DOI:
10.1039/C6RA15754H
(Communication)
RSC Adv., 2016,
6, 74726-74733
Synthesis and evaluation of new chromene based [1,8]naphthyridines derivatives as potential antimicrobial agents†
Received
17th June 2016
, Accepted 25th July 2016
First published on 26th July 2016
Abstract
Novel chromeno[4,3-f][1,8]naphthyridines derivatives were prepared by multicomponent reaction between 2-((sub)amino)quinoline-3-carbaldehyde 1a–f/2a–f, ethyl 2-cyanoacetate 3 and cyclohexane-1,3-dione 4 in ethanol with L-proline as a catalyst. This approach provided the title compounds 5a–f/6a–f in good yields and under mild simple one-step reaction conditions. The structures of the new compounds were unambiguously established by spectroscopic and analytical techniques. In addition, synthesized compounds were further evaluated for antimicrobial activity using a broth microdilution method.
Introduction
Bacterial resistance is a significant worldwide health problem, and has attracted considerable attention particularly due to the emergence and dissemination of multi-drug resistant strains and undesirable side effects of known antibiotic drugs.1–3 In order to overcome the threat of wide spread multi-drug resistance in Gram positive and Gram negative bacterial strains as well as fungi, in spite of the large number of antibiotics and chemotherapeutics available for medical use, there is an ongoing demand for new antifungal and antibacterial agents in the future.
There are six different isomeric forms of naphthyridines, based on the position of the nitrogens in the bicyclic system. Among them, the 1,8-naphthyridine group of compounds have attracted significant attention of researchers in the field of medicinal chemistry.4,5 In addition, 1,8-naphthyridine skeleton is present in many compounds that have been isolated from natural substances, with broad range of interesting biological activities such as antibacterial, antimycobacterial,6 antiallergitic,7 antitumor,8,9 antiaggressive and antiinflammatory,10 antihypertensive,11 and antimalarias.12
Also, attached the chromene ring system is considered to be one of the most imperative heterocycles in nature as it has the distinction of being the parent ring in countless derivatives of biological relevance.13–21 For the synthesis of final compounds, we use quinoline is recognized as an important structures in medicinal chemistry which reside in molecules with various biological activities.22–26
We follow our previous work with the use of small organic molecule like L-proline as a catalyst with MCRs strategy.27,28 L-Proline is an inexpensive and abundant amino acid that could act either as a base or an acid or both in concert and also as a nucleophile, and it has been used in many chemical reactions with high yields.29–33 Inspired by our previously reported chromene derivatives (Fig. 1), we have reported the synthesis of a series of chromeno-1,8-naphthyridine derivatives by reaction (MCRs) of substituted aldehydes, cyanoethyacetate and cyclohexane-1,3-dione using MCRs catalyzed by L-proline (Scheme 1).
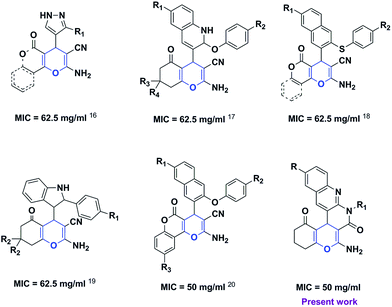 |
| Fig. 1 Previously reported chromene derivatives with their minimum MIC value. | |
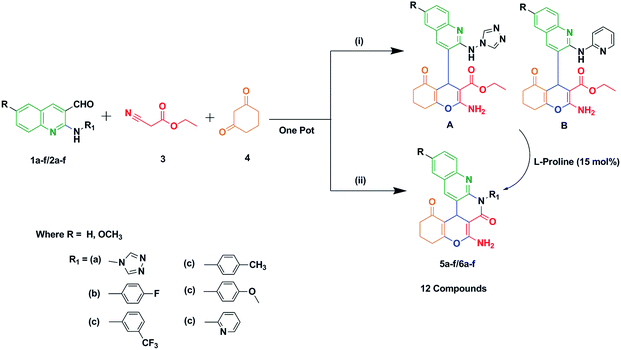 |
| Scheme 1 Synthetic pathway for the synthesis of chromeno[4,3-f][1,8]naphthyridines derivatives 5a–f/6a–f. Reagents and conditions: (i) different catalyst (5–10 mol%), 80 °C, 50–60 min to 3 h; (iii) L-proline (15 mol%), ethanol 80 °C, 2–3 h | |
Result and discussion
The key intermediates 2-((sub)amino)-6-(sub)quinoline-3-carbaldehyde 1a–f/2a–f were prepared by the Vilsmeier–Haack reaction of acetanilide to form 2-chloro-3-formylquinolines followed by the nucleophilic displacement of chloro group with different amines.34
The one-pot three-component cyclocondensation of a compounds 1a, ethyl 2-cyanoacetate 3 and cyclohexane-1,3-dione 4 in ethanol using different catalysts (5 mol%) e.g. pyridine, pyrrolidine, TEA (triethylamine), NaH (sodium hydride), L-proline, p-TSA (p-toluenesulfonic acid), acetic acid afforded only the targeted compounds A in good to excellent yields (55–80%). But in this study, quinoline second position of secondary amine was remaining free without reaction with carbonyl group which was confirmed by the proton spectra (compounds A and B). For the aim of cyclization to compound A, we are getting success in the cyclization of secondary amine and carbonyl groups using excess L-proline (15 mol%) at 80 °C temperature for 2–3 h as an optimal reaction conditions.
On the other way, we have attempted to use multicomponent strategy which includes the reaction of aldehydes 1a–f/2a–f, ethyl 2-cyanoacetate 3 and cyclohexane-1,3-dione 4 using different catalyst led to the final targeted compounds 5a–f/6a–f. In view of the success of the above reaction, we examined several catalysts for the scope of this promising reaction (Table 1). When pyridine/piperidine/TEA/NaH/L-proline/P-TSA/AcOH were used in the above reaction procedure, it was surprising to find that no yield of 5a was produced but only form A (Table 1, entries 1–7, yield 55–80%). Now, we were increased the reaction time from minutes to hours with amount of catalyst, there was no much change in the % yield of A (Table 1, entries 8–10, yield 75–80%). But, we were pleased to find that the expected yield of 5a could be increased by adding the sodium hydride and L-proline into the reaction compare to other catalyst (Table 1, entries 11–12, yield 5a 30–35%, A 60–65%). The data in Table 1, also disclose that increase the amount of catalyst (15 mol%) loading at the same time period is optimal for this transformation (Table 1, entry 19, yield 82%).
