DOI:
10.1039/C6RA16162F
(Paper)
RSC Adv., 2016,
6, 74734-74741
Thermosensitive molecularly imprinted polymers based on magnetic nanoparticles for the recognition of sulfamethazine†
Received
22nd June 2016
, Accepted 26th July 2016
First published on 27th July 2016
Abstract
In this study, a thermosensitive molecularly imprinted polymer (TMIP) was successfully synthesized for the selective recognition of sulfamethazine (SMZ) on the surface of magnetic nanoparticles. N-Isopropylacrylamide (NIPAM) as the thermosensitive monomer, methacrylic acid (MAA) as the functional monomer, ethyleneglycol dimethacrylate (EGDMA) as the cross-linking agent and azodiisobutyronitrile (AIBN) as the initiator were selected to prepare the TMIPs. The thermosensitive magnetic molecularly imprinted polymers were sensitive to the temperature and the adsorption/desorption process could be controlled by changing the temperature because the thermoresponsive monomer NIPAM can undergo a reversible volume transition between the swollen and collapsed phases. Moreover, the resultant TMIPs showed high adsorption capacity and specific affinity towards SMZ. The adsorption capacity of TMIPs reached the maximum when the adsorption temperature was around the LCST of NIPAM. The magnetic property of TMIPs provided fast separation while the thermoresponsive property offered simple elution for templates. Finally, the imprinted polymers were used for the enrichment of SMZ from real water with recoveries ranging from 83.2% to 96.8% determined by high performance liquid chromatography. The results indicated that the TMIPs were efficient for thermally modulated capture and release of the template, which enable its application for trace sulfamethazine in complicated samples.
1. Introduction
Sulfonamides are a kind of commonly used synthetic antimicrobial, which have been widely applied in veterinary clinical treatment and in animal feeds to promote livestock growth.1 Nevertheless, the wanton abuse of antibiotics has begun to show negative effects, which could endanger human health and cause allergies, carcinogenesis, and antibiotic resistance.2 Moreover, due to the extremely low concentration of antibiotics in water, it is difficult to remove the sulfonamides by conventional treatment technologies.3 So, developing a novel technology for the efficient detection and separation of sulfonamides from water is a great necessity and of importance.
Molecularly imprinting is an effective technique to produce specific binding sites for template molecules. Because the tailor-made recognition sites formed in the polymers are complementary in shape, size and functionality to the target molecules. In recent years, the molecularly imprinted polymers have been applied in various fields, such as solid phase extractions,4,5 sensors,6 drug delivery,7 and artificial antibodies.12–14 As we all known, several approaches to prepare molecularly imprinted polymers have been developed, for example, bulk imprinting, surface imprinting, and epitope imprinting.10 And among these methods, surface imprinting was the most popular one, by which the binding sites were immobilized on the surface of solid substrates.11 Thus, the restricted mass transfer would be reduced and the targets would be easily attached to the binding sites in this way. To date, several polymers have been prepared by surface imprinting as solid-phase extracting sorbents or as the stationary phase to adsorb SAs, such as sulfamethoxazole and sulfamethazine.1,8,15
Magnetic nanoparticles have been widely used as supporting matrix due to their easy preparation and low toxicity.9 What's more, Fe3O4 nanoparticles can be rapidly separated from sample solutions by an external magnetic field. And in recent years, considerable attention has been focused on temperature responsive materials. Because this kind polymers are sensitive to temperature and the structure of polymers would undergo volume phase transitions upon the temperature changed.16,17 What's more important, this kind transformation was reversible. Poly(Nisopropylacrylamide) (PNIPAM) is one of the most widely used thermosensitive monomer, containing a proportion of hydrophilic groups and hydrophobic groups. Lower Critical Solution Temperature (LCST) as the most important feature of PNIPAM is 32 °C.20 When the temperature was above the LCST, the polymers showed a hydrophobic, collapsed state and when the temperature was below the LCST, the polymers showed a hydrophilic, swollen state. So, the TMIPs can capture or release the template molecules just by changing external temperature. Thus, if we combine the temperature-responsive feature with magnetic molecularly imprinted polymers, then we would obtain a kind of material exhibiting both of their preponderances.
In this work, thermoresponsive molecularly imprinted polymers for selective recognition of sulfamethazine (SMZ) were synthesized. And the N-isopropylacrylamide (NIPAM) was selected as a thermosensitive monomer, which contains the hydrophobic isopropyl and hydrophilic amide group. Methacrylic acid (MAA) worked as additional monomer, which could form a stable complex with the template molecules mainly via hydrogen bonding interactions. Ethylene glycoldimethacrylate (EGDMA) as crosslinker was chosen to crosslink with functional monomers, forming a rigid structure in order to make the binding sites fixed. The temperature-responsive behavior of the polymers was investigated at different temperatures in this work. In order to research the binding properties of the TMIPs for SMZ, adsorption rate, adsorption capacity, and selectivity were investigated. Finally, the prepared imprinted polymers with optimal performance were successfully applied to absorb sulfamethazine from real water samples.
