DOI:
10.1039/C6RA23172A
(Paper)
RSC Adv., 2016,
6, 106308-106315
The design, synthesis and biological evaluation of pro-EGCG derivatives as novel anti-vitiligo agents†
Received
17th September 2016
, Accepted 21st October 2016
First published on 26th October 2016
Abstract
With the aim of overcoming the instability and poor membrane permeability of epigallocatechin-3-gallate (EGCG), a series of prodrugs of EGCG and its derivatives (pro-EGCGs) were designed and synthesized, and their protective effect on melanocytes against H2O2-induced cell damage was biologically evaluated. The enhanced potency of pro-EGCGs could be clearly observed, and the most potent compound, 3c, showed excellent protective effect of melanocytes against H2O2-induced cell death, and good effect on lactate dehydrogenase (LDH) release from melanocytes under oxidant stress. In addition, compounds 14b and 14c, which are the parent compounds of 3b and 3c, also showed good inhibitory activities against JAK1, JAK2 and JKA3, which are potential therapeutic targets for anti-vitiligo agents, demonstrating the promising perspective of 3b and 3c as both anti-oxidant and immuno-related agents for anti-vitiligo treatment.
1 Introduction
Vitiligo is a depigmenting skin disease caused by a dermatological disorder of the epidermis and hair follicles, manifesting clinically as expanding hypopigmented lesions of the skin.1 The prevalence of vitiligo is about 1% around the world.2 So far, the pathogenesis of vitiligo is not very clear, but it appears to involve immunologic factors, oxidative stress, sympathetic neurogenic disturbance and other factors.3,4 In particular, the deleterious effects of epidermal free radical (e.g. H2O2) overproduction and total antioxidant status have been extensively demonstrated in lesional and non lesional epidermis, as well as in melanocytes of vitiligo patients,5–10 raising the possibility that antioxidants could be useful to reduce the oxidative stress and the consequent damage to melanocytes.11,12 Moreover, clinical and laboratory findings have also demonstrated the major role of autoimmune factors in the progress of vitiligo.13,14
Epigallocatechin-3-gallate (EGCG) is one of the main chemical constituents of green tea, which has been used as an important traditional Chinese medicine for its biological activities including anti-oxidation, anti-inflammatory, anti-cancer, and neuroprotection.15 In our previous study, we assessed the role of EGCG in treatment of vitiligo induced by monobenzone in mice, demonstrating that EGCG can significantly delay and reduce the prevalence of depigmentation.16 In addition, we also proved that EGCG directly inhibited the kinase activity of Janus kinase 2 (JAK2) to affect the inflammatory signaling pathways. In primary cultured human melanocytes, EGCG pre-treatment attenuated interferon (IFN)-γ-induced phosphorylation of JAK2 and its downstream signal transducer and activator of transcription STAT1 and STAT3.17 Therefore, EGCG is a promising anti-vitiligo agent showing both anti-oxidation activities and immuno-related anti-inflammatory effect.
However, EGCG is notably unstable and is known to have poor bioavailability, owing to the presence of eight unprotected phenolic hydroxyl groups and a metabolically labile ester.18 A synthetic derivative of EGCG, obtained by acylation of EGCG, can act as a prodrug of EGCG (pro-EGCG) with enhanced stability, improved bioavailability, and increased cell membrane penetration.19 As part of our continued interest in the area of vitiligo treatment, herein we investigated the potential of pro-EGCG derivatives, i.e. prodrugs of EGCG and its derivatives (Fig. 1), as novel anti-vitiligo agents. These pro-EGCG derivatives can remarkably protect the melanocytes from H2O2-induced apoptosis via reducing the intercellular reactive oxygen species (ROS) level. Although the anti-oxidant biological activities of EGCG derivatives have been widely studied, to the best of our knowledge this is the first example of a relatively systematic structure–activity relationship (SAR) study for anti-vitiligo activities of a series of EGCG derivatives via a combination of prodrug strategy and structural optimization.
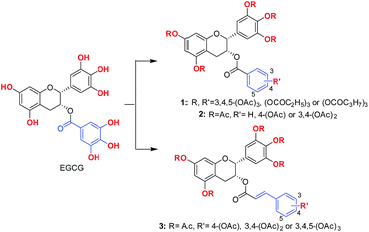 |
| Fig. 1 The structures of designed pro-EGCG derivatives. | |
2 Results and discussion
2.1 The design of pro-EGCG derivatives
Initially, acyl groups with different length were introduced onto the skeleton of EGCG, affording compounds 1a–c (Fig. 1). It was demonstrated that the cellular membrane penetration of 1a–c was increased compared with that of EGCG owing to their remarkably enhanced lipophilicities. Then, adjustment of the number of hydroxyl groups on the galloyl moiety of EGCG was considered as well as the introduction of the hydroxy-cinnamoyl moiety into EGCG, affording six pro-EGCG derivatives 2a–c and 3a–c (Fig. 1).
2.2 The synthesis of pro-EGCG derivatives
EGCG was chosen as the starting material since it is the most abundant catechin from green tea and commercially available. First, direct acylation of EGCG by use of appropriate acid anhydrides afforded acylated EGCG derivatives 1a–c. Then, benzylation of all of the hydroxyl groups of EGCG in the presence of benzyl bromide (BnBr) and potassium carbonate in anhydrous dimethylformamide (DMF) gave perbenzylated EGCG, 4, which was further alkaline hydrolyzed to afford pentabenzylated epigallocatechin, 5. Subsequently, esterification of 5 using various hydroxyl-substituted benzoyl chlorides afforded compounds 6a–c. Further hydrogenolysis of 6a–c using Pd/C at room temperature provided hydroxyl-substituted benzoates of EGC, 7a–c respectively. Then, acylation of 7a–c with acetic anhydride afforded 2a–c. In addition, the preparation of the hydroxyl-substituted caffeate of the 3-OH of EGC started with the protection of the hydroxyl groups of EGCG by tert-butyldimethylsilyl chloride (TBDMSCl) in anhydrous dimethylformamide (DMF) in the presence of imidazole at room temperature, affording key intermediate 8. Then reduction of 8 using LiAlH4 in tetrahydrofuran (THF) gave 5TBS-epigallocatechin, 9. In addition, TBS-protected hydroxyl-substituted caffeic acids 12 were obtained by TBS protection and subsequent Knoevenagel condensation from hydroxyl-substituted benzaldehydes 10. Furthermore, condensation reaction of 9 with various TBS-protected hydroxyl-substituted caffeic acids afforded compound 13. Next, deprotection of the TBS protecting group of 13 by use of HF–pyridine (HF–Py) provided 14. Finally, acyl EGCG derivatives 3a–c were obtained by direct acylation reaction of 14 (Scheme 1).