Table 1 Effects of different catalysts with 10 mL ethanol on the formation of 5a and Aa
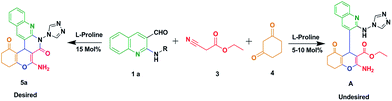
|
Entry |
Solvent |
Catalyst (mol%) |
Time |
% yieldb |
A |
5a |
All the reactions were carried out at 80 °C temperature. Isolated yield. No reaction occurred. m = minute, h = hour. |
1 |
EtOH |
Pyridine (5%) |
50–60 m |
75 |
—c |
2 |
EtOH |
Piperidine (5%) |
50–60 m |
80 |
—c |
3 |
EtOH |
TEA (5%) |
50–60 m |
80 |
—c |
4 |
THF |
NaH (5%) |
50–60 m |
80 |
—c |
5 |
EtOH |
L-Proline (5%) |
50–60 m |
80 |
—c |
6 |
EtOH |
P-TSA (5%) |
50–60 m |
60 |
—c |
7 |
EtOH |
AcOH (5%) |
50–60 m |
55 |
—c |
8 |
EtOH |
Pyridine (10%) |
2–3 h |
75 |
—c |
9 |
EtOH |
Piperidine (10%) |
2–3 h |
80 |
—c |
10 |
EtOH |
TEA (10%) |
2–3 h |
80 |
—c |
11 |
THF |
NaH (10%) |
2–3 h |
65 |
30 |
12 |
EtOH |
L-Proline (10%) |
2–3 h |
60 |
35 |
13 |
EtOH |
P-TSA (10%) |
2–3 h |
65 |
—c |
14 |
EtOH |
AcOH (10%) |
2–3 h |
55 |
—c |
15 |
EtOH |
Pyridine (15%) |
2–3 h |
75 |
—c |
16 |
EtOH |
Piperidine (15%) |
2–3 h |
76 |
—c |
17 |
EtOH |
TEA (15%) |
2–3 h |
70 |
—c |
18 |
THF |
NaH (15%) |
2–3 h |
25 |
70 |
19 |
EtOH |
L-Proline (15%) |
2–3 h |
10 |
82 |
20 |
EtOH |
P-TSA (15%) |
2–3 h |
55 |
—c |
21 |
EtOH |
AcOH (15%) |
2–3 h |
50 |
—c |
Also, one important point was found that the product 5a was increased with use of sodium hydride (Table 1, entry 18, yield 70%). Then, the scope of the reaction was investigated under the above optimal conditions (Table 1) using a series of equimolar amounts of 2-((sub)amino)quinoline-3-carbaldehyde 1a, ethyl 2-cyanoacetate 3 and cyclohexane-1,3-dione 4 in the presence of L-proline (15 mol%) in ethanol at 80 °C for 2–3 h, which afforded a final product 5a with 82% yield as a major product.
The most probable mechanism of L-proline mediated synthesis of chromeno[4,3-f][1,8]naphthyridines derivatives is illustrated in Scheme 2. The Knoevenagel condensation between aldehydes 1a–f/2a–f, ethyl 2-cyanoacetate 3, and L-proline followed by dehydration to form the heterylidenenitrile (i) which containing an electron-poor C
C double bond. Subsequent attack of cyclohexane-1,3-dione 4 to the intermediate (i), afforded (ii) which undergoes cyclization to form the intermediates (iii). Finally the intermediate (iii) undergoes intramolecular cyclization catalyzed by L-proline and forms the ultimate product (iv). To reveal the mechanistic pathway of the reaction course, we also isolated one such intermediate (iii). From the mechanism, it is apparent that both the secondary amine part and carboxylic acid part of L-proline significantly participate and catalyze the product formation.
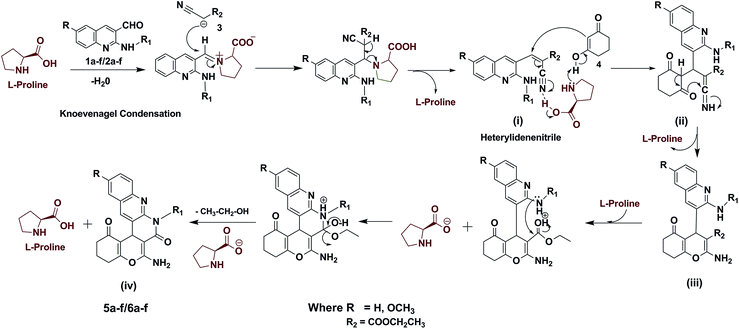 |
| Scheme 2 Possible mechanistic pathway for the synthesis of 5a–f/6a–f. | |
In this reaction, there were two possible pathways for the attack of amine to carbonyl groups. As shown in Fig. 2. Path-1 was difficult for the reaction of amine to carbonyl group as compare to path-2. The presence of easily removable group makes more favourable attack following the path 2, this was the main key factor of this research.
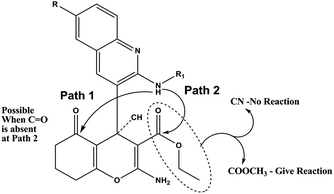 |
| Fig. 2 Possible pathway for the attack of amine. | |
Otherwise, one carbonyl group present on the path 1, we hope to the reaction of secondary amines with carbonyl group gave some different result. For the success of the path 1, we used malononitrile instead of ethyl 2-cyanoacetate but very confusing result has been generated which was unidentified. So, in our present molecule path 2 was only possibility to form the targeted compounds. We also use methyl 2-cyanoacetate, it was gave the same result as cyano ethyl acetate.
The structures of all the synthesized compounds were confirmed by 1H NMR, 13C NMR, IR and Mass spectrometry. The 1H NMR (DMSO-d6) spectrum of compound A, singlet peak observed at δ = 5.60 ppm of –CH proton (Fig. 2) and disappearance of –CHO peak which was observed in 1a at δ = 10.45 ppm which confirmed the cyclization of Knoevenagel intermediate as well as –NH2 protons of A gave singlet at δ = 6.60 ppm that conformed the NH2 group was present.
For the compounds 5a–f, the presence of three protons triplet in OCH2–
and quartet of two protons in
–CH3 of compound A which was disappeared in the compounds 5a–f which confirmed the cyclization reaction with removal of ethanol. In addition, 13C NMR spectra of compound 5a, a peak observed at around δ = 34.99 ppm for methane carbon (CH) which confirmed the cyclization. In FT-IR spectra, compounds 5a–f and 6a–f exhibited an absorption band at around 1580–1697 cm−1 for (C
O) stretching. Mass spectrometry of the synthesized compound 5a showed molecular ion peak 400.01 corresponding to exact mass. Similarly, all these compounds were characterized on the basis of spectral studies as well as elemental analysis.