2. Experimental
2.1 Materials
FeCl3·6H2O (≥99%) was supplied by Beijing Chemical Plant. Poly(4-styrenesulfonic acid-co-maleic acid, SS
:
MA = 3
:
1) sodium salt (PSSMA) was obtained from Sigma-Aldrich. Sulfadiazine (SDZ; 99%), sulfamethazine (SMZ; 99%), sulfamethoxazole (SMO; 99%), methacrylic acid (MAA; 99%) and N-isopropylacrylamide (NIPAM; 98%) were purchased from Aladdin Reagent Co., Ltd. (Shanghai, China). EGDMA, ethylene glycol, tetraethyl orthosilicate (TEOS; 98%) and γ-methacryloxypropyltrimethoxysilane (KH-570) were supplied by Sigma-Aldrich Chemical Co. (St. Louis, MO, USA). Hydrochloric acid (37%) was received from Nanjing Chemical Reagent Co., Ltd. (Nanjing, China). Glacial acetic acid (HAc), azobisisobutyronitrile (AIBN), acetonitrile, methanol, ethanol were obtained from Sinopharm Chemical Reagent Co., Ltd. (Shanghai, China). Methanol (for HPLC) was obtained from Tedia Co., Inc. (Ohio). All of the reagents were at least analytical-reagent grade and were used without further purification.
2.2 Instruments
Fourier Transform Infrared (FTIR) spectra were recorded on a Nicolet Nexus-470 FTIR spectrometer (USA) in the range of 450–4000 cm−1. The samples were mixed with KBr and pressed into a compact pellet. Morphology and structure of the microspheres were observed by a JEM-200CX high-resolution TEM instrument (JEOL, Japan). The magnetic properties of magnetic microspheres were assessed with a vibrating sample magnetometer (VSM, LakeShore 7307). The identification of the crystallographic structure was performed using a Rigaku D/max-γ B X-ray diffractometer (XRD) with monochromatized with Cu Kα radiation over the 2θ range of 20–80° at a scanning rate of 0.02 deg s−1.
2.2.1 Synthesis of Fe3O4. In a typical experiment, 2.0 g of poly(4-styrenesulfonic acid-co-maleic acid) sodium salt (PSSMA) was dissolved in ethylene glycol (80 mL) under magnetic stirring to form a clear solution, followed by the addition of FeCl3·6H2O (2.16 g) and sodium acetate (6.0 g). The obtained solution was then sealed in a Teflon-lined stainless-steel autoclave and heat at 200 °C for 10 h. After the reactants were cooled to room temperature, Fe3O4 microspheres were isolated by a magnet and washed at least three times with ethanol and deionized water, and then dried in vacuum for 12 h.18
2.2.2 Chemical modification of Fe3O4. For silica coating, the washed Fe3O4 were dispersed in water (24 mL). Then 4 mL of the Fe3O4 water dispersion was added to a solution containing ethanol (80 mL) and water (4 mL). The mixture was ultrasonicated for 15 min, prior to the addition 50 of ammonia hydroxide solution (4 mL). Then TEOS (0.8 mL) in ethanol (20 mL) was added into the above dispersion, the reaction was performed for another 90 min. Then the products were collected by a magnet and washed three times with ethanol and water.18Synthesis of Fe3O4@MPS nanoparticles followed the below procedure:19 Briefly, 300 mg of Fe3O4@SiO2 was added in 90 mL of toluene, sonicated for 10 min, and stirred vigorously. The mixing solution of 5 mL of MPS and 10 mL of toluene was then dropped into the resulting solution for 30 min. The stirring continued under a nitrogen atmosphere at 65 °C for 24 h. The products were collected by a magnet, rinsed with methanol several times thoroughly, and dried under vacuum.
2.2.3 Synthesis of imprinted and nonimprinted polymers. The thermosensitive magnetic molecularly imprinted polymers were prepared as follows. First, the template SMZ (1 mmol) and functional monomers MAA (2 mmol), NIPAM (2 mmol) were dissolved in 50 mL of acetonitrile and then the solution was put in a refrigerator for 12 h to obtain the template–monomer complex. Then, 250 mg Fe3O4@MPS and EGDMA (20 mmol) were dissolved in 50 mL of acetonitrile by sonication for 10 min, and then, the preassembly solution was added to the above solution. After purging with nitrogen for 15 min, AIBN (0.1 g) was added to the reaction system as an initiator. To obtain a high crosslinking density, prepolymerization was undertaken at 50 °C for 6 h and then stirred at 60 °C for 24 h. Finally, the resultant products were washed with methanol, and dried under vacuum. Then, the TMIPs were eluted with methanol/acetic acid (9
:
1 v/v) in a Soxhlet extraction apparatus to remove the template. The NIPs were synthesized by a similar method except for the absence of SMZ in the polymerization mixture.
