DOI:
10.1039/C6RA15605C
(Paper)
RSC Adv., 2016,
6, 79830-79835
An A–π–A′ structural ratiometric fluorescent probe based on benzo[e]indolium for bisulfite and its application in sugar samples and living cells†
Received
15th June 2016
, Accepted 13th August 2016
First published on 19th August 2016
Abstract
An A–π–A′ structural ratiometric fluorescent probe 1 bearing a benzo[e]indolium moiety was developed for the detection of HSO3− in PBS buffer solution. HSO3− was expected to react with probe 1 via a Michael addition reaction, causing dramatic changes in its emission spectrum (from 578 nm to 466 nm) and an obvious color change from orange red to blue which could be observed by the naked eye. The reaction could be complete in 30 seconds in the sensing system, and displayed a high selectivity and sensitivity for HSO3− with a detection limit of 15 nM. The practical value of the probe was confirmed for application to detect the level of HSO3− in sugar samples with good recovery and in living cells.
1. Introduction
Bisulfite (HSO3−), as an effective preservative, has been widely used in foods, beverages and pharmaceutical products.1 It has been reported that extensive HSO3− intake would cause asthmatic attacks and allergic reactions, such as difficulty in breathing, wheezing, hives, and gastrointestinal distress in some individuals.2,3 Due to the above mentioned harmful effects towards people, the threshold levels of HSO3− in food and medicine have been controlled rigorously in many countries. For instance, the total content of sulfur (calculated by SO2) in white granulated sugar is strictly regulated as <30 mg kg−1 in China.4 Hence, considerable attention has been attracted to the development of rapid, facile, and reliable techniques for HSO3− detection because of the food quality assurance and human healthy.
Fluorescent probes have become powerful tools for sensing and imaging trace amounts of samples due to their simplicity, sensitivity, low-toxicity and easy of operation in recent years.5,6 To date, some fluorescent probes have been developed for monitoring HSO3− on the basis of complexation with amines,7,8 the selective de-protection of a levulinate group,9–12 selective nucleophilic reaction with aldehyde13–20 and N
N in metal complexes,21,22 and Michael-type additions.23–39 However, most of these probes may suffer from the risk of slow response time (2 min to 10 h) or an organic co-solvent, which limited their utility. Recently, Song and co-workers reported a fluorescent turn-on probe and a ratiometric fluorescent probe, respectively.31,32 Both of them were based on a near-infrared benzopyrylium dye which could detect HSO3− in aqueous solution (HEPES, pH = 7.4) with an incredible response time (<15 s) and a satisfactory detection limit. Compared to the intensity-based fluorescent probes, the ratiometric fluorescent probes enable the measurement of emission intensities at two different wavelengths, which increase the dynamic range and provide a built-in correction for environmental effects.40 Thereby, it has remained a great challenge to construct new sensitive and selective ratiometric fluorescent probes for the rapid HSO3− detection in pure aqueous environments and in real samples.
Herein, we introduce a new ratiometric fluorescent probe 1 which is constructed by a benzo[e]indolium fluorophore (an electron acceptor A) and a malononitrile group (another electron acceptor A′), that is, an A–π–A′ type compound. The A–π–A′ type compound could effectively avoid the severe emission quenching which were frequently encountered in D–π–A type compounds owing to dipole-induced intermolecular interactions.41 Probe 1 has shown a selective response to HSO3− over other anions in PBS buffer (pH = 7.4, 10 mM), and a distinguished fluorescent color change from orange red to cyan can be observed. HSO3− is expected to undergo a Michael addition reaction with the C-4 atom in the ethylene group, which is activated by the electron-withdrawing feature of the positively-charged benzo[e]indolium fragment and the malononitrile group (Scheme 1).