Antimicrobials activity
All the synthesized compounds were screened by broth dilution method35,36 for their antibacterial activity against three Gram positive bacteria S. pneumonia, B. subtilis, C. tetani and three Gram negative bacteria E. coli, S. typhi and V. cholerae. They were also evaluated for their in vitro antifungal activity against C. albicans and A. fumigatus Ciprofloxacin and ampicillin were used as standard antibacterial drugs and griseofulvin and nystatin were used as standard antifungal drugs also tested under the similar conditions for comparison. The results are summarized in Table 2.
Table 2 Biological screening data of compounds (5a–f) and (6a–f)a
Minimum inhibitory concentration (MIC, μg ml−1) |
Compound |
Gram-positive bacteria |
Gram-negative bacteria |
Fungi |
S.P. |
B.S. |
C.T. |
E.C. |
S.T. |
V.C. |
C.A. |
A.F. |
MTCC 1936 |
MTCC 441 |
MTCC 449 |
MTCC 443 |
MTCC 98 |
MTCC 3906 |
MTCC 227 |
MTCC 3008 |
B.S.: Bacillus subtilis, C.T.: Clostridium tetani, S.P.: Streptococcus pneumonia, E.C.: Escherichia coli, S.T.: Salmonella typhi, V.C.: Vibrio cholera, A.F.: Aspergillus fumigatus, C.A.: Candida albicans, MTCC: Microbial Type Culture Collection. Ampi.: ampicillin, Cipro.: Ciprofloxacin, Grise.: griseofulvin, Nyst.: nystatin, (−). Not tested. Bold numbers indicate more potent compounds compared to standard drugs. |
5a |
250 |
62.5 |
250 |
500 |
200 |
500 |
250 |
1000 |
5b |
62.5 |
250 |
100 |
50 |
100 |
200 |
250 |
500 |
5c |
200 |
62.5 |
50 |
200 |
50 |
500 |
500 |
1000 |
5d |
250 |
500 |
500 |
100 |
100 |
200 |
1000 |
250 |
5e |
500 |
200 |
250 |
500 |
200 |
250 |
250 |
1000 |
5f |
200 |
250 |
62.5 |
500 |
500 |
200 |
500 |
500 |
6a |
500 |
200 |
200 |
500 |
250 |
200 |
250 |
250 |
6b |
100 |
50 |
200 |
62.5 |
500 |
62.5 |
250 |
500 |
6c |
100 |
62.5 |
200 |
50 |
250 |
200 |
500 |
>1000 |
6d |
200 |
500 |
250 |
100 |
250 |
500 |
1000 |
250 |
6e |
200 |
200 |
500 |
200 |
250 |
250 |
250 |
500 |
6f |
62.5 |
100 |
100 |
100 |
250 |
500 |
250 |
500 |
Amp |
250 |
250 |
250 |
100 |
100 |
100 |
— |
— |
Cipro |
50 |
50 |
100 |
25 |
25 |
25 |
— |
— |
Nyst |
— |
— |
— |
— |
— |
— |
100 |
100 |
Griseo |
— |
— |
— |
— |
— |
— |
500 |
100 |
For the compounds 5a–f (where R = H), compound 5a show the better activity against Gram positive bacteria Bacillus subtilis with (MIC = 62.5 μg mL−1) and fungi C. albicans (MIC = 250 μg mL−1). Compound 5b (electron withdrawing group –F) exhibited better activity against Gram negative bacteria E. coli (MIC = 50 μg mL−1), Gram positive bacteria S. pneumonia (MIC = 62.5 μg mL−1), C. tetani (MIC = 100 μg mL−1) and fungi C. albicans (MIC = 250 μg mL−1). Also, compound 5c (presence of CF3) exhibited better activity against Gram negative bacteria S. typhi (MIC = 50 μg mL−1), Gram positive bacteria S. pneumonia (MIC = 200 μg mL−1), B. subtilis (MIC = 62.5 μg mL−1), and C. tetani (MIC = 50 μg mL−1). Compound 5d (with CH3) show moderate activity to all the species. Compound 5e (presence of OCH3) was only active against Gram positive bacteria B. subtilis (MIC = 200 μg mL−1) and fungi C. albicans (MIC = 250 μg mL−1). Compound 5f show the better activity against Gram positive bacteria S. pneumonia (MIC = 200 μg mL−1) and C. tetani (MIC = 62.5 μg mL−1).
For compounds 6a–f (where R = OCH3), comp. 6a was active against Gram positive bacteria B. subtilis and C. tenani with MIC = 200 μg mL−1, and fungi C. albicans (MIC = 250 μg mL−1). Compound 6b exhibited better activity against Gram positive bacteria S. pneumonia (MIC = 100 μg mL−1), B. subtilis (MIC = 50 μg mL−1), C. tenani (MIC = 200 μg mL−1), and Gram negative bacteria E. coli and V. cholerae with (MIC = 62.5 μg mL−1), and fungi C. albicans (MIC = 250 μg mL−1). Compound 6c show the better activity against Gram positive bacteria S. pneumonia (MIC = 100 μg mL−1), B. subtilis (MIC = 62.5 μg mL−1), C. tenani (MIC = 200 μg mL−1) and Gram negative bacteria E. coli (MIC = 50 μg mL−1). Also, compound 6d was only exhibited good activity against Gram positive bacteria S. pneumonia (MIC = 200 μg mL−1). Compound 6e show good activity against Gram positive bacteria S. pneumonia (MIC = 200 μg mL−1), B. subtilis (MIC = 200 μg mL−1) and fungi C. albicans (MIC = 250 μg mL−1). Moreover, compound 6f exhibited the better activity against Gram positive bacteria S. pneumonia (MIC = 62.5 μg mL−1), B. subtilis (MIC = 100 μg mL−1), C. tenani (MIC = 100 μg mL−1) and fungi C. albicans (MIC = 250 μg mL−1).