2.3 Measurement of equilibrium swelling ratio
In order to investigate the thermoresponsive properties of the imprinted polymers, swelling ratios at different temperatures were measured using the gravimetric method. First, certain amounts of TMIPs/TNIPs were dried under vacuum to obtain the dry weight (Wd). Then these samples packaged by filter paper and the other single filter paper were incubated in phosphate buffer (10 mmol L−1, pH = 7.0) for 6 h at 15, 20, 25, 30, 35, 40 and 50 °C, respectively. After equilibrium, the samples packaged by filter paper and the single filter paper were weighed, respectively. And the difference value between them was recorded as the wet weight of the samples (Wt). Then, the swelling ratio was calculated with the following formula:21
Swelling ratio = (Wt − Wd)/Wd |
2.4 Adsorption experiments
Adsorption isotherms experiments were conducted: 10 mg of TMIPs or TNIPs were added into the centrifuge tubes containing 5 mL of methanol solution with SMZ concentration varying from 0.1 to 0.4 mM and the centrifuge tubes were shaken for 5 h at 35 °C. After magnetic separation, the SMZ concentration in the supernatant was measured by HPLC at 270 nm. The equilibrium adsorption capacities of TMIPs or TNIPs for the template SMZ were calculated by the following formula:
where C0 and C1 are the initial SMZ concentration and final SMZ concentration in the supernatants (mM), V is the volume of the SMZ solution (mL), m is the weight of TMIPs or TNIPs (mg).
In order to study the effect of temperature on the capture of SMZ, a series of experiments were carried out with temperature ranging from 25 to 40 °C.
Desorption capacities of the TMIPs or TNIPs were investigated at 15 °C to further study the thermosensitive property of the polymers. Briefly, 5.00 mL eluant of methanol–acetic acid (90
:
10, v/v) was added to centrifuge tubes containing the fully loaded polymers, and the centrifuge tubes were shaked for 2 h to remove the template molecules. After magnetic separation, the concentration of SMZ in the supernatant solution was measured by HPLC at 270 nm.
3. Results and discussion
3.1 Preparation of thermosensitive magnetic imprinted polymers
The synthesis schematic diagram of the thermosensitive magnetic imprinted polymers was illustrated in Fig. 1, including the following steps: synthesis of Fe3O4, modification of Fe3O4 with silica coatings, functionalization of Fe3O4@SiO2 with vinyl groups, and polymerization in the presence of crosslinking agent, initiator and porogen after the self-assembly of the template and functional monomers. Silica was selected to encapsulate Fe3O4 nanoparticles in order to increase the dispersibility of magnetic nanoparticles and its bioconjugation with various groups.19 Then, the vinyl groups were grafted on the surface of the Fe3O4@SiO2 through the reaction between MPS and silanol group. The vinyl groups reacted with the functional monomer MAA, which made the polymer layers more easily encapsulated on the nanoparticles.
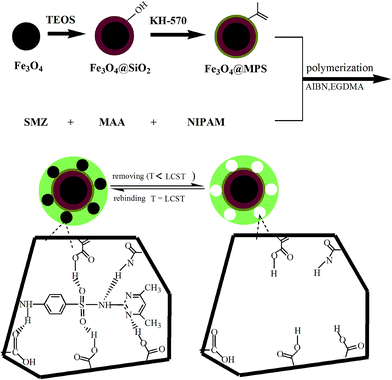 |
| Fig. 1 Schematic illustration of the preparation of TMIPs. | |
The adsorption capabilities of the TMIPs would be affected by many factors. In this work, the type of porogen and the proportion of the functional monomers as the influence factors were discussed. The driving forces for the TMIPs to capture SMZ were hydrogen bonding and hydrophobic interaction.23 The solvents had the influence on the formation of hydrogen bonds.22 So, acetonitrile and methanol were selected for comparison, both of which could dissolve the template molecule and functional monomers. The corresponding results are displayed in Fig. S1.† Finally, acetonitrile was chosen as solvent in the synthesis experiments due to its weak polarity because the strongly polar solvent would weaken the formation of hydrogen bonds. As we all known, too many excessive functional monomers may lead to extensive nonspecific recognition sites. Thus, in the following experiments, the ratio of functional monomers and the template was fixed at 4
:
1.24 But in order to seek the optimal imprinted polymers, five kinds of TMIPs differing in the proportion of the functional monomers were prepared. Their corresponding adsorption capacities for SMZ are displayed in Fig. S2.† Among these TMIPs, MIP2 showed the greatest binding capacity for SMZ, which was therefore selected for further investigation.
