicates that protein-induced ultrathin MoS2 sheets have good dispersion stability in water.
Fig. 3 gives XRD results of bulk MoS2, BSA–MoS2, and HB–MoS2. The diffractive peaks at 32.7°, 39.0°, 56.8° are in good agreement with (100), (103), (110) planes of the MoS2 hexagonal structure, although these peaks are quite weaker and broader in the patterns of BSA–MoS2 and HB–MoS2. Furthermore, the diffractive peak corresponding to (002) plane of MoS2 is significantly broadened and its intensity is decreased much, which suggests less stacking and more ultrathin MoS2 sheets in the direction of the Z-axis perpendicular to atomic layers produced. These results are consistent with the microscopic morphology of bulk MoS2, BSA–MoS2 and HB–MoS2 characterized by SEM, as shown in the inserted picture of Fig. 3. Obviously, bulk MoS2 presents a clear multilayer with large lateral flake dimension (Fig. 3Ains). However, protein-induced MoS2 samples (as shown in Fig. 3Bins and Cins) exhibit a reassembled sheet-like structure with the lateral sizes of hundreds of nanometers, and ultrathin layer with a smooth surface can be observed from the exfoliated MoS2. In comparison, the efficiency of HB on MoS2 exfoliation is higher than that of BSA, it may be due to the HB molecule has stronger binding energy than BSA on MoS2 edge.
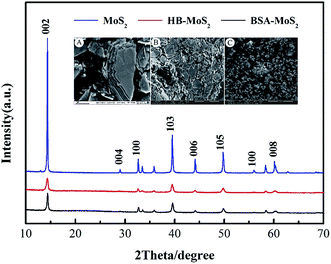 |
| Fig. 3 X-ray diffraction spectra of the bulk MoS2, BSA–MoS2 and HB–MoS2. Inserted picture: scanning electron microscope (SEM) images of (A) bulk MoS2, (B) BSA–MoS2 and (C) BSA–MoS2. | |
TGA results of bulk MoS2, BSA–MoS2 and HB–MoS2 shown in Fig. 4 give supplementary and supportive evidences for protein-induced exfoliation. TGA curves for BSA–MoS2 and HB–MoS2 can be divided into three stages: little weight loss below 100 °C can be assigned to the absorbed water; the weight loss from 200 °C to 360 °C is mainly due to the thermal decomposition of protein. The weight loss from 400 °C and 800 °C is correspond to the decomposition of MoS2, which is similar to the curve for bulk MoS2.
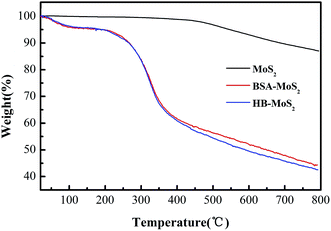 |
| Fig. 4 TGA curves of bulk MoS2, BSA–MoS2 and HB–MoS2. | |
The ultrathin structure is confirmed by TEM. From Fig. 5A and B, transparent BSA–MoS2 and HB–MoS2 flakes with an average size of about 100 nm can be observed, implying that the sheets are quite thin when comparing with bulk MoS2 shown in Fig. 5C. In addition, HR-TEM images of protein-induced MoS2 indicate perfect hexagonal structure with a lattice parameter of 0.27 nm, corresponding to its lattice spacing of (100) plane. Selected area electron diffraction (SAED) patterns (the inset of Fig. 5A and B) reveal that ultrathin MoS2 possesses a hexagonal symmetry structure, which indicate that protein-induced treatment does not damage its original nanostructure.