Experimental section
Thin-layer chromatography (TLC) was carried out on aluminum supported silica gel plates (Merck 60F254, 0.25 mm thickness, Merck) with visualization of components by UV light. Column chromatography was carried out on silica gel (Merck 230–400 mesh). 1H and 13C NMR spectra were recorded on Bruker 400 MHz Spectrometer using DMSO-d6 as solvents and TMS as internal standard. Mass spectra were scanned on a Shimadzu LCMS 2010 spectrometer (Shimadzu, Tokyo, Japan). The IR spectra were recorded on a Perkin-Elmer Spectrum GX FT-IR Spectrophotometer (Perkine-Elmer, USA) using potassium bromide pellets in the range 4000–400 cm−1. Elemental analysis (% C, H, N) was carried out using Perkine Elmer 2400 series-II elemental analyzer (Perkine-Elmer, USA) and all compounds are within ±0.4% of the theoretical compositions.
General procedure for the synthesis of the title compounds 5a–f/6a–f
2-((sub)amino)quinoline-3-carbaldehyde 1a–f/2a–f (1 mmol), ethyl 2-cyanoacetate 3 (1 mmol) and cyclohexane-1,3-dione 4 (1 mmol) in the presence of L-proline (15 mol%) in ethanol (10 mL) at 80 °C for 2–3 h. The reaction progress was monitored by TLC (chloroform
:
methanol (9
:
1 mL)). Cool down the reaction mixture, liquid viscous crude mass was poured into cold water (25 mL), and then extracted with dichloromethane (30 mL × 3) and the organic layer was washed with water (20 mL × 3). The organic phase was dried with anhydrous sodium sulfate and filtrate it. Filtrate was evaporates obtain pure solid which on further recrystallized in volume ratio of chloroform and methanol (5
:
5 mL) to afforded a final product 5a–f/6a–f in 78–85% yields.
2-(4H-1,2,4-Triazol-4-ylamino)quinoline-3-carbaldehyde (1a). White solid; mp 120–125 °C; IR (KBr, νmax, cm−1) = 2930 (Ar-C-H), 3330 (–NH), 1613 (C
O str.); 1H NMR (400 MHz, DMSO-d6) δ = 7.81–8.45 (m, 6H), 8.88 (s, 1H), 9.69 (s, 1H), 10.45 (s, 1H, CHO) ppm; 13C NMR (100 MHz DMSO-d6) δ = 121.54, 123.55, 125.36, 126.55, 128.66, 132.55, 140.36, 145.66, 148.66, 158.57, 192.33 (C
O); MS calc. for C12H9N5O (239.23); anal. calc. C, 60.25; H, 3.79; N, 29.27; found: C 60.40, H 3.70, N 29.10%.
Ethyl 4-(2-(4H-1,2,4-triazol-4-ylamino)quinolin-3-yl)-2-amino-5-oxo-5,6,7,8-tetrahydro-4H-chromene-3-carboxylate (A). Light yellow solid; mp 220–224 °C; IR (KBr, νmax, cm−1) = 3432, 3371 (–NH); 1H NMR (400 MHz, DMSO-d6) δ = 1.05 (t, 3H, J1 = 6.4, J2 = 6.0,
), 1.79–2.70 (m, 6H, 3 × CH2), 3.56 (q, 2H, J = 7.6, J2 = 6.8,
) 5.60 (s, 1H, CH), 6.60 (s, 2H, NH2), 6.84–6.70 (m, 3H), 6.96–9.98 (m, 2H), 7.65 (m, 2H) 8.49 (s, 1H) ppm; 13C NMR (100 MHz DMSO-d6) δ = 20.20 (CH2), 25.11 (CH2), 34.12 (CH2CO), 37.53 (CH), 111.56, 113.56, 119.23, 121.23, 122.56, 123.56, 126.42, 128.92, 130.55, 132.55, 134.77, 136.21, 138.66, 145.63, 154.86, 162.36 (Ar-C), 168.46 and 195.63 (C
O) ppm; MS calc. for C23H22N6O4 (446.46); anal. calc. 61.87; H, 4.97; N, 18.82; found: C 61.97, H 5.12, N 18.98%.
Ethyl 2-amino-5-oxo-4-(2-(pyridin-2-ylamino)quinolin-3-yl)-5,6,7,8-tetrahydro-4H-chromene-3-carboxylate (B). Light yellow solid; mp 220–224 °C; IR (KBr, νmax, cm−1) = 3432, 3371 (–NH); 1H NMR (400 MHz, DMSO-d6) δ = 0.99 (t, 3H, J1 = 6.8, J2 = 7.2,
), 1.77–2.68 (m, 6H, 3 × CH2), 3.14 (q, 2H, J1 = 7.6, J2 = 6.8
), 5.52 (s, 1H, CH), 6.62 (s, 2H, NH2), 6.77 (t, 2H, J = 7.6), 6.99–7.04 (m, 3H, Ar-H), 7.16 (d, 1H, J = 6.8), 7.96 (d, 3H, J = 5.6), 8.87 (s, 1H) ppm; 13C NMR (100 MHz DMSO-d6) δ = 20.20 (CH2), 25.11 (CH2), 34.12 (CH2CO), 37.53 (CH), 111.56, 113.56, 119.23, 121.23, 122.56, 123.56, 124.32, 126.42, 128.92, 130.55, 132.12, 132.55, 134.77, 136.21, 137.26, 138.66, 140.63, 153.23, 154.86, 162.36 (Ar-C), 168.46 and 195.63 (C
O) ppm; MS calc. for C26H24N4O4 (456.46); anal. calc. C, 68.41; H, 5.30; N, 12.27; found: C 68.58, H 5.42, N 12.40%.
6-Amino-8-(4H-1,2,4-triazol-4-yl)-3,4,8,14b-tetrahydro-1H-benzo[b]chromeno[4,3-f][1,8]naphthyridine-1,7(2H)-dione (5a). Slight yellow solid; mp 201–205 °C; IR (KBr, νmax, cm−1) = 3333 and 3232 (–NH), 1H NMR (400 MHz, DMSO-d6) δ = 1.47–2.16 (m, 6H, 3 × CH2 of cyclohexenone ring), 5.19 (s, 1H, CH), 7.03 (s, 2H, NH2), 7.33 (d, 1H, J = 7.6), 7.42 (t, 1H, J = 7.2), 7.53 (t, 1H, J = 7.6), 7.64 (d, 1H, J = 8.0), 7.95–8.09 (m, 3H) ppm; 13C NMR (100 MHz DMSO-d6) δ = 21.18 (CH2), 28.19 (CH2), 34.99 (CH2CO), 36.61 (CH), 80.75, 111.08, 114.86, 119.78, 122.00, 127.99, 129.59, 131.40, 134.67, 138.09, 138.37, 138.59, 145.68, 161.68 (Ar-C), 169.41 and 195.70 (C
O) ppm; MS calc. for C21H16N6O3 (400.01); anal. calc. C, 62.99; H, 4.03; N, 20.99; found: C 63.12, H 4.15, N 21.10%.