3.2 Characterizations of thermosensitive magnetic imprinted polymers
The TEM images revealed that the diameter of the Fe3O4 was about 150 nm with an regular shape. And from Fig. 2A we can see that these sub-micrometer sized clusters were formed by the aggregation of many small nanocrystals with sizes of 5–8 nm.18 Fig. 2B showed that the Fe3O4 was successfully coated with silica and the thickness of SiO2 layers was about 18 nm. Then the Fig. 2C showed that the thickness of the polymer layers for the TMIPs was about 15 nm, revealing that the binding sites almost existed at the surface of the TMIPs. And this could be the most important reason for fast rebinding and releasing the template.
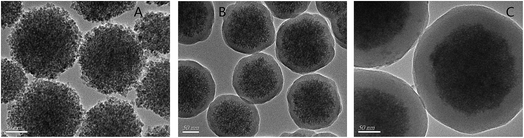 |
| Fig. 2 TEM images of Fe3O4 (A), Fe3O4@SiO2 (B), and TMIPs (C). | |
The FT-IR spectrum of Fe3O4, Fe3O4@SiO2, Fe3O4@MPS, TMIPs were compared in Fig. 3. In Fig. 3A, the peak at 580.71 cm−1 was characteristic of the Fe–O stretching vibration for Fe3O4 magnetic nanoparticles. In Fig. 3B, the peaks around 801.65 cm−1 was characteristic of the Si–O–Si symmetric stretching vibration and the peaks around 953.39 cm−1, 1090.25 cm−1 were attributed to the Si–O–H stretching vibrations and Si–O–Si asymmetric stretching vibration, respectively. All of these meant that the silica was successfully encapsulated on the surface of Fe3O4. Meanwhile, in Fig. 3C, the strong peaks of the C
O group at 1709.10 cm−1,carbonylic groups vibration at 1649.59 cm−1 and C–H stretching vibration in methylene group at 2976.53 cm−1 indicated the double bonds were successful grafted on the vinyl-modified Fe3O4@SiO2. In Fig. 3D and E, the FT-IR spectra of TMIPs without the template was almost consistent with the FT-IR spectra of TNIPs.
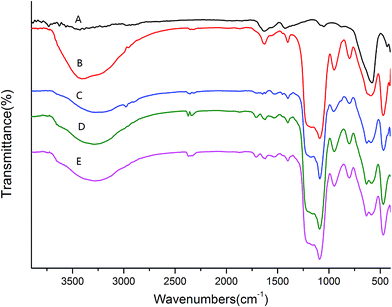 |
| Fig. 3 FT-IR spectra of the Fe3O4 (A), Fe3O4@SiO2 (B), Fe3O4@MPS (C), TMIPs (D) and TNIPs (E). | |
Fig. S3† showed the XRD patterns of the products. These samples had similar diffraction patterns and the positions of the peaks remained unchanged, which meant that the crystalline structure of Fe3O4 did not been changed in each synthesized process. And all of the diffraction peaks could be indexed to the face center cubic structures of magnetite (JCPDS no. 19-0629).
The reason to choose magnetic nanoparticles as the carrier was due to the magnetic characteristic, which could make the materials separated from the solution quickly. Therefore, it was important and necessary to test the VSM to characterize the magnetic properties of the materials. Fig. 4 showed that the saturation magnetization values of Fe3O4, Fe3O4@SiO2, Fe3O4@MPS and TMIPs were 59 emu g−1, 42 emu g−1, 31 emu g−1, and 27 emu g−1, respectively. Although the saturation magnetization of TMIPs was much lower than that of Fe3O4 due to the step by step modification, TMIPs also had enough magnetic to be separated with the external magnet.
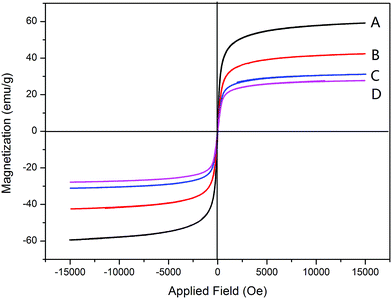 |
| Fig. 4 Hysteresis loops of Fe3O4 (A), Fe3O4@SiO2 (B), Fe3O4@MPS (C) and TMIPs (D). | |
3.3 Thermoresponsive properties of thermosensitive magnetic imprinted polymers
Swelling experiments were investigated at different temperatures in order to research the relative hydrophilicity of the prepared materials. As displayed in Fig. 5, the swelling ratio of the TMIPs and that of the TNIPs showed the similar variation trend. The swelling ratios of them were relative high and stable when the temperature was below 30 °C, which indicated that the TMIPs and the TNIPs had strong hydrophilicity at low temperature. However, the swelling ratios of them exhibited a dramatic decline with the temperature ranging from 30 °C to 40 °C, which could be explained by the decreased hydrophilicities of the polymers along with the volume phase transitions. The change of hydrophilicity resulted from the existence of temperature-sensitive monomer NIPAM.25 When the temperature was above 40 °C, the swelling ratios of them were low, because the hydrophobicity increased. The results showed that such a stimuli-responsive property was good for the imprinted polymers to modulate the capture and release process.