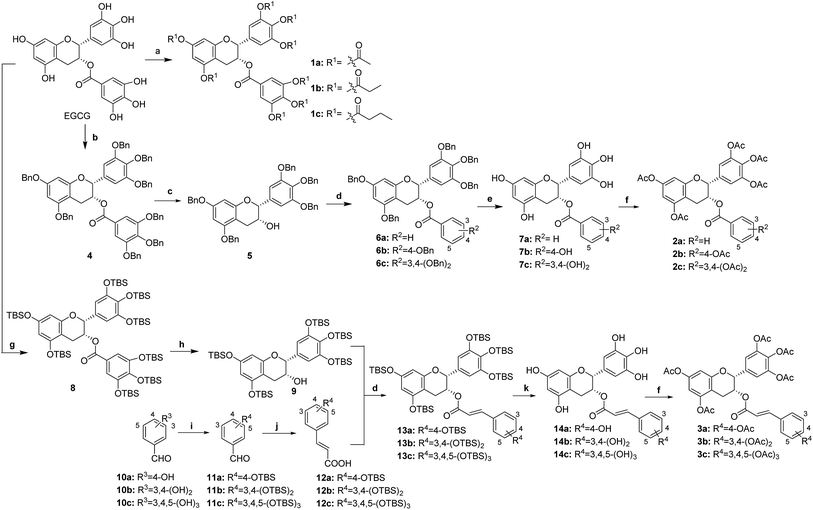 |
| Scheme 1 Synthesis of hydroxyl-substituted benzoates of EGCG. Reagents and conditions: (a) appropriate acid anhydride, EtOAc, TEA; (b) BnBr, KOH, EtOH/H2O; (c) K2CO3, MeOH; (d) appropriate benzoyl chloride, DMAP; (e) Pd/C, H2; (f) Ac2O, DMAP; (g) TBDMSCl, imidazole, DMF; (h) LiAlH4, THF; (i) DCC, DMAP, CH2Cl2; (j) pyridine, piperidine, malonic acid; (k) HF–Py complex, THF. | |
2.3 Pro-EGCG derivatives protected melanocytes from H2O2-induced cell damage
It was investigated whether these synthesized pro-EGCG derivatives protect melanocytes from H2O2-induced cell death, which can mimic the oxidative damage of the physiological vitiligo state. An in vitro screening model was used for evaluating the active anti-vitiligo agents.20 In this model, prior to treatment with 1 mM H2O2, the melanocytes were treated with EGCG or pro-EGCG derivatives for 1 hour. Subsequently, the culture solution was removed to eliminate the effect of residual compounds in the culture. As shown in Fig. 2, the survival of melanocytes in the negative control group (0.1% DMSO) was remarkably decreased, while the survival of melanocytes in the EGCG group was moderate in a dose-dependent manner, ranging from 36.2% to 67.6%, which would be caused by the low membrane penetration ability of EGCG. To our delight, the protective effect of pro-EGCG with full acyl protection was significantly improved compared with that of EGCG, maybe due to the increased cellular drug concentration in the prodrug strategy. In particular, peracetylated EGCG derivative 1a exhibited the most potent protecting capacity for melanocytes against H2O2-induced oxidative stress, and the survival of melanocytes in the presence of 1a (40 μM) reached up to 91%. In addition, as the length of acyl group attached to EGCG was further increased, as exemplified in compounds 1b and 1c, the potency of the protecting capacity decreased. Next, the number of hydroxy groups on the gallic acid moiety of EGCG derivatives was reduced to investigate the effect on their capacity to protect melanocytes from H2O2-induced oxidative stress. Obviously, the potency of their protecting capacity was significantly related with the number of gallic acid moieties; indeed, compounds 2a–c only showed moderate activities at a concentration of 10 μM and 20 μM, while the high dose (40 μM) group of 2a and 2c showed promising activities, which were better than that of EGCG.
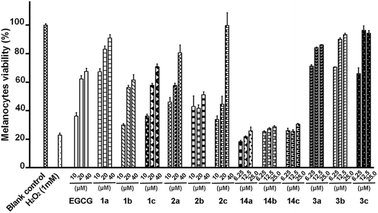 |
| Fig. 2 The protective effect of EGCG and pro-EGCG derivatives by measurement of melanocyte viability under H2O2-induced cell apoptosis. Compounds 1a–c protect cells from H2O2-induced cell death. | |
Furthermore, we replaced gallic acid with caffeic acid derivatives. The resulting peracetylated compounds, 3a–c, exhibited promising activities in a dose-dependent manner. Of interest, all of these three compounds at a concentration of 25 μM showed excellent protective effect of melanocytes from H2O2-induced cell death with almost 100% cell viabilities, significantly more potent than that of their parent compounds 14a–c and peracetylated EGCG derivative 1a. It is indicated that the promising activities of 3a–c would also be caused by the diverse and potent biological properties of caffeic acid, including anti-inflammatory activity, anti-oxidative activity, and so on.21
The cytotoxic activities of these pro-EGCG derivatives were also evaluated to ensure their non-toxicity at the biological assay concentration. Obviously, all of the compounds exhibited a non-cytotoxic effect against melanocytes, demonstrating their excellent safe profiles (Table S1†).
2.4 Lactate dehydrogenase (LDH) release assay
With the aim of explaining the mechanism of the protection action of 3c, the most potent compound, the amount of LDH release was analysed, because of the important role of LDH in cell destruction under oxidant stress.22 As shown in Table 1, the percentage release of total LDH in the blank control group was 7.58%, which is very low under normal physiological conditions, while the amount of LDH released was significantly increased to 47.19% on exposure to 1 mM H2O2. As expected, H2O2-induced leakage of LDH can be remarkably diminished in a dose-dependent manner by the pretreatment of melanocytes with EGCG or 3c; in fact, the potency of 3c is much higher than that of EGCG, which is consistent with their protective effect against H2O2-induced cell damage of melanocytes.
Table 1 The effect of pro-EGCG 3c on lactate dehydrogenase (LDH) release from melanocytes under oxidant stress
Compd |
Concentration (μM) |
LDH release (% of total LDH) |
Prior to exposure to 1 mM H2O2, melanocytes were pre-treated with the indicated compound for 1 hour. |
Blank |
— |
7.58 |
H2O2 |
— |
47.19 |
EGCGa |
6.25 |
45.27 |
12.5 |
39.30 |
25 |
34.33 |
3ca |
6.25 |
21.99 |
12.5 |
15.37 |
25 |
13.17 |
2.5 Inhibition of JAK kinase assays
In addition to the anti-oxidant properties of pro-EGCG derivatives, we also paid close attention to another potential therapeutic target (Janus kinases, JAKs) for anti-vitiligo.23 We anticipated that the excellent protective effect on melanocytes was also related to the inhibition of Janus kinases (JAKs). To our delight, compounds 14b and 14c, which are the parent drugs of compounds 3b and 3c, respectively, showed good inhibitory activities against all JAK subtypes, JAK1, JAK2, and JAK3, with IC50 at micromolar concentrations (Table 2). Of interest, both compounds 14b and 14c showed promising selective profiles against different JAKs, for example, the selectivity of 14c for JAK3 as against JAK1 and JAK2 was 45.2- and 107-fold, respectively. Compounds 3b and 3c would be hydrolyzed in the endochylema to release 14b and 14c, resulting in the blockade of the JAKs pathway which is an important immune-related signal pathway for the development of vitiligo.