6-Amino-8-(4-fluorophenyl)-3,4,8,14b-tetrahydro-1H-benzo[b]chromeno[4,3-f][1,8]naphthyridine-1,7(2H)-dione (5b). Light yellow solid; mp 220–224 °C; IR (KBr, νmax, cm−1) = 3432, 3371 (–NH2); 1H NMR (400 MHz, DMSO-d6) δ = 1.80–2.12 (m, 6H, 3 × CH2 of cyclohexenone ring), 5.12 (s, 1H, CH), 7.29 (s, 2H, NH2), 7.69 (t, 1H, J = 7.6), 7.86 (t, 1H, J = 7.2), 8.04 (d, 1H, J = 8.4), 8.12–8.14 (m, 5H), 8.58 (s, 1H) ppm; 13C NMR (100 MHz DMSO-d6) δ = 20.20 (CH2), 25.11 (CH2), 34.12 (CH2CO), 37.53 (CH), 80.36, 113.56, 119.23, 121.23, 122.56, 124.52, 124.88, 126.42, 128.92, 130.55, 132.55, 136.21, 137.26, 138.66, 140.63, 153.23, 162.36 (Ar-C), 168.46 and 195.63 (C
O) ppm; MS calc. for C25H18FN3O3 (427.45); anal. calc. C, 70.25; H, 4.24; N, 9.83; found: C 70.35, H 4.36, N 9.92%.
6-Amino-8-(3-(trifluoromethyl)phenyl)-3,4,8,14b-tetrahydro-1H-benzo[b]chromeno[4,3-f][1,8]naphthyridine-1,7(2H)-dione (5c). Light yellow solid; mp 235–238 °C; IR (KBr, νmax, cm−1) = 3411 and 3336 (–NH2); 1H NMR (400 MHz, DMSO-d6) δ = 1.37–2.11 (m, 6H, 3 × CH2 of cyclohexenone ring), 5.03 (s, 1H, CH), 7.20 (s, 2H, NH2), 7.39 (t, 1H, J = 7.6), 7.56 (d, 1H, J = 7.6), 7.72–7.99-(m, 6H), 8.24 (s, 1H) ppm; 13C NMR (100 MHz DMSO-d6) δ = 21.22 (CH2), 25.23 (CH2), 34.78 (CH2CO), 36.53 (CH), 80.23, 112.53, 119.41, 121.69, 122.96, 123.23, 124.36, 124.96, 126.52, 127.63, 130.84, 132.48, 134.94, 136.36, 137.45, 138.71, 140.69, 153.73, 154.23, 162.46 (Ar-C), 168.13 and 195.66 (C
O) ppm; MS calc. for C26H18F3N3O3 (477.05); anal. calc. C, 65.41; H, 3.80; N, 8.80; found: C 65.55, H 3.90, N 8.89%.
6-Amino-8-p-tolyl-3,4,8,14b-tetrahydro-1H-benzo[b]chromeno[4,3-f][1,8]naphthyridine-1,7(2H)-dione (5d). Light yellow solid; mp 205–208 °C; IR (KBr, νmax, cm−1) = 3416 and 3360 (–NH);,1H NMR (400 MHz, DMSO-d6) δ = 1.78–2.11 (m, 9H, 3 × CH2 of cyclohexenone ring + CH3), 5.64 (s, 1H, CH), 7.39–7.52 (m, 5H, Ar-H + NH2), 7.72 (t, 1H, J1 = 7.6, J2 = 8.0), 7.84–7.93 (m, 2H), 8.06 (d, 1H, J = 8.8), 8.21 (s, 1H), 8.34 (s, 1H) ppm; 13C NMR (100 MHz DMSO-d6) δ = 20.19 (CH3), 21.23 (CH3), 25.19 (CH2), 34.69 (CH2CO), 36.56 (CH), 79.79, 111.11, 114.56, 119.55, 121.78, 122.51, 123.23, 126.69, 128.74, 130.74, 132.66, 134.96, 137.48, 138.74, 140.66, 154.69, 162.74 (Ar-C), 168.42 and 195.74 (C
O) ppm; MS calc. for C26H21N3O3 (423.16); anal. calc. C, 73.74; H, 5.00; N, 9.92; found: C 73.93, H 5.11, N 10.01%.
6-Amino-8-(4-methoxyphenyl)-3,4,8,14b-tetrahydro-1H-benzo[b]chromeno[4,3-f][1,8]naphthyridine-1,7(2H)-dione (5e). Light yellow solid; mp 210–214 °C; IR (KBr, νmax, cm−1) = 3317 and 3217 (–NH); 1H NMR (400 MHz, DMSO-d6) δ = 2.08–2.21 (m, 6H, 3 × CH2 of cyclohexenone ring), 3.75 (s, 3H, OCH3), 5.13 (s, 1H, CH), 7.24 (s, 2H, NH2), 7.63 (t, 1H, J1 = 8, J2 = 7.2), 7.73–7.80 (m, 2H), 7.89–7.97 (m, 3H), 8.15–8.21 (m, 3H) ppm; 13C NMR (100 MHz DMSO-d6) δ = 20.81 (CH2), 24.53 (CH2), 34.23 (CH2CO), 37.56 (CH), 78.23, 113.12, 120.66, 121.63, 122.58, 123.63, 124.87, 128.69, 130.74, 132.66, 134.96, 136.74, 137.66, 138.74, 140.56, 158.74, 162.69 (Ar-C), 168.56 and 195.41 (C
O) ppm; MS calc. for C26H21N3O4 (439.15); anal. calc. C, 71.06; H, 4.82; N, 9.56; found: C 70.21, H 0.4.96 N 9.65%.
6-Amino-8-(pyridin-2-yl)-3,4,8,14b-tetrahydro-1H-benzo[b]chromeno[4,3-f][1,8]naphthyridine-1,7(2H)-dione (5f). Light yellow solid; mp 220–225 °C; IR (KBr, νmax, cm−1) = 3405 and 3295 (–NH); 1H NMR (400 MHz, DMSO-d6) δ = 1.99–2.32 (m, 6H, 3 × CH2 of cyclohexenone ring), 5.63 (s, 1H, CH), 6.72 (s, 2H, NH2), 7.06 (d, 1H, J = 7.2), 7.16–7.19 (m, 5H), 7.45 (s, 1H), 7.76 (t, 1H, J = 7.2), 7.92 (t, 1H, J = 6.4) ppm; 13C NMR (100 MHz DMSO-d6) δ = 20.77 (CH2), 25.63 (CH2), 35.23 (CH2CO), 37.56 (CH), 79.38, 113.63, 119.75, 121.69, 122.74, 123.01, 124.63, 125.23, 128.11, 130.63, 132.74, 134.17, 137.12, 138.46, 141.42, 153.23, 154.74, 161.56 (Ar-C), 169.23 and 195.56 (C
O) ppm; MS calc. for C24H18N4O3 (410.10); anal. calc. C, 70.23; H, 4.42; N, 13.65; found: C 70.38, H 4.51; N, 13.79%.