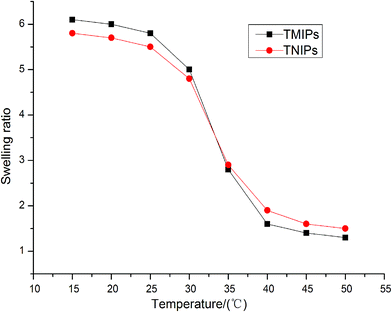 |
| Fig. 5 Swelling ratio curves of the prepared adsorption materials. | |
3.4 Effect of temperature on the adsorption of TMIPs
The thermosensitive polymers can undergo reversible volume transitions between the swollen and the collapsed phases, which can be triggered by external temperature. Thus, the effect of temperature on the adsorption of TMIPs and TNIPs were investigated. As shown in Fig. 6, the adsorption capacities of the TMIPs first increased and then decreased with the temperature ranging from 25 °C to 40 °C. This phenomenon was related to the volume change of imprinting cavities. When the temperature was below 35 °C, the polymers were hydrophilic and the imprinting cavities swelled. When the temperature was above 35 °C, although the hydrophobic interactions between the PNIPAm chains and the template increase, the polymers gradually shrunk with the volume change of PNIPAm. Meanwhile, the adsorption capacities of the TMIPs reached the maximum around 35 °C, in which state the shape of the cavities would accord with the template accurately, resulting in the relatively well adsorption performance. For the TNIPs, the adsorption capacities increased slightly along with the increase of temperature. This might be the reason that the temperature did not influence the nonspecific interactions greatly. So, the following adsorption experiments would be carried out at 35 °C.
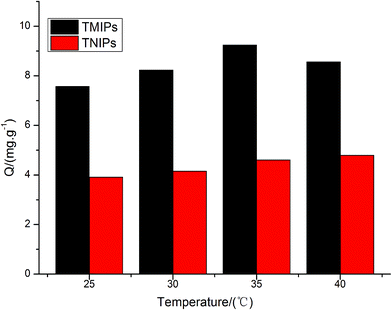 |
| Fig. 6 Effect of temperature on adsorption capacity (Q) of TMIPs and TNIPs. | |
3.5 Adsorption experiments
3.5.1 Analysis of the adsorption kinetics. The following adsorption kinetics of the TMIPs and TNIPs were investigated at 0.2 mM SMZ. As shown by the kinetic curves Fig. 7A, the adsorption capacities of the TMIPs leveled off and remained constant after 80 min, which indicated that most of the imprinted sites were saturated with the templates. Most of the templates were adsorbed to the binding sites at a fast rate, suggesting that the imprinted sites of the magnetic MIPs were on or near the surface. At the same time, the TNIPs showed a low affinity but with the similar variation trend of TMIPs as an obvious increasement in amount adsorption from 0 to 80 min.
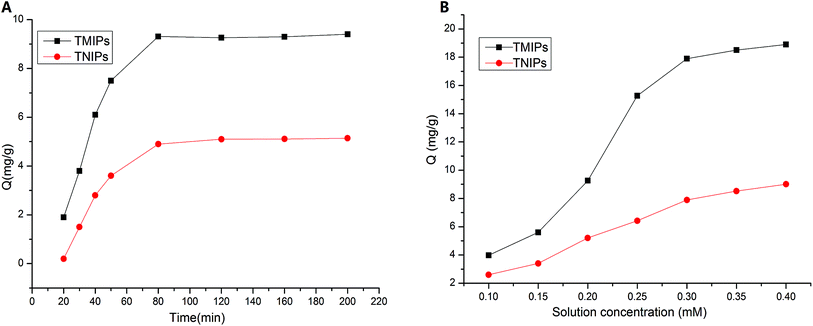 |
| Fig. 7 Adsorption kinetics (A) and adsorption isotherms (B) of TMIPs and TNIPs. | |
3.5.2 Analysis of the adsorption isotherms. Seen from the binding isotherm curves Fig. 7B, the adsorption capacities of both TMIPs and TNIPs for the templates increased with the increment of the templates initial concentration. The TMIPs exhibited much higher adsorption capacities of the templates compared with the TNIPs. The adsorption capacities of TMIPs were about 2-fold over that of TNIPs at 0.4 mM of SMZ. Because specific recognition sites were formed in the synthesis process of TMIPs, but imprinting sites matched with SMZ did not exist in imprinting shell of TNIPs and the distribution of functional groups on the surface of NIPs was random, which led to few effective binding sites. All of these could proved that the high adsorption capacities of the TMIPs were due to the imprinting effect.In order to further estimate the binding properties of TMIPs, the saturation binding datas were fitted to the equation as follows:
where
Q and
Qmax represented the experimental adsorption capacity and the theoretical maximum adsorption capacity of the polymers (mg g
−1) to the templates, respectively.