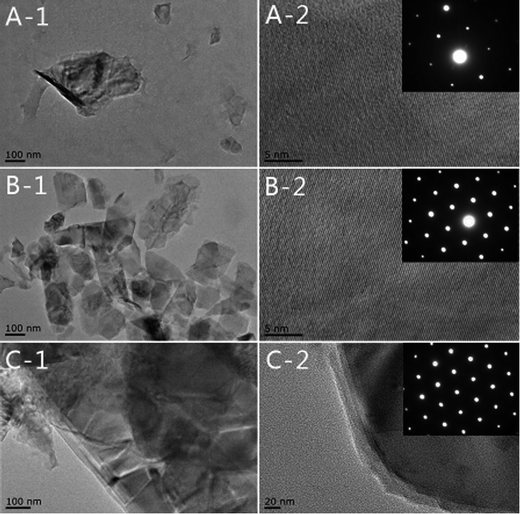 |
| Fig. 5 TEM (A-1, B-1, C-1, C-2), HRTEM (A-2, B-2) images of the ultrathin MoS2 prepared by different methods. BSA–MoS2 (A-1, A-2); HB–MoS2 (B-1, B-2); bulk MoS2 sonication for 48 h (C-1, C-2). Inserted picture: selected area electron diffraction (SAED) pattern of each sample. | |
3.2 Tribological properties
According to our experimental results, the boundary lubrication would occur under a load of 147 N. The lubrication will be failed in 3 minutes for pure water (the calculated average value of friction coefficient is 0.383); while water-based system containing BSA–MoS2 or HB–MoS2 are quite effective in reducing the friction coefficient. The real-time changing tendency of friction coefficient for water-based system containing BSA–MoS2 is shown in Fig. 6. With BSA–MoS2 concentration increasing, the friction coefficient is dropped remarkably. When concentration is more than 0.10 wt%, the average values of friction coefficient are stabilized in the range between 0.06 and 0.08 (Fig. 7), which gives a reduction of 85% when compared with pure water system. As we known, when the worn surface is lubricated by pure water, friction pairs contact directly due to poor separation ability of water, the rough bumps can form rapidly and further scratched the surface, and then dry friction will occur in the friction experiment. Ultrathin MoS2 has great specific surface area, which helps to form protective film and prevent direct metal friction and abrasion wear. In addition, ultrathin MoS2 sheets can easily enter and stay in the contact area of the worn surfaces, ensure the integrity of the lubricating film, thus the stability of the friction coefficient. At the same time, the curve of the wear scar diameter (WSD) gives similar tendency with the friction coefficient, which indicates that BSA–MoS2 can improve the anti-wear performance of water-based lubricating system. As shown in the inserted picture of Fig. 7, the surfaces of friction pairs lubricated by water-based system containing 0.10 wt%, 0.13 wt%, and 0.16 wt% of BSA–MoS2 are smoother than that lubricated with water-based system containing 0.01 wt%, 0.04 wt% of BSA–MoS2, and the related furrows on wear scar surfaces are shallower and narrower. The reason can be attributed to “separate effect” caused by ultrathin MoS2. At a proper concentration, a homogenous and dense protective film will form on the frictional surface, which can partly avoid the direct contact of rough surfaces; as a result, shallower and narrower furrows can be achieved. On the other hand, the dense lubrication film is difficult to form at a lower concentration, some ‘peaks’ on the rubbing surfaces will be in contact directly, resulting in thick and deep furrows.