6-Amino-12-methoxy-8-(4H-1,2,4-triazol-4-yl)-3,4,8,14b-tetrahydro-1H-benzo[b]chromeno[4,3-f][1,8]naphthyridine-1,7(2H)-dione (6a). Light yellow solid; mp 201–205 °C; IR (KBr, νmax, cm−1) = 3333 and 3232 (–NH), 1H NMR (400 MHz, DMSO-d6) δ = 1.55–2.13 (m, 6H, 3 × CH2 of cyclohexenone ring), 3.81 (m, 3H, OCH3), 5.34 (s, 1H, CH), 6.19 (s, 2H, NH2), 6.30 (d, 1H, J = 8), 6.78 (d, 1H, J = 8), 7.02 (s, 1H), 7.18 (s, 1H), 7.86–7.6 (m, 2H) ppm; 13C NMR (100 MHz DMSO-d6) δ = 21.20 (CH2), 28.17 (CH2), 34.94 (CH2CO), 36.70 (CH), 58.78 (OCH3), 80.09, 111.47, 114.98, 119.75, 122.13, 124.77, 127.40, 130.92, 132.05, 134.43, 136.31, 145.05, 154.44, 161.44 (Ar-C), 169.31 and 195.59 (C
O) ppm; MS calc. for C22H18N6O4 (430.03); anal. calc. C, 61.39; H, 4.22; N, 19.53; found: C 61.48, H 4.32, N 19.65%.
6-Amino-8-(4-fluorophenyl)-12-methoxy-3,4,8,14b-tetrahydro-1H-benzo[b]chromeno[4,3-f][1,8]naphthyridine-1,7(2H)-dione (6b). Light yellow solid; mp 220–224 °C; IR (KBr, νmax, cm−1) = 3432, 3371 (–NH); 1H NMR (400 MHz, DMSO-d6) δ = 1.39–2.14 (m, 6H, 3 × CH2 of cyclohexenone ring), 3.60 (s, 3H, OCH3), 5.21 (s, 1H, CH), 7.21 (s, 2H, NH2), 7.38–7.47 (m, 1H), 7.57–7.62 (m, 5H), 7.70 (d, 1H, J = 7.2), 7.96 (d, 1H, J = 8.0) ppm; 13C NMR (100 MHz DMSO-d6) δ = 20.31 (CH2), 25.74 (CH2), 34.56 (CH2CO), 37.74 (CH), 57.86 (OCH3), 79.63, 110.11, 114.56, 121.85, 122.63, 126.33, 128.78, 130.66, 132.55, 134.88, 136.36, 137.79, 138.56, 140.78, 153.63, 154.56, 162.41 (Ar-C), 168.66 and 195.17 (C
O) ppm; MS calc. for C26H20FN3O4 (457.45); anal. calc. C, 68.26; H, 4.41; N, 9.19; found: C 68.40, H 4.52, N 9.28%.
6-Amino-12-methoxy-8-(3-(trifluoromethyl)phenyl)-3,4,8,14b-tetrahydro-1H-benzo[b]chromeno[4,3-f][1,8]naphthyridine-1,7(2H)-dione (6c). Light yellow solid; mp 235–238 °C; IR (KBr, νmax, cm−1) = 3411 and 3336 (–NH2); 1H NMR (400 MHz, DMSO-d6) δ = 1.55–1.97 (m, 6H, 3 × CH2 of cyclohexenone ring), 3.32 (s, 3H, OCH3), 5.38 (s, 1H, CH), 7.25 (s, 2H, NH2), 7.60–7.64 (m, 1H), 7.72–7.95 (m, 3H), 7.89–7.96 (m, 2H) 8.17–8.20 (m, 2H) ppm; 13C NMR (100 MHz DMSO-d6) δ = 21.23 (CH2), 25.66 (CH2), 34.78 (CH2CO), 37.74 (CH), 57.23 (OCH3), 79.25, 110.63, 113.21, 119.63, 121.56, 122.88, 123.69, 124.62, 126.85, 128.92, 130.66, 132.33, 132.02, 136.63, 137.74, 138.86, 140.96, 153.74, 154.86, 162.55 (Ar-C), 169.23 and 196.11 (C
O) ppm; MS calc. for C27H20F3N3O4 (507.30); anal. calc. C, 63.90; H, 3.97; N, 8.28; found: C 64.08, H 4.12, N 8.36%.
6-Amino-12-methoxy-8-p-tolyl-3,4,8,14b-tetrahydro-1H-benzo[b]chromeno[4,3-f][1,8]naphthyridine-1,7(2H)-dione (6d). Light yellow solid; mp 205–208 °C; IR (KBr, νmax, cm−1) = 3416 and 3360 (–NH2);,1H NMR (400 MHz, DMSO-d6) δ = 1.95–2.23 (m, 9H, 3 × CH2 of cyclohexenone ring + CH3), 3.31 (s, 3H, OCH3), 5.21 (s, 1H, CH), 7.24 (s, 2H, NH2), 7.35–7.58 (m, 4H), 7.10 (t, 1H, J = 7.6), 7.84 (d, 2H, J = 8.0), 8.09 (s, 1H) ppm; 13C NMR (100 MHz DMSO-d6) δ = 20.10 (CH3), 21.29 (CH2), 25.66 (CH2), 34.93 (CH2CO), 36.53 (CH), 58.52 (OCH3), 80.25, 111.23, 114.63, 120.74, 121.33, 122.74, 124.63, 125.23, 127.80, 128.32, 131.74, 135.47, 138.41, 139.47, 141.63, 150.79, 153.91, 161.55 (Ar-C), 168.36 and 197.12 (C
O) ppm; MS calc. for C27H23N3O4 (453.17); anal. calc. C, 71.51; H, 5.11; N, 9.27; found: C 71.66, H 5.31, N 9.40%.