Table 2 The inhibitory activities of compounds 14b and 14c against JAKs
Compd |
IC50 (μM) |
JAK1 |
JAK2 |
JAK3 |
14b |
30.2 |
15.4 |
1.7 |
14c |
53.5 |
22.6 |
0.5 |
3 Conclusion
In summary, prodrug strategy was investigated to overcome the instability and poor membrane permeability of EGCG. Several key structural factors in EGCG were considered in our study and their relative structure–activity relationships (SAR) were also explored systematically. Nine pro-EGCGs were synthesized and biologically evaluated; the potency of these compounds in protecting melanocytes from H2O2-induced cell death can be clearly observed, and some of them showed significantly improved activities compared with that of EGCG. A preliminary study of the protection mechanism of compound 3c against oxidant-stress-caused cell damage to melanocytes was carried out, which suggested that compound 3c can remarkably diminish H2O2-induced leakage of LDH. In addition to the anti-oxidant properties of pro-EGCG derivatives, compounds 14b and 14c, the parent compounds of 3b and 3c, showed good inhibitory activities against JAKs which are another potential immuno-related therapeutic target for anti-vitiligo, demonstrating the promising perspective of 3b and 3c as both anti-oxidant and immuno-related agents for anti-vitiligo treatment.
4 Experimental section
4.1 Chemistry
Proton NMR spectra were obtained on a Bruker AVII 500 or 400 NMR spectrometer (500 or 400 MHz) with use of CDCl3, CD3OD, or DMSO-d6 as solvent. Carbon-13 NMR spectra were obtained on a Bruker spectrometer (125 MHz) by use of CDCl3, CD3OD or DMSO-d6 as solvent. Carbon-13 chemical shifts were referenced to the center peak of CDCl3 (d 77.0 ppm) or DMSO-d6 (d 39.5 ppm). Multiplicities are recorded by the following abbreviations: s, singlet; d, double; t, triplet; q, quartet; m, multiplet; J, coupling constant (Hz). Electrospray ionization mass spectroscopy (ESI-MS) spectra were obtained with a Shimadzu LCMS-2020 mass spectrometer. (−)-Epigallocatechin-3-gallate (EGCG) was purchased from Nanjing Guangrun Biotechnology Co., Ltd. All the fine chemicals were obtained from commercial suppliers and used without purification.
4.2 The synthesis of (2R,3R)-5,7-diacetoxy-2-(3,4,5-triacetoxyphenyl)chroman-3-yl-3,4,5-triacetoxybenzoate (1a)
Triethylamine (TEA, 2.2 g, 21.8 mmol) and acetic anhydride (2.2 g, 21.8 mmol) were added to a solution of EGCG (1 g, 2.18 mmol) in EtOAc in an ice bath, and the mixture was stirred for 4 hours. The reaction was quenched with water, extracted with EtOAc and then washed with Na2CO3(aq). The organic layer was concentrated under reduced pressure and the residue was purified by column chromatography (ethyl acetate
:
petroleum ether (EA
:
PE) = 1
:
1) to provide compound 1a as a white solid. Analytical data: yield 90%; 1H NMR (500 MHz, CDCl3) δ 7.62 (s, 2H), 7.23 (s, 2H), 6.73 (d, J = 2.0 Hz, 1H), 6.60 (d, J = 2.0 Hz, 1H), 5.63 (d, J = 1.5 Hz, 1H), 5.17 (s, 1H), 3.02 (m, 2H), 2.31–2.22 (m, 24H). The data are consistent with those in the literature.24
4.3 The identical method to that for 1a was used to synthesize and purify (2R,3R)-5,7-dipropionyloxy-2-(3,4,5-tripropionyloxyphenyl)chroman-3-yl-3,4,5-tripropionyloxybenzoate (1b) as a white solid
Analytical data: yield 75%; 1H NMR (500 MHz, CDCl3) δ 7.63 (s, 2H), 7.25 (s, 2H), 6.76 (d, J = 2.0 Hz, 1H), 6.64 (d, J = 2.0 Hz, 1H), 5.71–5.66 (m, 1H), 5.20 (s, 1H), 3.04 (m, 2H), 2.61–2.50 (m, 16H), 1.29–1.21 (m, 24H). The data are consistent with those in the literature.24
4.4 The identical method to that for 1a was used to synthesize and purify (2R,3R)-5,7-dibutyryloxy-2-(3,4,5-tributyryloxyphenyl)chroman-3-yl-3,4,5-tributyryloxybenzoate (1c) as a white solid
Analytical data: yield 80%; 1H NMR (400 MHz, CDCl3) δ 7.59 (s, 2H), 7.21 (s, 2H), 6.73 (d, J = 2.2 Hz, 1H), 6.60 (d, J = 2.2 Hz, 1H), 5.67 (s, 1H), 5.18 (s, 1H), 3.02 (m, 2H), 2.70–2.28 (m, 16H), 1.96–1.64 (m, 16H), 1.13–0.81 (m, 24H). The data are consistent with those in the literature.24
4.5 The synthesis of (2R,3R)-5,7-bis(benzyloxy)-2-(3,4,5-tris(benzyloxy)phenyl)chroman-3-yl-3,4,5-tris(benzyloxy)benzoate (4)
EGCG (2 g, 4.3 mmol) was dissolved in anhydrous dimethyl formamide (DMF) (30 mL). Potassium carbonate (12 g, 87 mmol) and benzyl bromide (6.2 mL, 52.4 mmol) were added successively. The mixture was stirred at room temperature (rt) for 48 h. The reaction mixture was added to water and extracted with EtOAc. The organic layer was further washed with water and brine, then dried with anhydrous Na2SO4, and evaporated. Finally, the residue was purified by flash column chromatography, and recrystallized in petroleum ether–EtOAc (10