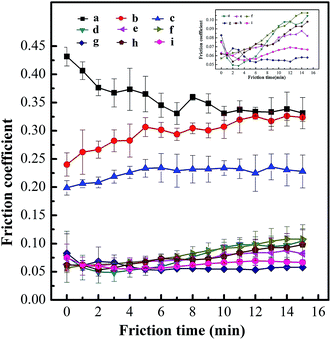 |
| Fig. 6 Real-time changing tendency of friction coefficient with time obtained from water-based lubricating systems with different BSA–MoS2 concentration. (a) 0.01 wt%; (b) 0.04 wt%; (c) 0.07 wt%; (d) 0.10 wt%; (e) 0.13 wt%; (f) 0.16 wt%; (g) 0.19 wt%; (h) 0.22 wt%; (i) 0.25 wt%. | |
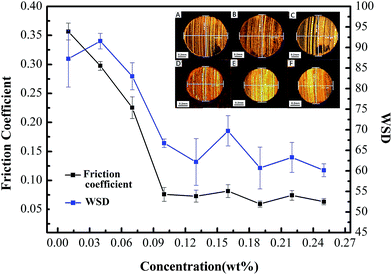 |
| Fig. 7 Average friction coefficient and wear scar diameter obtained from water-based lubricating systems with different BSA–MoS2 concentration (the unit of WSD is 0.01 mm). Inserted picture: the wear scar photos of the steel balls after friction test for water-based lubricating systems containing (A) 0.01 wt%; (B) 0.04 wt%; (C) 0.07 wt%; (D) 0.10 wt%; (E) 0.13 wt%; (F) 0.16 wt% of BSA–MoS2. | |
Meanwhile, similar behaviors in improving the friction performance can be observed in the water-based system containing ultrathin BSA–MoS2 flakes (in our experiments, 0.10 wt% is the optimal addition ratio, corresponding to the smallest friction coefficient of 0.07 similarly) when compared with BSA–MoS2, as shown in Fig. 8. On the other hand, 0.10 wt% of bulk MoS2 cannot decrease the friction coefficient of water-based lubricating system. These obvious contrast data reconfirms that ultrathin BSA–MoS2 and HB–MoS2 flakes are useful additives for improving the friction-reduction and anti-wear ability of water-based lubricating system.
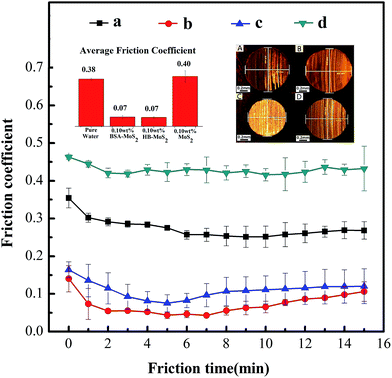 |
| Fig. 8 Real-time changing tendency of friction coefficient with time obtained from water-based lubricating systems with different MoS2 concentration: (a) 0.01 wt% of HB–MoS2; (b) 0.10 wt% of HB–MoS2; (c) 0.19 wt% of HB–MoS2; (d) 0.10 wt% of bulk MoS2. Inserted picture on the top left corner: average friction coefficient for pure water; water-based lubricating systems with 0.10 wt% of BSA–MoS2; 0.10 wt% HB–MoS2; 0.10 wt% of bulk MoS2 Inserted picture on the top right corner: the wear scar photos of the steel balls after friction test water-based lubricating systems with (A) 0.01 wt% HB–MoS2; (B) 0.10 wt% of bulk MoS2; (C) 0.10 wt% HB–MoS2; (D) 0.19 wt% HB–MoS2 respectively. | |
3.3 Related mechanism
Fig. 9 suggests one possible mechanism for ultrathin MoS2 flakes improving lubricating performance of water-based system. When the worn surface lubricated by pure water, many ‘peaks’ on the rubbing surfaces will be in contact directly due to poor separation ability of pure water, thus a rough surface corresponds to rather higher friction coefficient in the friction experiment.32,33 When the worn surface lubricated with pure water containing certain concentration (below 0.10 wt%) of ultrathin MoS2 (Fig. 9b), lower friction coefficient and smooth worn surface can be achieved, because MoS2 flakes can separate ‘peaks’ on the rubbing surfaces. When the friction pairs are lubricated by water-based system with higher concentration (above 0.10 wt%) of ultrathin MoS2 (Fig. 9c), ultrathin MoS2 would separate all ‘peaks’ and fill the micro-scratches on the rubbing surface.