6-Amino-12-methoxy-8-(4-methoxyphenyl)-3,4,8,14b-tetrahydro-1H-benzo[b]chromeno[4,3-f][1,8]naphthyridine-1,7(2H)-dione(6e). Light yellow solid; mp 210–214 °C; IR (KBr, νmax, cm−1) = 3317 and 3217 (–NH2); 1H NMR (400 MHz, DMSO-d6) δ = 2.26–2.46 (m, 6H, 3 × CH2 of cyclohexenone ring), 3.72–3.89 (m, 6H, 2 × OCH3), 5.55 (s, 1H, CH), 7.01–7.05 (m, 4H, Ar-H + NH2), 7.37 (s, 1H), 7.47 (s, 1H), 7.57–7.83 (m, 4H, Ar-H); 13C NMR (100 MHz DMSO-d6) δ = 21.29 (CH2), 24.56 (CH2), 34.81 (CH2CO), 37.36 (CH), 57.12, 57.92 (OCH3), 79.26, 110.56, 115.74, 120.56, 122.52, 123.96, 125.49, 126.74, 128.82, 130.59, 132.74, 137.41, 140.66, 145.74, 149.63, 158.56, 160.55 (Ar-C), 168.74 and 197.63 (C
O) ppm; MS calc. for C27H23N3O5 (469.40); anal. calc. C, 69.07; H, 4.94; N, 8.95; found: C 69.18, H 5.11, N 9.02%.
6-Amino-12-methoxy-8-(pyridin-2-yl)-3,4,8,14b-tetrahydro-1H-benzo[b]chromeno[4,3-f][1,8]naphthyridine-1,7(2H)-dione (6f). Light yellow solid; mp 220–225 °C; IR (KBr, νmax, cm−1) = 3405 and 3295 (–NH); 1H NMR (400 MHz, DMSO-d6) δ = 1.62–2.19 (m, 6H, 3 × CH2 of cyclohexenone ring), 3.42 (s, 3H, OCH3), 5.49 (s, 1H, CH), 7.21 (s, 2H, NH2), 7.41–7.44 (m, 2H), 7.56–7.72 (m, 3H), 7.81–7.91 (m, 2H), 8.33 (s, 1H); 13C NMR (100 MHz DMSO-d6) δ = 21.32 (CH2), 25.12 (CH2), 34.62 (CH2CO), 37.50 (CH), 58.31 (OCH3), 77.49, 110.46, 115.74, 120.74, 121.78, 122.49, 124.74, 127.12, 129.48, 130.52, 132.65, 134.59, 136.49, 139.21, 144.41, 150.74, 155.63, 162.55 (Ar-C), 169.36 and 198.47 (C
O) ppm; MS calc. for C25H20N4O4 (440.01); anal. calc. C, 68.17; H, 4.58; N, 12.72; found: C 68.31, H 4.68; N, 12.86%.
Antimicrobial screening method35
All the glass apparatus used were sterilized before use. Mueller–Hinton broth was used as nutrient medium to grow and dilute the compound suspension for the test bacteria and Sabouraud Dextrose broth used for fungal nutrition. Inoculums size for test strain was adjusted to 108 CFU [Colony Forming Unit] per milliliter by comparing the turbidity. Each synthesized compound was diluted obtaining 2000 μg mL−1 concentration, as a stock solution. The results are recorded in the form of primary and secondary screening. The compounds 5a–f and 6a–f were screened for their antibacterial activity against three Gram-negative (Vibrio cholera MTCC 3906, Escherichia coli MTCC 443 and Salmonella typhi MTCC 98) bacteria and three Gram-positive (Streptococcus pneumonia MTCC 96, Clostridium tetani MTCC 449 and Bacillus subtilis MTCC 441) bacteria at concentrations of 1000, 500, and 250 μg mL−1 as primary screening. DMSO was used as vehicle to get desired concentrations of compounds to test upon microbial strains. The compounds found to be active in the primary screening, were further screened in a second set of dilution at concentrations of 200, 100, 62.5, and 50 μg mL−1. Ten microliter suspensions from each well were further inoculated and growth was noted after 24 and 48 h. The lowest concentration, which showed no visible growth (turbidity) after spot subculture was considered as MIC for each compound. In this study, ampicillin, and ciprofloxacin were used as standard antibacterial drugs, whereas griseofulvin and nystatin were used as standard antifungal drugs. The protocols are summarized in Table 2.
Conclusion
To the best of our knowledge, this is the first convenient procedure for the synthesis of chromeno based naphthyridines derivatives by the multicomponent reaction between 2-((sub)amino)quinoline-3-carbaldehyde 1a–f/2a–f, ethyl 2-cyanoacetate 3 and cyclohexane-1,3-dione 4 in ethanol with L-proline as a catalyst. Out of twelve, eleven compounds displayed good in vitro antimicrobials activity ranging from 50 to 200 μg mL−1. From the result of activity data, the most potent compounds 5b, 5c, 6b and 6c were show the better activity against to Gram negative and Gram positive bacteria with minimum MIC = 50 μg mL−1, and also compounds 5b and 6b show good antifungal activity (MIC = 250 μg mL−1). These excellent activities could be credited with presence of electron withdrawing group (F, CF3). Also, compounds contained methyl group show the moderate activity (5d, 6d). Moreover, triazole containing compounds show the better antifungal activity (MIC = 250 μg mL−1) i.e. 5a, 6a.
Acknowledgements
The authors are thankful to the Head, Department of Chemistry, Sardar Patel University for providing 1H NMR and 13C NMR spectroscopy and research facilities. We are also thankful to the DST-Pursed, for providing mass spectroscopy facilities, Vallabh Vidyanagar, Gujarat, India. One of the authors Jayvirsinh D. Gohil is grateful to UGC, New Delhi for Basic Science Research Fellowship for Meritorious Students.
References
- T. Frieden, US department of Health & Human Services, accessed in Feb 16, 2014, at http://www.hhs.gov/asl/testify/2010/04/t20100428b.html, 2010.
- H. W. Boucher, G. H. Talbot, J. S. Bradley, J. E. Edwards, D. Gilbert, L. B. Rice, M. Scheld, B. Spellberg and J. Bartlett, Clin. Infect. Dis., 2009, 48, 1–12 CrossRef PubMed.
- J. L. Martinez, A. Fajardo, L. Garmendia, A. Hernandez, J. F. Linares, L. Martínez-Solano and M. B. Sánchez, FEMS Microbiol. Rev., 2009, 33, 44–65 CrossRef CAS PubMed.
- V. P. Litvinov, Russ. Chem. Rev., 2004, 73, 637–670 CrossRef CAS.