C was the free concentration of SMZ at equilibrium in the solution and
Kd is the dissociation constant.
According to Langmuir model, the adsorption occurred uniformly on the active sites of the polymers.26 Once a template occupied a binding site, no further adsorption could take place at this site. It was observed that the experimental datas were fitted to the Langmuir adsorption isotherm model in Fig. S5.† The adsorption process of SMZ onto TMIPs could be considered monolayer adsorption. And the linear regression equation was C/Q = 0.03771X + 1.0074 (r = 0.9872). From the slope and the intercept of this plot, the values of Kd and Qmax were calculated to be 0.037 L mg−1 and 26.5 mg g−1.
3.5.3 Analysis of the binding specificity. Specific adsorption experiments were conducted in order to prove that the TMIPs had selectivity for SMZ due to the tailor-made cavities. SMO and SDZ as the structural analogues of SMZ and DBP as dissimilar structure compound were chosen in this part. The structures of the three sulfonamides were analogous, just differing in the size of the R-group. From Fig. 8, the TMIPs exhibited significantly higher binding capacities toward SMZ than the others, suggesting that the template molecule SMZ had a relatively higher affinity for the imprinted polymers. The specificity of the TMIPs relied on the conformational memory of imprinting cavities formed in the imprinting process. There were interactions of hydrogen bonding between the hydroxyl group in MAA and sulfonyl group in SMZ. The binding amounts of the imprinted polymers for the structural analogues were relative higher than that of DBP, because both the structural analogues also have the group –S(
O)2−. SDZ was similar to SMZ in molecular structure, just with the absence of two methyl groups in R-group, which could make SDZ suffer less steric hindrance due to its simple structure. The binding amounts of TMIPs for the SMO were low. This may be the reason that the difference in the structure and size hindered SMO from being captured. The TMIPs had nearly no selective adsorption to DBP, which might result from the imprinting effect. Obviously, the adsorption capacities of the TMIPs to these compounds were much higher than that of the TNIPs, respectively.
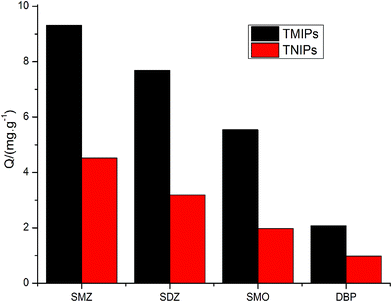 |
| Fig. 8 The specific recognition capability of TMIPs and TNIPs for SMZ and other interferences. | |
3.6 Desorption
The imprinted polymers became hydrophilic and swollen at low temperature, so the hydrophobic effect and hydrogen bonding between the TMIPs and the templates were weakened, which could prompt the release of SMZ. The results were shown in ESI Fig. S4,† the desorption rate of the fully loaded TMIPs reached 93.5% with only once washing operation at 15 °C. The results showed that this kind imprinted polymers had excellent desorption ability due to the thermoresponsive performance, which offered great superiority to the reusability. At the same time, we found that the TMIPs released more SMZ than TNIPs. This may be the reason that the nonspecific reactions were not deeply affected by temperature.