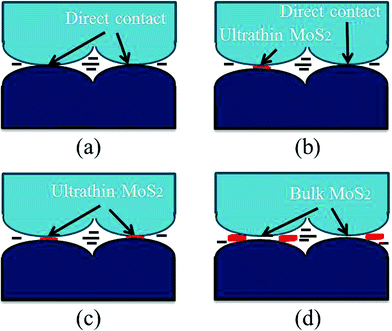 |
| Fig. 9 Illustration of the lubrication mechanism. (a) Pure water with no additives; (b) water-based lubricating system with small concentration of ultrathin MoS2; (c) water-based lubricating system with higher concentration of ultrathin MoS2; (d) water-based lubricating system with bulk MoS2. | |
Consequently, the thick, deep furrows on the worn surface cannot be found as shown in Fig. 7 (inserted picture D, E, F) and Fig. 8 (inserted picture on the top right corner, C, D). Together with the good dispersion in pure water, ultrathin MoS2 sheets can easily enter and stay in the contact area of the worn surfaces and serve as ‘nanofillers’ to form a surface protective and lubricious layer, which can result in effective friction reduction. Furthermore, ultrathin MoS2 sheets could provide quite easier shear and slider between friction pairs. As for 0.10 wt% of bulk MoS2 (Fig. 9d), their being difficult to be exfoliated makes it hard to enter the contact areas and work as an effective lubricant, which leads to an unexpected lubricating effects.
4. Conclusions
Protein-induced ultrathin MoS2 (BSA–MoS2 and HB–MoS2) were successfully synthesized via ultra-sonication based exfoliation. Both BSA–MoS2 and HB–MoS2 possess good dispersion and stability in pure water, which was advantageous to be used as water-based lubricating additives. In comparison to pure water and water-based lubricating system containing bulk MoS2, 0.10 wt% of BSA–MoS2 and HB–MoS2 can decrease the friction coefficient and wear scar diameter effectively. These results indicated that protein-induced ultrathin MoS2 had a good potential for the application as water-based lubricating additives.
Acknowledgements
This work is financially supported by the National Natural Science Foundation of China (U1332134, 51635004), the Natural Science Foundation of Jiangsu Province (BK20150505), the Natural Science Foundation of Suzhou (SYG201329), the Fundamental Research Funds for the Central Universities (3202006301, 3202006403), the Qing Lan Project and the International Foundation for Science, Stockholm, Sweden, the Organization for the Prohibition of Chemical Weapons, The Hague, Netherlands, through a grant to Lei Liu (F/4736-2) and the Tribology Science Fund of State Key Laboratory of Tribology (SKLTKF15A11).
References
- G. J. Guan, S. G. Zhang, S. H. Liu, Y. Q. Cai, M. Low, C. P. Teng, I. Y. Phang, Y. Cheng, K. L. Duei, B. M. Srinivasan, Y. G. Zheng, Y. W. Zhang and M. Y. Han, Protein Induces Layer-by-Layer Exfoliation of Transition Metal Dichalcogenides, J. Am. Chem. Soc., 2015, 137, 6152–6155 CrossRef CAS PubMed.
- R. R. Chianelli, E. B. Prestridge, T. A. Pecoraro and J. P. Deneufville, Molybdenum disulfide in the poorly crystalline“Rag” structure, Science, 1979, 203(4385), 1105–1107 CAS.
- Z. Chen, X. W. Liu, Y. H. Liu, S. Gunsel and J. B. Luo, Ultrathin MoS2 Nanosheets with Superior Extreme Pressure Property as Boundary Lubricants, Sci. Rep., 2015, 5, 12869 CrossRef CAS PubMed.
- X. P. Dai, K. L. Du, Z. Z. Li, H. Sun, Y. Yang, W. Zhang and X. Zhang, Enhanced hydrogen evolution reaction on few-layer MoS2 nanosheets-coated functionalized carbon nanotubes, Int. J. Hydrogen Energy, 2015, 40, 8877–8888 CrossRef CAS.
- L. Liu, Y. W. Chen and P. Huang, Preparation and tribological properties of organically modified graphite oxide in liquid paraffin at ultra-low concentrations, RSC Adv., 2015, 5, 90525–90530 RSC.
- X. H. Zhang, X. H. Huang, M. Q. Xue, X. Ye, W. N. Lei, H. Tang and C. S. Li, Hydrothermal synthesis and characterization of 3D flower-like MoS2 microspheres, Mater. Lett., 2015, 148, 67–70 CrossRef CAS.