- A. Madaan, R. Verma, V. Kumar, A. T. Singh, S. K. Jain and M. Jaggi, Arch. Pharm., 2015, 348, 837–860 CrossRef CAS PubMed.
- M. Badawneh, L. Bellini, T. Cavallini, C. Manera, G. Saccomanni and P. L. Ferrarini, Il Farmaco, 2003, 58, 859–866 CrossRef CAS PubMed.
- M. H. Sherlock, J. J. Kaminski, W. C. Tom, J. F. Lee, S. C. Wong, W. Kreutner, R. W. Bryant and A. T. McPhail, J. Med. Chem., 1988, 31, 2108–2121 CrossRef CAS PubMed.
- L. Fu, X. Feng, J.-J. Wang, Z. Xun, J.-D. Hu, J.-J. Zhang, Y.-W. Zhao, Z.-B. Huang and D.-Q. Shi, ACS Comb. Sci., 2014, 17, 24–31 CrossRef PubMed.
- P. Acosta, E. Butassi, B. Insuasty, A. Ortiz, R. Abonia, S. A. Zacchino and J. Quiroga, Molecules, 2015, 20, 8499–8520 CrossRef CAS PubMed.
- G. Roma, M. Di Braccio, G. Grossi, F. Mattioli and M. Ghia, Eur. J. Med. Chem., 2000, 35, 1021–1035 CrossRef CAS PubMed.
- P. L. Ferrarini, C. Mori, M. Badawneh, V. Calderone, R. Greco, C. Manera, A. Martinelli, P. Nieri and G. Saccomanni, Eur. J. Med. Chem., 2000, 35, 815–826 CrossRef CAS PubMed.
- G. Barlin and W. Tan, Aust. J. Chem., 1984, 37, 1065–1073 CrossRef CAS.
- F. M. Abdelrazek, P. Metz, O. Kataeva, A. Jaeger and S. F. El-Mahrouky, Arch. Pharm., 2007, 340, 543–548 CrossRef CAS PubMed.
- N. M. Sabry, H. M. Mohamed, E. S. A. Khattab, S. S. Motlaq and A. M. El-Agrody, Eur. J. Med. Chem., 2011, 46, 765–772 CrossRef CAS PubMed.
- S. Rahmani-Nezhad, M. Safavi, M. Pordeli, S. K. Ardestani, L. Khosravani, Y. Pourshojaei, M. Mahdavi, S. Emami, A. Foroumadi and A. Shafiee, Eur. J. Med. Chem., 2014, 86, 562–569 CrossRef CAS PubMed.
- N. D. Vala, H. H. Jardosh and M. P. Patel, Chin. Chem. Lett., 2016, 27, 168–172 CrossRef CAS.
- C. B. Sangani, N. M. Shah, M. P. Patel and R. G. Patel, Med. Chem. Res., 2013, 22, 3831–3842 CrossRef CAS.
- J. A. Makawana, M. P. Patel and R. G. Patel, Arch. Pharm., 2012, 345, 314–322 CrossRef CAS PubMed.
- H. G. Kathrotiya and M. P. Patel, Med. Chem. Res., 2012, 21, 3406–3416 CrossRef CAS.
- D. C. Mungra, M. P. Patel, D. P. Rajani and R. G. Patel, Eur. J. Med. Chem., 2011, 46, 4192–4200 CrossRef CAS PubMed.
- E. Maalej, F. Chabchoub, M. J. Oset-Gasque, M. Esquivias-Pérez, M. P. González, L. Monjas, C. Pérez, C. de los Ríos, M. I. Rodríguez-Franco and I. Iriepa, Eur. J. Med. Chem., 2012, 54, 750–763 CrossRef CAS PubMed.
- G. G. Ladani and M. P. Patel, New J. Chem., 2015, 39, 9848–9857 RSC.
- O. Afzal, S. Kumar, M. R. Haider, M. R. Ali, R. Kumar, M. Jaggi and S. Bawa, Eur. J. Med. Chem., 2015, 97, 871–910 CrossRef CAS PubMed.
- I. Briguglio, R. Loddo, E. Laurini, M. Fermeglia, S. Piras, P. Corona, P. Giunchedi, E. Gavini, G. Sanna and G. Giliberti, Eur. J. Med. Chem., 2015, 105, 63–79 CrossRef CAS PubMed.
- N. Shobeiri, M. Rashedi, F. Mosaffa, A. Zarghi, M. Ghandadi, A. Ghasemi and R. Ghodsi, Eur. J. Med. Chem., 2016, 114, 14–23 CrossRef CAS PubMed.
- S. R. Patel, R. Gangwal, A. T. Sangamwar and R. Jain, Eur. J. Med. Chem., 2015, 93, 511–522 CrossRef CAS PubMed.
- M. B. Kanani and M. P. Patel, RSC Adv., 2014, 4, 28798–28801 RSC.
- G. G. Ladani and M. P. Patel, RSC Adv., 2015, 5, 76943–76948 RSC.
- P. Gunasekaran, P. Prasanna and S. Perumal, Tetrahedron Lett., 2014, 55, 329–332 CrossRef CAS.
- A. Hasaninejad, S. Firoozi and F. Mandegani, Tetrahedron Lett., 2013, 54, 2791–2794 CrossRef CAS.
- L. C. Rao, H. Meshram, N. S. Kumar, N. N. Rao and N. J. Babu, Tetrahedron Lett., 2014, 55, 1127–1131 CrossRef.
- S. Chandrasekhar, K. Vijeender and C. Sridhar, Tetrahedron Lett., 2007, 48, 4935–4937 CrossRef CAS.
- L. Fu, W. Lin, M.-H. Hu, X.-C. Liu, Z.-B. Huang and D.-Q. Shi, ACS Comb. Sci., 2014, 16, 238–243 CrossRef CAS PubMed.
- J. D. Gohil, H. B. Patel and M. P. Patel, Heterocycl. Lett., 2016, 6, 31–42 Search PubMed.
- M. Ferraro, Methods for Dilution Antimicrobial Susceptibility Tests for Bacteria That Grow Aerobically; Approved Standard, 8th edn, 2003 Search PubMed.
- J. D. Gohil, H. B. Patel and M. P. Patel, Indian J. Adv. Chem. Sci., 2016, 4, 102–113 Search PubMed.
Footnote |
† Electronic supplementary information (ESI) available. See DOI: 10.1039/c6ra15754h |
|
This journal is © The Royal Society of Chemistry 2016 |
Click here to see how this site uses Cookies. View our privacy policy here.