 |
| Scheme 1 Probe 1 and its synthesis route. | |
2. Experimental
2.1 Materials and chemicals
All reagents and solvents were purchased from commercial source and used without further purification, if not stated. All reactions were carried out on the magnetic stirrers and their reaction process was monitored on thin layer chromatography (TLC). Stock solutions (100 mM) of HSO3−, HS−, F−, Cl−, Br−, I−, AcO−, ClO4−, NO3−, N3−, SO42−, HSO4−, SCN−, PO43−, HPO42−, H2PO4−, Cys, Hcy, and GSH were prepared by direct dissolution of proper amounts of their sodium salts. Absorption and fluorescence spectra were taken on a Shimadzu UV-1800 spectrophotometer and a Hitachi F-2700 fluorescence spectrometer, respectively. 1H NMR and 13C NMR measurements were recorded at 600 and 150 MHz on a Brucker Avance 600 MHz spectrometer. Dimethyl sulfoxide (DMSO-d6) was solvent, and tetramethylsilane (TMS) was used as internal standard. HRMS (ESI) were taken on a Fourier transform ion cyclotron resonance mass spectrometry (Varian 7.0T). An Olympus FV-1000 confocal fluorescence microscope and HepG2 cells were used in the living cell experiment.
2.2 Preparation of probe 1
In a 25 mL round-bottom flask, terephthalaldehyde (200 mg, 1.49 mmol) and malononitrile (98 mg, 1.49 mmol) were dissolved in ethanol (15 mL) and the mixture was stirred at 80 °C for 12 h. After cooling to room temperature, the precipitate was collected by filtration, washed with cold ethanol to afford the crude product. The pure product was obtained by further purification using silica gel chromatography as a light yellow solid 2 (190 mg, 70%). 1H NMR (600 MHz, CDCl3) δ 9.87 (s, 1H), 8.65 (s, 1H), 8.48 (d, 2H), 8.45 (d, 2H).
To a solution of 1, 2, 3, 3-tetramethylbenz[e]indolium iodide (70 mg, 0.2 mmol) in ethanol (10 mL) was added the prepared 2 (36 mg, 0.2 mmol) and the solution was refluxed for 12 h. After cooling to room temperature, the precipitate was collected by filtration, washed with cold ethanol. The pure product was obtained by recrystallization from ethanol as dark red solid (88 mg, 77%). 1H NMR (600 MHz, DMSO-d6): δ 8.65 (s, 1H), 8.49 (m, 4H), 8.35 (d, 1H), 8.25 (d, 1H), 8.17 (d, 1H), 8.12 (d, 2H), 7.90 (d, 1H), 7.88 (t, 1H), 7.76 (t, 1H), 4.34 (s, 3H), 2.03 (s, 6H). 13C NMR (150 MHz, DMSO-d6): δ 186.0, 176.5, 167.2, 152.5, 142.4, 141.9, 137.8, 134.4, 134.0, 131.8, 131.0, 128.9, 127.2, 118.8, 116.9, 57.6, 39.2, 28.0. HRMS (ESI) calcd. for [M]+ 388.1806, found 388.1808 (Fig. S1–S3†).
2.3 Preparation of solutions of probe 1 and analytes
Deionized water was used as the solvent for titration experiment. The titrations were carried out in 10 mm quartz cuvettes at room temperature. Probe 1 was dissolved in DMSO (spectroscopic grade) to afford a concentration of 10 mM stock solution, and then diluted with PBS buffer (pH = 7.4, 10 mM) to a concentration of 20 μM. Anions (as their Na+ salt, 10 mM and 100 mM) in deionized water were added to the diluted probe solution and used for the sensing behavior experiment. The resulting solution was shaken well and kept at room temperature for 30 seconds before measuring the spectra. The excitation wavelength was 400 nm, and the PMT voltage was 400 V. The excitation and emission slit width were 5 nm and 5 nm, respectively.
2.4 1H NMR analysis experiments of probe 1
The solution of probe 1 (3 × 10−3 M) in DMSO-d6 (450 μL) and D2O (50 μL) was placed in the NMR tube, and sodium bisulfite powder was added. All the sodium bisulfite was soluble.
2.5 Sugar samples test
Granulated sugar, soft sugar and crystal sugar purchased from supermarket were used in the sample analysis. Sample solution was prepared by dissolving 5.0 g of sugar in deionized water and diluting to 10 mL. Aliquots of the sugar solution were added directly to the PBS buffer (pH = 7.4, 10 mM) containing probe 1 (20 μM), and the emission intensity at 466 nm and 578 nm were recorded.