- W. Y. Wang, L. Li, K. Wu, G. H. Zhu, S. Tan, W. S. Li and Y. Q. Yang, Hydrothermal synthesis of bimodal mesoporous MoS2 nanosheets and their hydrodeoxygenation properties, RSC Adv., 2015, 5, 61799–61807 RSC.
- M. A. Bissett, I. A. Kinloch and R. A. W. Dryfe, Characterization of MoS2–Graphene Composites for High-Performance Coin Cell Supercapacitors, ACS Appl. Mater. Interfaces, 2015, 7, 17388–17398 CAS.
- X. P. Ren, L. Q. Pang, Y. X. Zhang, X. D. Ren, H. B. Fan and S. Z. Liu, One-step hydrothermal synthesis of monolayer MoS2 quantum dots for highly efficient electrocatalytic hydrogen evolution, J. Mater. Chem. A, 2015, 3, 10693–10697 CAS.
- S. D. Jiang, G. Tang, Z. M. Bai, Y. Y. Wang, Y. Hu and L. Song, Surface functionalization of MoS2 with POSS for enhancing thermal, flame-retardant and mechanical properties in PVA composites, RSC Adv., 2014, 4, 3253–3262 RSC.
- G. B. Feng, A. X. Wei, Y. Zhao and J. Liu, Synthesis of flower-like MoS2 nanosheets microspheres by hydrothermal method, J. Mater. Sci.: Mater. Electron., 2015, 26, 8160–8166 CrossRef CAS.
- X. P. Dai, Z. Z. Li, K. L. Du, H. Sun, Y. Yang, X. Zhang, X. Y. Ma and J. Wang, Facile Synthesis of In situ Nitrogenated Graphene Decorated by Few-Layer MoS2 for Hydrogen Evolution Reaction, Electrochim. Acta, 2015, 171, 72–80 CrossRef CAS.
- A. Ambrosi, Z. Sofer and M. Pumera, Lithium intercalation compound dramatically influences the electrochemical properties of exfoliated MoS2, Small, 2015, 11, 605–612 CrossRef CAS PubMed.
- A. Y. S. Eng, A. Ambrosi, Z. Sofer, P. Simek and M. Pumera, Electrochemistry of transition metal dichalcogenides: strong dependence on the metal-to-chalcogen composition and exfoliation method, ACS Nano, 2014, 8, 12185–12198 CrossRef CAS PubMed.
- J. Kim, S. Byun, A. J. Smith, J. Yu and J. X. Huang, Enhanced electrocatalytic properties of transition-metal dichalcogenides sheets by spontaneous gold nanoparticle decoration, J. Phys. Chem. Lett., 2013, 4, 1227–1232 CrossRef CAS PubMed.
- Y. D. Liu, L. Ren, X. Qi, L. W. Yang, G. L. Hao, J. Li, X. L. Wei and J. X. Zhong, Preparation, characterization and photoelectrochemical property of ultrathin MoS2 nanosheets via hydrothermal intercalation and exfoliation route, J. Alloys Compd., 2013, 571, 37–42 CrossRef CAS.
- J. X. Guo, F. F. Li, Y. F. Sun, X. Zhang and L. Tang, Oxygen-incorporated MoS2 ultrathin nanosheets grown on graphene for efficient electrochemical hydrogen evolution, J. Power Sources, 2015, 291, 195–200 CrossRef CAS.
- N. V. Petrova, I. N. Yakovkin and D. A. Zeze, Metallization and stiffness of the Li-intercalated MoS2 bilayer, Appl. Surf. Sci., 2015, 353, 333–337 CrossRef CAS.
- E. Mieda, R. Azumi, S. Shimada, M. Tanaka, T. Shimizu and A. Ando, Nanoprobe characterization of MoS2 nanosheets fabricated by Li-intercalation, Jpn. J. Appl. Phys., 2015, 54, 08LB07 CrossRef.