:
1) to afford 4 as a white solid. Analytical data: yield 45%; 1H NMR (500 MHz, CDCl3) δ 7.48–7.18 (m, 42H), 6.77 (d, J = 9.5 Hz, 2H), 6.43 (d, J = 2.0 Hz, 1H), 6.38 (d, J = 2.0 Hz, 1H), 5.70 (s, 1H), 5.12–4.66 (m, 17H), 3.16 (dd, J = 17.5, 4.5 Hz, 1H), 3.10 (dd, J = 17.5, 2.0 Hz, 1H). The data are consistent with those in the literature.25
4.6 The synthesis of (2R,3R)-5,7-bis(benzyloxy)-2-(3,4,5-tris(benzyloxy)phenyl)chroman-3-ol (5)
Compound 4 (500 mg, 0.4 mmol) was dissolved in a solution of methanol (5 mL) and ethylene glycol dimethyl ether (5 mL), and then potassium carbonate (193 mg, 1.4 mmol) was added. The resulting mixture was stirred at rt for 5 h until thin layer chromatography (TLC) showed the reaction had been completed. Then the solvent was evaporated, and the residue was purified by flash chromatography on silica gel to afford compound 5 as a viscous liquid. Analytical data: yield 85%; 1H NMR (400 MHz, CDCl3) δ 7.58–7.17 (m, 25H), 6.81 (s, 2H), 6.29 (s, 2H), 5.22–4.86 (m, 10H), 4.25 (d, J = 29.8 Hz, 1H), 3.02 (d, J = 17.2 Hz, 1H), 2.93 (dd, J = 17.2, 3.8 Hz, 1H), 1.68 (d, J = 5.2 Hz, 1H). The data are consistent with those in the literature.25
4.7 The synthesis of (2R,3R)-5,7-dihydroxy-2-(3,4,5-trihydroxyphenyl)chroman-3-yl benzoate (7a)
Benzoylchloride (240 mg, 0.174 mmol) was dissolved in dry CH2Cl2 (10 mL) and added dropwise into a solution of compound 5 (100 mg, 0.116 mmol) and dimethylaminopyridine (DMAP) (35.36 mg, 0.28 mmol) in CH2Cl2 (10 mL) at 0 °C. The mixture was stirred at rt overnight, the solvent was then evaporated, and the resulting mixture was extracted with EtOAc, and washed with water. The organic layer was dried using anhydrous Na2SO4, and evaporated. The crude product 6a was used directly in the next step without further purification. Then, under a hydrogen atmosphere, 10% Pd/C (150 mg) was added to a solution of the above-obtained compound 6a in ethyl acetate (10 mL). The resulting mixture was stirred at rt overnight. Then the mixture was evaporated to remove the solvent in vacuum. The residue was purified by flash column chromatograph to afford 7a as a yellow solid. Analytical data: yield 82%; 1H NMR (400 MHz, DMSO-d6) δ 9.29 (s, 1H), 9.05 (s, 1H), 8.77 (s, 2H), 7.99 (s, 1H), 7.80 (d, J = 8.0 Hz, 2H), 7.61 (t, J = 7.6 Hz, 1H), 7.47 (t, J = 7.6 Hz, 2H), 6.44 (s, 2H), 5.92 (s, 1H), 5.84 (s, 1H), 5.41 (s, 1H), 5.02 (s, 1H), 2.99 (dd, J = 17.4, 4.2 Hz, 1H), 2.75 (d, J = 16.8 Hz, 1H); 13C NMR (125 MHz, CD3OD) δ 166.0, 156.6, 156.5, 155.8, 145.4, 132.7, 132.3, 130.0, 129.4, 129.2, 128.1, 105.3, 97.8, 95.1, 94.4, 77.1, 69.4, 60.2, 25.3, 19.5, 13.1; electrospray ionization mass spectrometry (ESI-MS) m/z 411 (M + H)+.
4.8 The identical method to that for 7a was used to synthesize and purify (2R,3R)-5,7-dihydroxy-2-(3,4,5-trihydroxyphenyl)chroman-3-yl 4-hydroxybenzoate (7b)
Analytical data: yield 76%; 1H NMR (500 MHz, DMSO-d6) δ 10.30 (s, 1H), 9.28 (s, 1H), 9.05 (s, 1H), 8.77 (s, 2H), 8.00 (s, 1H), 7.67 (d, J = 8.5 Hz, 2H), 6.80 (d, J = 8.5 Hz, 2H), 6.44 (s, 2H), 5.88 (m, 2H), 5.32 (s, 1H), 5.00 (s, 1H), 2.95 (dd, J = 17.0, 4.5 Hz, 1H), 2.72 (d, J = 15.5 Hz, 1H); 13C NMR (125 MHz, CD3OD) δ 166.1, 162.0, 156.5, 156.4, 155.8, 145.3, 132.3, 131.5, 129.4, 120.9, 114.7, 105.3, 97.9, 95.1, 94.4, 77.1, 68.8, 48.5, 25.3; ESI-MS m/z 427 (M + H)+.
4.9 The identical method to that for 7a was used to synthesize and purify (2R,3R)-5,7-dihydroxy-2-(3,4,5-trihydroxyphenyl)chroman-3-yl 3,4-dihydroxybenzoate (7c)
Analytical data: yield 69%; 1H NMR (500 MHz, CD3OD) δ 7.34 (d, J = 1.5 Hz, 2H), 6.80–6.71 (m, 1H), 6.53 (d, J = 4.5 Hz, 2H), 5.98 (d, J = 3.0 Hz, 2H), 5.53 (dd, J = 9.0, 7.5 Hz, 1H), 5.00 (s, 1H), 3.01 (dd, J = 17.0, 4.5 Hz, 1H), 2.88 (dd, J = 17.5, 2.5 Hz, 1H); 13C NMR (125 MHz, CD3OD) δ 166.2, 156.5, 155.8, 150.3, 145.3, 144.5, 132.4, 129.4, 122.6, 121.3, 116.1, 114.5, 105.4, 98.0, 95.1, 94.4, 77.2, 68.7, 60.2, 25.4, 19.5, 13.1; ESI-MS m/z 443 (M + H)+.
4.10 The identical method to that for 1a was used to synthesize and purify 5-((2R,3R)-5,7-diacetoxy-3-(benzoyloxy)chroman-2-yl)benzene-1,2,3-triyl triacetate (2a)
Analytical data: yield 76%;1 H NMR (400 MHz, DMSO-d6) δ 7.71 (t, J = 11.4 Hz, 2H), 7.56 (m, 1H), 7.56–7.34 (m, 3H), 7.37–7.07 (m, 1H), 6.91–6.59 (m, 2H), 5.76–5.38 (m, 2H), 3.15 (dd, J = 17.6, 4.0 Hz, 1H), 2.94 (d, J = 17.6 Hz, 1H), 2.65–2.06 (m, 15H).