3.7 Reusability
Reusability is an important parameter to evaluate the prepared polymers for the application. Seen from Fig. 9, after adsorption and desorption for five cycles, the average recovery could reach 89.6% and only 10.4% loss was observed for the binding capacity of TMIPs. The results showed that the thermosensitive imprinted polymers had good reusability just by altering the temperature in this study. What's more important, the transition between swollen and collapsed phases was reversible. This way to release the templates had enough advantages than the monotonous elution only with detergents repeatedly, which usually blocked the active sites and led to poor reusability.27
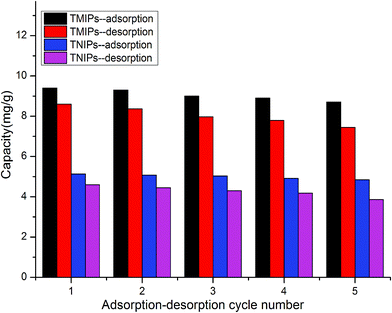 |
| Fig. 9 Reusabilities of TMIPs and TNIPs. | |
3.8 Determination of SMZ in the real-water samples
The feasibility of using the prepared TMIPs to selectively enrich the SMZ in real water was examined. In this study, the real water was collected from Yudai River in Jiangsu University. After precipitation for about 30 min, the real water was filtered with a 0.45 μm filter membrane prior to use. From Fig. 10A, we could see that there was no peak of SMZ in the blank real water sample. Then the real water sample was spiked with 10 mg L−1 SMZ. From Fig. 10B, there was obvious peak of SMZ. And the peak areas of other distractors were almost invisible because the base line of the chromatogram was smooth. The peak of SMZ appeared to be weak again in Fig. 10C, which meant that the templates had been selectively captured. The recoveries of SMZ in the real water samples ranged from 83.2% to 96.8%. The RSDs were less than 6.3%. All of the results revealed that the TMIPs could be applied to the selective extraction, enrichment, and determination of SMZ in real-water samples.
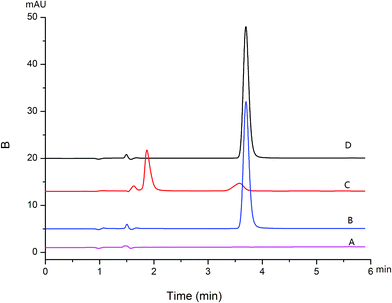 |
| Fig. 10 HPLC chromatograms of the blank real water samples (A), real water samples spiked with 10 mg L−1 SMZ (B), SMZ remaining in supernatant solution after selective recognition by TMIPs (C), SMZ in release solution (D). | |
4. Conclusion
In this study, thermosensitive magnetic molecular imprinted polymers were prepared by copolymerization of NIPAM, MAA and AIBN on the surface of Fe3O4@MPS microspheres in the presence of template SMZ. The TMIPs exhibited excellent selectivity and adsorption capacities for the template molecule. The effect of temperature on the adsorption capacity of TMIPs was also investigated, and the results proved that the TMIPs could undergo a reversible volume transition between the swollen and collapsed phases with changing of the temperature. Moreover, the separation was simplified due to the magnetic force of the TMIPs. Thus, the results exhibited its potential application in the recognition, extraction and separation of SMZ from the real samples. In the following work, we would further optimize the experimental conditions to enhance the imprinting efficiency, such as imprinting factor and specific rebinding capacity.
Acknowledgements
This work was sponsored by the Jiangsu Natural Science Foundation of China (No. BK20151323, BK20151337), Postdoctoral Science Foundation of China (No. 2014M560405, 2015T80515), Postdoctoral Science Foundation of Jiangsu Province (No. 1401012A), Jiangsu Collaborative Innovation Center of Technology and Material of Water Treatment.
References
- X. D. Mao and H. Y. Sun, Well-defined sulfamethazine-imprinted magnetic nanoparticles via surface-initiated atom transfer radical polymerization for highly selective enrichment of sulfonamides in food samples, Anal. Methods, 2015, 7, 4708 RSC.
- J. L. Martinez, Antibiotics and antibiotic resistance genes in natural environments, Science, 2008, 321, 365 CrossRef CAS PubMed.
- W. H. Xu, G. Zhang and X. D. Li, Occurrence and elimination of antibiotics at four sewage treatment plants in the Pearl River Delta, South China, Water Res., 2007, 41, 4526 CrossRef CAS PubMed.
- G. Wulff, Molecular imprinting in cross-linked materials with the aid of molecular templates A way towards artificial antibodies, Angew. Chem., Int. Ed. Engl., 1995, 34, 1812 CrossRef CAS.
- T. P. Rao, S. Daniel and J. M. Gladis, Tailored materials for preconcentration or separation of metals by ion-imprinted polymers for solid-phase extraction, TrAC, Trends Anal. Chem., 2004, 23, 28 CrossRef.
- K. Haupt and K. Mosbach, Molecularly imprinted polymers and their use in biomimetic sensors, Chem. Rev., 2000, 100, 2495 CrossRef CAS PubMed.
- F. Puoci, G. Cirillo and M. Curcio, Molecularly imprinted polymers in drug delivery: state of art and future perspectives, Expert Opin. Drug Delivery, 2011, 8, 1379 CrossRef CAS PubMed.
- Y. R. Zhao and C. F. Bi, Preparation of molecularly imprinted polymers based on magnetic carbon nanotubes for determination of sulfamethoxazole in food samples, RSC Adv., 2015, 5, 70309 RSC.