- S. H. Song, B. H. Kim, D. H. Choe, J. Kim, D. C. Kim, D. J. Lee, J. M. Kim, K. J. Chang and S. Jeon, Bandgap widening of phase quilted, 2D MoS2 by oxidative intercalation, Adv. Mater., 2015, 27, 3152–3158 CrossRef CAS PubMed.
- X. X. Wang, F. X. Nan, J. L. Zhao, T. Yang, T. Ge and K. Jiao, A label-free ultrasensitive electrochemical DNA sensor based on thin-layer MoS2 nanosheets with high electrochemical activity, Biosens. Bioelectron., 2015, 64, 386–391 CrossRef CAS PubMed.
- J. H. Kim, J. Kim, S. D. Oh, S. Kim and S. H. Choi, Sequential structural and optical evolution of MoS2 by chemical synthesis and exfoliation, J. Korean Phys. Soc., 2015, 66, 1852–1855 CrossRef CAS.
- X. M. Li, W. Z. Wang, L. Zhang, D. Jiang and Y. l. Zheng, Water-exfoliated MoS2 catalyst with enhanced photoelectrochemical activities, Catal. Commun., 2015, 70, 53–57 CrossRef CAS.
- Y. W. Lihui, H. Yu, X. R. Yang, J. J. Zhou, Q. Zhang, Y. Q. Zhang, Z. M. Luo, S. Su and L. H. Wang, Rapid preparation of single-layer transition metal dichalcogenide nanosheets via ultrasonication enhanced lithium intercalation, Chem. Commun., 2016, 52, 529–532 RSC.
- V. Nicolosi, M. Chhowalla, M. G. Kanatzidis, M. S. Strano and J. N. Coleman, Liquid exfoliation of layered materials, Science, 2013, 340, 1226419 CrossRef.
- G. Eda, H. Yamaguchi, D. Voiry, T. Fujita, M. W. Chen and M. Chhowalla, et al., Photoluminescence from chemically exfoliated MoS2, Nano Lett., 2011, 11, 5111–5116 CrossRef CAS PubMed.
- Z. Liu, Y. B. Wang, Z. Y. Wang, Y. G. Yao, J. Q. Dai, S. Das and L. B. Hu, Solvo-thermal microwave-powered two-dimensional material exfoliation, Chem. Commun., 2016, 52, 5757–5760 RSC.
- X. M. Li, W. Z. Wang, L. Zhang, D. Jiang and Y. L. Zheng, Water-exfoliated MoS2 catalyst with enhanced photoelectrochemical activities, Catal. Commun., 2015, 70, 53–57 CrossRef CAS.
- R. K. Joshi, S. Shukla, S. Saxena, G.-H. Lee, V. Sahajwalla and S. Alwarappan, Hydrogen generation via photoelectrochemical water splitting using chemically exfoliated MoS2 layers, AIP Adv., 2016, 6, 015315 CrossRef.
- F. X. Jiang, J. H. Xiong, W. Q. Zhou, C. C. Liu, L. Y. Wang, F. Zhao, H. X. Liu and J. K. Xu, Use of organic solvent-assisted exfoliated MoS2 for optimizing the thermoelectric performance of flexible PEDOT: PSS thin films, J. Mater. Chem. A, 2016, 4, 5265–5273 CAS.
- V. Forsberg, R. Y. Zhang, J. Backstrom, C. Dahlstrom, B. Andres, M. Norgren, M. Andersson, M. Hummelgard and H. Olin, Exfoliated MoS2 in Water without Additives, PLoS One, 2016, 11, 0154522 Search PubMed . (Page 7, Line 7 in the revised paper).
- Y. Gu, X. Zhao, Y. Liu and Y. X. Lv, Preparation and tribological properties of dual-coated TiO2 nanoparticles as water-based lubricant additives, J. Nanomater., 2014, 2014, 2 CrossRef.
- Y. F. Mo, K. T. Turner and I. Szlufarska, Friction laws at the nanoscale, Nature, 2009, 457, 1116–1119 CrossRef CAS PubMed.
|
This journal is © The Royal Society of Chemistry 2016 |