4.11 The identical method to that for 1a was used to synthesize and purify 5-((2R,3R)-5,7-diacetoxy-3-((4-acetoxybenzoyl)oxy)chroman-2-yl)benzene-1,2,3-triyl triacetate (2b)
Analytical data: yield 83%; 1H NMR (500 MHz, DMSO-d6) δ 7.86–7.73 (m, 2H), 7.41 (s, 2H), 7.28–7.16 (m, 2H), 6.73 (dd, J = 74.0, 2.0 Hz, 2H), 5.61 (d, J = 40.5 Hz, 2H), 3.16 (dd, J = 17.5, 4.0 Hz, 1H), 2.95 (d, J = 16.5 Hz, 1H), 2.39–2.15 (m, 18H). The data are consistent with those in the literature.26
4.12 The identical method to that for 1a was used to synthesize and purify 5-((2R,3R)-5,7-diacetoxy-3-((3,4-diacetoxybenzoyl)oxy)chroman-2-yl)benzene-1,2,3-triyl triacetate (2c)
Analytical data: yield 90%; 1H NMR (500 MHz, DMSO-d6) δ 7.67 (dd, J = 8.5, 1.5 Hz, 1H), 7.61 (d, J = 1.5 Hz, 1H), 7.41 (s, 2H), 7.36 (d, J = 8.5 Hz, 1H), 6.80 (d, J = 2.0 Hz, 1H), 6.68 (t, J = 13.5 Hz, 1H), 5.64 (s, 1H), 5.56 (s, 1H), 3.16 (dd, J = 17.5, 4.0 Hz, 1H), 2.96 (d, J = 17.5 Hz, 1H), 2.47–2.03 (m, 21H).
4.13 The synthesis of (−)-epigallocatechin-3-gallate octa(tert-butyldimethylsilyl) ether (8)
To a solution of EGCG (20.0 g, 43.6 mmol, 1.0 equiv.) in dry DMF (100 mL) was added imidazole (29.8 g, 438 mmol) followed by TBDMSCl (65.8 g, 436 mmol, 10 equiv.) at 0 °C. The solution was stirred overnight at room temperature. The reaction mixture was quenched with water (100 mL) and extracted with hexane (100 mL × 3). The combined organic layers were washed with water (50 mL × 3) and saturated aqueous NaCl (50 mL × 3), dried over NaSO4(s), filtered, and concentrated under reduced pressure to provide crude white compound 8, which was used directly for the next step.
4.14 The synthesis of (−)-epigallocatechin penta(tert-butyldimethylsilyl) ether (9)
To (−)-epigallocatechin-3-gallate octa(tert-butyldimethylsilyl) ether (8, 13.0 g, 9.4 mmol) in THF (100 mL) was slowly added LiAlH4 (0.72 g, 18.9 mmol) at 0 °C. The solution was warmed to room temperature and stirred for 3 hours. The reaction was quenched with water in an ice bath and filtered. The cake was washed with EtOAc (50 mL) and the combined filtrates were condensed under reduced pressure. The residue was purified by column chromatography (1.0% EtOAc in petroleum ether) to provide (−)-epigallocatechin penta(tert-butyldimethylsilyl) ether, 9, as a white solid. Analytical data: yield 80%. 1H NMR (500 MHz, CDCl3) δ 6.65 (s, 2H), 6.15 (d, J = 2.0 Hz, 1H), 6.01 (d, J = 2.0 Hz, 1H), 4.88 (s, 1H), 4.19 (d, J = 29.5 Hz, 1H), 2.88 (qd, J = 16.5, 4.0 Hz, 2H), 1.04 (d, J = 1.5 Hz, 18H), 1.02–1.00 (m, 9H), 0.97 (s, 18H), 0.29–0.26 (m, 6H), 0.24 (s, 12H), 0.23 (s, 6H), 0.16 (t, J = 3.5 Hz, 6H). The data are consistent with those in the literature.15
4.15 The identical method to that for 8 was used to synthesize and purify 4-((tert-butyldimethylsilyl)oxy) benzaldehyde (11a)
Analytical data: yield 75%; 1H NMR (500 MHz, CDCl3) δ 9.91 (s, 1H), 7.98–7.65 (m, 2H), 7.12–6.79 (m, 2H), 1.02–1.01 (m, 9H), 0.28–0.26 (m, 6H). The data are consistent with those in the literature.27
4.16 The identical method to that for 8 was used to synthesize and purify 3,4-bis((tert-butyldimethylsilyl)oxy) benzaldehyde (11b)
Analytical data: yield 82%; 1H NMR (500 MHz, CDCl3) δ 9.83 (s, 1H), 7.39 (q, J = 1.5 Hz, 2H), 6.97 (d, J = 7.5 Hz, 1H), 1.02 (d, J = 1.5 Hz, 18H), 0.31–0.19 (m, 12H). The data are consistent with those in the literature.27
4.17 The identical method to that for 8 was used to synthesize and purify 3,4,5-tris((tert-butyldimethylsilyl)oxy)benzaldehyde (11c)
Analytical data: yield 87%; 1H NMR (500 MHz, CDCl3) δ 9.75 (s, 1H), 7.06 (d, J = 11.5 Hz, 2H), 1.02–0.96 (m, 27H), 0.15–0.07 (m, 18H). The data are consistent with those in the literature.28
4.18 The synthesis of (E)-3-(4-((tert-butyldimethylsilyl)oxy)phenyl)acrylic acid (12a)
To 20 mL of pyridine containing 2 drops of piperidine was added 4-((tert-butyldimethylsilyl)oxy)benzaldehyde (11a, 3 g, 12.7 mmol) and malonic acid (2.6 g, 25.4 mmol). The mixture was heated at 85 °C for 5 hours, cooled to room temperature, and stirred overnight. The reaction mixture was quenched with 2 N HCl (100 mL) and extracted with EtOAc (100 mL × 3). The combined the residue was purified by column chromatography (dichloromethane
:
MeOH organic layers were washed with water and brine, dried over NaSO4(s), filtered, and concentrated under reduced pressure. = 10
:
1) to provide 12a as a white solid. Analytical data: yield 37%; 1H NMR (500 MHz, CDCl3) δ 7.74 (t, J = 17.0 Hz, 1H), 7.52–7.41 (m, 2H), 6.92–6.84 (m, 2H), 6.32 (t, J = 16.0 Hz, 1H), 1.01 (d, J = 2.0 Hz, 9H), 0.27–0.23 (m, 6H); ESI-MS m/z 279 (M − H)−
4.19 The identical method to that for 12a was used to synthesize and purify (E)-3-(3,4-bis((tert-butyldimethysilyl)oxy)phenyl)acrylic acid (12b)
Analytical data: yield of 51%. 1H NMR (500 MHz, CDCl3) δ 7.69 (d, J = 15.5 Hz, 1H), 7.07 (dd, J = 7.0, 1.5 Hz, 2H), 6.92–6.81 (m, 1H), 6.26 (t, J = 11.5 Hz, 1H), 1.03–1.01 (m, 18H), 0.25 (d, J = 4.5 Hz, 12H). ESI-MS m/z 409 (M − H)−.