- K. Aguilar-Arteaga, J. A. Rodriguez and E. Barrado, Magnetic Solids in Analytical Chemistry: A Review, Anal. Chim. Acta, 2010, 674, 157 CrossRef CAS PubMed.
- A. Bossi and F. Bonini, Molecularly Imprinted Polymers for the Recognition of Protein: The State of Art, Biosens. Bioelectron., 2007, 22, 1131 CrossRef CAS PubMed.
- Y. Lv, T. Tan and F. Svec, Molecular Imprinting of Proteins in Polymers Attached to the Surface of Nanomaterials for Selective Recognition of Biomacromolecules, Biotechnol. Adv., 2013, 31, 1172 CrossRef CAS PubMed.
- D. R. Kryscio and N. A. Peppas, Critical review and perspective of macromolecularly imprinted polymers, Acta Biomater., 2012, 8, 461 CrossRef CAS PubMed.
- X. W. Kan, Z. L. Xing and A. H. Zhu, Molecularly imprinted polymers based electrochemical sensor for bovine hemoglobin recognition, Sens. Actuators, B, 2012, 168, 395 CrossRef CAS.
- W. Zhang, X. W. He, Y. Chen, W. Y. Li and Y. K. Zhang, Molecularly imprinted polymer anchored on the surface of denatured bovine serum albumin modified CdTe quantum dots as fluorescent artificial receptor for recognition of target protein, Biosens. Bioelectron., 2012, 31, 84 CrossRef CAS PubMed.
- X. Kong and R. X. Gao, Synthesis and characterization of the core–shell magnetic molecularly imprinted polymers (Fe3O4@MIPs) adsorbents for effective extraction and determination of sulfonamides in the poultry feed, J. Chromatogr. A, 2015, 1245, 8 CrossRef PubMed.
- G. Pan, Q. Guo, C. Cao and H. Yang, Thermo-Responsive Molecularly Imprinted Nanogels for Specific Recognition and Controlled Release of Proteins, Soft Matter, 2013, 9, 3840 RSC.
- Z. Hua, Z. Chen, Y. Li and M. Zhao, Thermosensitive and Salt Sensitive Molecularly Imprinted Hydrogel for Bovine Serum Albumin, Langmuir, 2008, 24, 5773 CrossRef CAS PubMed.
- J. Gao, X. Z. Ran and C. M. Shi, One-step Solvothermal Synthesis of Highly Water-soluble, Negatively Charged Superparamagnetic Fe3O4 Colloidal Nanocrystal Clusters, Nanoscale, 2013, 15, 7026 RSC.
- S. S. Miao and M. S. Wu, Core–Shell Magnetic Molecularly Imprinted Polymers as Sorbent for Sulfonylurea Herbicide Residues, J. Agric. Food Chem., 2015, 63, 3634 CrossRef CAS PubMed.
- X. J. Li and B. L. Zhang, Preparation and characterization of bovine serum albumin surface imprinted thermosensitive magnetic polymer microsphere and its application for protein recognition, Biosens. Bioelectron., 2014, 51, 261 CrossRef CAS PubMed.
- P. F. Ma and Z. P. Zhou, Preparation and application of sulfadiazine surface molecularly imprinted polymers with temperature-responsive properties, J. Appl. Polym. Sci., 2015, 41769, 1 Search PubMed.
- J. X. He and S. Wang, Molecularly Imprinted Polymer Online Solid-Phase Extraction Coupled with High-Performance Liquid Chromatography-UV for the Determination of Three Sulfonamides in Pork and Chicken, Food Chem., 2008, 56, 2919 CrossRef CAS PubMed.
- L. G. Chen and X. P. Zhang, Fast and Selective Extraction of Sulfonamides from Honey Based on Magnetic Molecularly Imprinted Polymer, Agric. Food Chem., 2009, 57, 10073 CrossRef CAS PubMed.
- G. Vasapollo and R. D. Sole, Molecularly imprinted polymers: present and future prospective, Int. J. Mol. Sci., 2011, 12, 5908 CrossRef CAS PubMed.
- S. Bai, H. Zhang, J. Sun and J. Han, Preparation and pH-Responsive Performance of Silane-Modified Poly(methylacrylic acid), J. Appl. Polym. Sci., 2014, 131 Search PubMed.
- R. T. Ma and Y. P. Shi, Magnetic molecularly imprinted polymer for the selective extraction of quercetagetin from Calendula officinalis extract, Talanta, 2015, 134, 650 CrossRef CAS PubMed.
- R. Schirhagl, Bioapplications for Molecularly Imprinted Polymers, Anal. Chem., 2014, 86, 250 CrossRef CAS PubMed.
Footnote |
† Electronic supplementary information (ESI) available. See DOI: 10.1039/c6ra16162f |
|
This journal is © The Royal Society of Chemistry 2016 |