4.20 The identical method to that for 12a was used to synthesize and purify (E)-3-(3,4,5-tris((tert-butyl dimethylsilyl)oxy)phenyl)acrylic acid (12c)
Analytical data: yield 43%; 1H NMR (500 MHz, CDCl3) δ 7.61 (d, J = 15.5 Hz, 1H), 6.74 (s, 2H), 6.21 (d, J = 15.5 Hz, 1H), 1.07–0.91 (m, 27H), 0.27–0.14 (m, 18H); ESI-MS m/z 539 (M − H)−.
4.21 The synthesis of (2R,3R)-5,7-di((tert-butyldimethysilyl)oxy)-2-(3,4,5-tri((tert-butyldimethylsilyl)oxy)phenyl)chroman-3-yl (E)-3-(4-((tert-butyldimethylsilyl)oxy)phenyl)acrylate (13a)
To a solution of compound 12a (1.0 equiv.), N,N′-dicyclohexylcarbodiimide (DCC, 2.2 equiv.), and 4-dimethylaminopyridine (DMAP, 2.2 equiv.) in dry CH2Cl2 was added with hydroxyl-substituted caffeic acid (2.0 equiv.) at 0 °C. Then the solution was stirred at room temperature for 3 h, the insoluble urea was filtered, and the filtrate was concentrated under reduced pressure. The residue was purified by column chromatography (1.0% EtOAc in petroleum ether) to provide 13a as a white solid. Analytical data for 13a: yield 40%; 1H NMR (500 MHz, CDCl3) δ 7.53–7.47 (m, 1H), 7.35 (t, J = 5.5 Hz, 2H), 6.84–6.80 (m, 2H), 6.62 (s, 2H), 6.23–6.17 (m, 2H), 6.00 (t, J = 2.5 Hz, 1H), 5.57–5.51 (m, 1H), 5.02 (s, 1H), 1.02–0.91 (m, 54H), 0.25–0.09 (m, 36H).
4.22 The identical method to that for 13a was used to synthesize and purify (2R,3R)-5,7-di((tert-butyldimethylsilyl)oxy)-2-(3,4,5-tri((tert-butyldimethylsilyl)oxy)phenyl)chroman-3-yl (E)-3-(3,4-di((tert-butyldimethylsilyl)oxy)phenyl)acrylate (13b)
Analytical data for 13b: yield 53%; 1H NMR (500 MHz, CDCl3) δ 7.43 (d, J = 15.5 Hz, 1H), 7.01–6.88 (m, 2H), 6.83–6.75 (m, 1H), 6.66–6.57 (m, 2H), 6.18 (t, J = 7.5 Hz, 1H), 6.12 (d, J = 15.5 Hz, 1H), 5.99 (dd, J = 7.5, 2.0 Hz, 1H), 5.55 (s, 1H), 5.01 (s, 1H), 2.95 (ddd, J = 20.5, 17.5, 4.0 Hz, 2H), 1.02–0.88 (m, 63H), 0.30–0.06 (m, 42H).
4.23 The identical method to that for 13a was used to synthesize and purify (2R,3R)-5,7-di((tert-butyldimethylsilyl)oxy)-2-(3,4,5-tri((tert-butyldimethylsilyl)oxy)phenyl)chroman-3-yl (E)-3-(3,4,5-tri((tert-butyldimethylsilyl)oxy)phenyl)acrylate (13c)
Analytical data for 13c: yield 65%; 1H NMR (500 MHz, CDCl3) δ 7.36 (d, J = 15.5 Hz, 1H), 7.17 (s, 1H), 6.62 (s, 1H), 6.61 (s, 1H), 6.20 (d, J = 2.0 Hz, 1H), 6.06 (d, J = 15.5 Hz, 1H), 6.00 (d, J = 2.0 Hz, 1H), 5.66 (d, J = 13.0 Hz, 1H), 5.58 (s, 1H), 5.43 (s, 1H), 2.99–2.90 (m, 2H), 1.04–0.86 (m, 72H), 0.26–0.08 (m, 48H).
4.24 The synthesis of (2R,3R)-5,7-dihydroxy-2-(3,4,5-trihydroxyphenyl)chroman-3-yl (E)-3-(4-hydroxyphenyl) acrylate (14a)
To a solution of compound 13a (100 mg, 0.088 mmol) in a plastic beaker was added HF–Py complex (60 equiv.) and Py (60 equiv.). The reaction was stirred at room temperature. After the reaction was completed, the solution was quenched with 1 N HCl and extracted with EtOAc. The combined organic layer was concentrated under reduced pressure and the residue was purified by column chromatography (DCM
:
MeOH = 10
:
1) to provide compound 14a as a yellow solid. Analytical data: yield 56%; 1H NMR (500 MHz, CD3OD) δ 7.51 (d, J = 16.0 Hz, 1H), 7.46–7.38 (m, 2H), 6.82–6.71 (m, 2H), 6.56–6.48 (m, 2H), 6.27 (d, J = 16.0 Hz, 1H), 5.97 (dd, J = 8.5, 2.5 Hz, 2H), 5.52–5.43 (m, 1H), 4.96 (s, 1H), 2.98 (dd, J = 17.0, 4.5 Hz, 1H), 2.85 (dd, J = 17.0, 2.5 Hz, 1H); 13C NMR (125 MHz, CD3OD) δ 171.6, 167.2, 159.7, 156.5, 156.4, 155.7, 145.4, 145.3, 129.8, 129.4, 125.9, 115.3, 113.9, 105.5, 98.0, 95.2, 94.4, 77.1, 68.4, 60.1, 25.3, 19.4, 13.1; ESI-MS m/z 453 (M + H)+.
4.25 The identical method to that for 14a was used to synthesize and purify (2R,3R)-5,7-dihydroxy-2-(3,4,5-trihydroxyphenyl)chroman-3-yl (E)-3-(3,4-dihydroxyphenyl)acrylate (14b)
Analytical data: yield 68%; 1H NMR (500 MHz, CD3OD) 1H NMR (500 MHz, CD3OD) δ 7.44 (d, J = 16.0 Hz, 1H), 7.01 (d, J = 2.0 Hz, 1H), 6.92 (dd, J = 8.0, 2.0 Hz, 1H), 6.76 (d, J = 8.0 Hz, 1H), 6.52 (s, 2H), 6.20 (d, J = 16.0 Hz, 1H), 5.98 (dd, J = 8.0, 2.0 Hz, 2H), 5.52–5.40 (m, 1H), 4.96 (s, 1H), 2.98 (dd, J = 17.0, 4.5 Hz, 1H), 2.84 (dd, J = 17.0, 2.5 Hz, 1H); 13C NMR (125 MHz, CD3OD) δ 167.2, 156.5, 156.4, 155.7, 148.1, 145.8, 145.3, 132.4, 129.3, 126.4, 121.6, 115.0, 113.8, 113.7, 105.4, 97.9, 95.1, 94.4, 77.1, 68.4, 60.1, 25.3, 19.4, 13.1; ESI-MS m/z 469 (M + H)+.
4.26 The identical method to that for 14a was used to synthesize and purify (2R,3R)-5,7-dihydroxy-2-(3,4,5-trihydroxyphenyl)chroman-3-yl (E)-3-(3,4,5-trihydroxyphenyl)acrylate (14c)
Analytical data: yield 67%; 1H NMR (500 MHz, CD3OD) δ 7.36 (d, J = 15.5 Hz, 1H), 6.55 (m, 4H), 6.16 (d, J = 15.5 Hz, 1H), 5.52–5.43 (m, 1H), 4.96 (s, 1H), 2.98 (dd, J = 17.0, 4.5 Hz, 1H), 2.84 (dd, J = 17.0, 2.5 Hz, 1H); 13C NMR (125 MHz, CD3OD) δ 171.7, 167.3, 156.5, 156.4, 155.7, 146.1, 145.6, 145.4, 136.1, 132.4, 129.3, 125.4, 113.9, 107.3, 105.5, 98.0, 77.0, 68.4, 60.1, 25.1, 19.4, 12.9; ESI-MS m/z 485 (M + H)+.
4.27 The synthesis of 5-((2R,3R)-5,7-diacetoxy-3-(((E)-3-(4-acetoxyphenyl)acryloyl)oxy)chroman-2-yl)benzene-1,2,3-triyl triacetate (3a)
To a solution of 14a (20 mg, 0.044 mmol) in EtOAc were added DMAP (27 mg, 0.22 mmol) and acetic anhydride (36 mg, 0.35 mmol) in an ice bath and stirred for 4.0 h. The reaction was quenched with water and Na2CO3(aq) in an ice bath and extracted with EtOAc. The combined organic layer was concentrated under reduced pressure and the residue was purified by column chromatography (EA
:
PE = 1
:
1) to provide compound 3a as a white solid. Analytical data: yield 69%; 1H NMR (500 MHz, CDCl3) δ 7.55 (dd, J = 12.5, 6.5 Hz, 3H), 7.29–7.28 (m, 2H), 7.11 (d, J = 8.5 Hz, 2H), 6.73 (d, J = 2.0 Hz, 1H), 6.61 (d, J = 2.0 Hz, 1H), 6.32 (d, J = 16.0 Hz, 1H), 5.55 (s, 1H), 5.19 (s, 1H), 3.01 (d, J = 20.0 Hz, 2H), 2.41–2.15 (m, 18H).
4.28 The identical method to that for 14a was used to synthesize and purify 5-((2R,3R)-5,7-diacetoxy-3-(((E)-3-(3,4-diacetoxyphenyl) acryloyl)oxy)chroman-2-yl)benzene-1,2,3-triyl triacetate (3b)
Analytical data: yield 78%; 1H NMR (500 MHz, CDCl3) δ 7.53–7.48 (m, 1H), 7.41 (dd, J = 8.5, 2.0 Hz, 1H), 7.37 (d, J = 2.0 Hz, 1H), 7.28 (s, 2H), 7.21 (t, J = 5.5 Hz, 1H), 6.73 (d, J = 2.0 Hz, 1H), 6.61 (dd, J = 4.5, 2.0 Hz, 1H), 6.32 (d, J = 16.0 Hz, 1H), 5.55 (s, 1H), 5.18 (s, 1H), 3.02 (m, 2H), 2.36–2.20 (m, 21H).
4.29 The identical method to that for 14a was used to synthesize and purify 5-((E)-3-(((2R,3R)-5,7-diacetoxy-2-(3,4,5-triacetoxyphenyl)chroman-3-yl)oxy)-3-oxoprop-1-en-1-yl)benzene-1,2,3-triyl triacetate (3c)
Analytical data: yield 81%; 1H NMR (500 MHz, CDCl3) δ 7.47 (t, J = 13.5 Hz, 1H), 7.28 (s, 2H), 7.27 (s, 2H), 6.67 (dd, J = 54.0, 2.0 Hz, 2H), 6.31 (d, J = 16.0 Hz, 1H), 5.55 (s, 1H), 5.18 (s, 1H), 3.09–2.92 (m, 2H), 2.38–2.20 (m, 24H).
4.30 Materials and cell culture
Melanocytes were purchased from Cellbio Biological Engineering Limited Company (Shanghai, China) and cultured at 37 °C in an incubator with 5% CO2 and 95% humidity. All culture media and supplements used for the isolation and cultivation of melanocytes were obtained from Gibco (Carlsbad, CA, USA) with the exception of recombinant human basic fibroblast growth factor (bFGF; Pepro-Tech, Rocky Hill, NJ, USA) and isobutylmethylxanthine (IBMX; Sigma-Aldrich, St Louis, MO, USA). The CytoTox 96 Non-Radioactive Cytotoxicity Assay kit and Lactate Dehydrogenase Assay kit were purchased from Promega (Madison, WI, USA). Cells were cultured in Hu16 medium (F12 supplemented with 10% fetal bovine serum (FBS), 20 ng mL−1 bFGF and 20 mg mL−1 IBMX) and cultured at 37 °C with 5% CO2 and 95% humidity. The cells were used between passages 2 and 5. Melanocytes were initially plated at a density of 1 × 104 cells per well in 96-well plates or at a density of 3 × 105 cells per well in 6-well plates and incubated overnight before each experiment.
4.31 Cell viability assay
Cell viability was measured using the Non-Radioactive Cell Proliferation Assay (MTS) kit in accordance with the manufacturer's protocol. To examine the cell viability, 10 μL of MTS solution was added to each well of 96-well plates and incubated at 37 °C for 1 h. The absorbance at 490 nm was measured by a microplate spectrophotometer (SpectraMax190; MDC, Sunnyvale, CA USA). All experiments were performed in triplicate in three independent experiments.
4.32 Lactate dehydrogenase (LDH) release assay
Melanocytes were initially plated into a 96-well plate. After pretreatment, supernatant from each well of the plate was transferred to the corresponding well of a 96-well enzymatic assay plate and incubated with reconstituted substrate mix for 30 min. The reaction was stopped by the addition of stop solution, and the absorbance was measured with a microplate spectrophotometer at 490 nm. The LDH leakage was then calculated as percentage (%) of the total LDH activity (LDH in the total cell lysate) according to the equation: % LDH released = (LDH activity in the supernatant/total LDH activity) × 100%.
4.33 In vitro JAK inhibition assays
The inhibitory activity of compounds against JAK1/2/3 enzyme was evaluated according to a published procedure.29 The incubation mixtures contained the following components: 1.1 nM JAK1/2/3, 1.5 μM peptide substrate (5-FAM-KKKKEEIYFFFG-OH for JAK2) and 30 μM ATP. After incubation for 180 min, the reaction mixture was analyzed on a Caliper LabChip 3000 (Caliper LifeSciences, Hopinkton, MA, USA) by electrophoretic separation of the fluorescent substrate and phosphorylated product. The virtual screening hits were firstly evaluated at the concentration of 20 μg mL−1, and further tested for seven concentrations prepared by 3- or 4-fold serial dilution if the preliminary activity was more than 60%. The IC50 values were generated by the analytical software Prism 5.0 (GraphPad Software Pte Ltd).
Acknowledgements
This work was supported by the Key Scientific Innovation Program of Hangzhou (Grant No: 20122513A02), the Science Foundation of the Ministry of Health (Grant No: WKJ2012-2-036), the National Natural Science Foundation of China (Grant No: 81271758 and 81472887), and the National Key Clinical Specialty Construction Project of China.
References
- K. Ezzedine, et al., Pigm. Cell Melanoma Res., 2012, 25, E1–E13 CrossRef CAS PubMed.
- T. Passeron and J.-P. Ortonne, J. Autoimmun., 2005, 25, 63–68 CrossRef CAS PubMed.
- S. Shin, S.-K. Hann and S. H. Oh, Photodermatol., Photoimmunol. Photomed., 2016, 32, 28–33 CrossRef CAS PubMed.
- N. C. Laddha, et al., Exp. Dermatol., 2013, 22, 245–250 CrossRef CAS PubMed.
- K. U. Schallreuter, J. M. Wood and J. Berger, J. Invest. Dermatol., 1991, 97, 1081–1085 CrossRef CAS PubMed.
- V. Maresca, et al., J. Invest. Dermatol., 1997, 109, 310–313 CrossRef CAS PubMed.
- K. U. Schallreuter and S. Elwary, Life Sci., 2007, 80, 2221–2226 CrossRef CAS PubMed.
- K. U. Schallreuter, et al., Biochem. Biophys. Res. Commun., 2007, 360, 70–75 CrossRef CAS PubMed.
- K. U. Schallreuter, et al., J. Invest. Dermatol., 2008, 128, 808–815 CrossRef CAS PubMed.
- K. U. Schallreuter, et al., J. Invest. Dermatol., 2001, 116, 167–174 CrossRef CAS PubMed.
- M. L. Dell'Anna, et al., Clin. Exp. Dermatol., 2007, 32, 631–636 CrossRef PubMed.
- M. A. Middelkamp-Hup, et al., J. Am. Acad. Dermatol., 2004, 50, 41–49 CrossRef PubMed.
- R. van den Wijngaard, A. Wankowicz-Kalinska, C. Le Poole, B. Tigges, W. Westerhof and P. Das, Lab. Invest., 2000, 80, 1299–1309 CrossRef CAS PubMed.
- Y. Lili, W. Yi, Y. Ji, S. Yue, S. Weimin and L. Ming, PLoS One, 2012, 7, e37513 CAS.
- S. F. Lin, et al., Eur. J. Med. Chem., 2010, 45, 6068–6076 CrossRef CAS PubMed.
- Y. Zhu, et al., Fitoterapia, 2014, 99, 243–251 CrossRef CAS PubMed.
- W.-X. Ning, S.-W. Wang, X.-W. Dong, D. Y. Liu, L.-F. Fu, R. Jin and A.-E. Xu, Biol. Pharm. Bull., 2015, 38, 1700–1706 CAS.
- Z.-Y. Chen, et al., J. Agric. Food Chem., 2001, 49(1), 477–482 CrossRef CAS PubMed.
- W. H. Lam, et al., Bioorg. Med. Chem., 2004, 12, 5587–5593 CrossRef CAS PubMed.
- T. C. Lei, V. M. Virador, W. D. Vieira and V. J. Hearing, Anal. Biochem., 2002, 305, 260–268 CrossRef CAS PubMed.
- J. H. Chen and C.-T. Ho, J. Agric. Food Chem., 1997, 45, 2374–2378 CrossRef CAS.
- P. Jovanovic, L. Zoric, I. Stefanovic, B. Dzunic, J. Djordjevic-Jocic, M. Radenkovic and M. Jovanovic, Bosnian J. Basic Med. Sci., 2010, 10, 83–88 CAS.
- H. Choi and J. Han, et al., J. Invest. Dermatol., 2013, 133, 528–536 CrossRef CAS PubMed.
- B. T. Utenova, K. E. Malterud and F. Rise, ARKIVOC, 2007, 9, 6–16 Search PubMed.
- L. Li and T. H. Chan, Org. Lett., 2001, 3, 739–741 CrossRef CAS PubMed.
- K. Osanai, C. Huo and K. R. Landis-Piwowar, et al., Tetrahedron, 2007, 63, 7565–7570 CrossRef CAS PubMed.
- C. A. Faler and M. M. Joullié, Org. Lett., 2007, 9, 1987–1990 CrossRef CAS PubMed.
- B. A. Horenstein and K. Nakanishi, J. Am. Chem. Soc., 1989, 111, 6242–6246 CrossRef CAS.
- H. H. Wan, G.
M. Schroeder, A. C. Hart, J. Inghrim, J. Grebinski, J. S. Tokarski, M. V. Lorenz, D. You, T. McDevie, B. Penhallow, R. Vuppugalla, Y. P. Zhang, X. M. Gu, R. Iyer, L. J. Lombardo, G. L. Trainor, S. Ruepp, J. Lippy, Y. Blat, J. S. Sack, J. A. Khan, K. Stefanski, B. Sleczka, A. Mathur, J. H. Sun, M. K. Wong, D. R. Wu, P. Li, A. Gupta, P. N. Arunachalam, B. Pragalathan, S. Narayanan, K. C. Nanjundaswamy, P. Kuppusamy and A. V. Purandare, ACS Med. Chem. Lett., 2015, 6, 850–855 CrossRef CAS PubMed.
Footnotes |
† Electronic supplementary information (ESI) available: Table S1. See DOI: 10.1039/c6ra23172a |
‡ These authors contributed equally to these work. |
|
This journal is © The Royal Society of Chemistry 2016 |