2.6 Preparation of test papers and detection
The neutral filter papers were dipped in the stock solution of 1 (10 mM) and dried. Then various solutions of anions (1 mM, 2.5 μL) were seriatim dropped. The concentrations of sugar samples for test paper experiment were 1, 0.7, 0.4, 0.1 g mL−1.
2.7 Cell incubation and fluorescence imaging
The HepG2 cells were provided by of Shanxi Medical University. Cells were seeded onto the cover slips and cultured in DMEM in an incubator (37 °C, 5% CO2 and 20% O2). After 24 hours, the cover slips were washed 3 times with PBS to remove the media and then cultured in PBS for use. Probe 1 (10 μM) was added to above cellular samples and incubated for 15 min, and then washed 3 times with PBS. In a further experiment, HSO3− (15 μM) was added to the cells and the fluorescence change was observed under the confocal fluorescence microscope.
2.8 Cytotoxicity assays
HepG2 cells were seeded onto the cover slips and cultured in DMEM in an incubator (37 °C, 5% CO2 and 20% O2). Before the experiment, the cells were placed in a 96-well plate, followed by addition of various concentrations of probe 1. Then the cells were incubated at 37 °C in an atmosphere of 5% CO2 and 20% O2 for 12 hours, followed by MTT assays (n = 5).
3. Results and discussion
3.1 Spectral studies of probe 1
The detection environment was investigated at first. As shown in Fig. S4,† the probe was stable in a pH range of 1–8 and displayed the satisfied response for HSO3− in the physiological pH region. Thus, the UV-vis absorption and emission spectra of probe 1 towards HSO3− were measured in PBS buffer (pH = 7.4, 10 mM). Fig. 1 shows the absorption (a) and emission (b) responses of probe 1 (20 μM) with different amounts of HSO3− (0–60 μM). Probe 1 exhibited a yellow color with an obvious band at 380 nm and a shoulder band at 445 nm (Fig. 1a), which was ascribed to the typical absorption induced by the π–π conjugation. Upon addition of HSO3−, the absorption bands were gradually attenuated and a new band at 268 nm was appeared, suggesting that the π–π conjugation is interrupted due to the nucleophilic attack of HSO3−. The addition also induced an obvious color change from yellow to colorless (inset of Fig. 1a), which could be observed by naked eye. Gratifyingly, the free probe 1 showed an emission band centered at 578 nm. In contrast, upon addition of HSO3− (Fig. 1b), the emission band at 578 nm was decreased and a new band at 466 nm increased remarkably, which could be attributed to the formation of 2-methylene benzo[e]indoline moiety. A well-defined isoemissive point at 536 nm was also observed. The ratiometric change in fluorescence intensity indicated that the addition reaction between probe 1 and HSO3− interrupted the π-conjugation, and the fluorescence of 2-methylenebenzo[e]indoline was recovered. Accordingly, the fluorescence color changed from orange red to cyan (inset of Fig. 1b). The distinct emission shift (about 112 nm) was favorable for ratiometric fluorescence detection. Moreover, the ratio of the emission intensities at 466 and 578 nm (I465/I571) was increased (25-fold, from 0.10 to 2.5) with the addition of HSO3− and became constant when the amount of HSO3− added reached 2.4 equiv. (48 μM) (Fig. S5†). Under similar conditions, the ratio changes produced an excellent linear function with HSO3− concentration between 0 and 24 μM, and the detection limit for HSO3− was determined to be 0.15 μM (R2 = 0.9930) based on S/N = 3 (Fig. S6†), which is sufficient to probe the HSO3− concentration in cells (ca. 16 μM).23
 |
| Fig. 1 Absorption (a) and emission spectra (b) of probe 1 (20 μM) in PBS buffer (pH 7.4, 10 mM) treated with HSO3− and their photographs in the absence or presence of HSO3− (60 μM) under sunlight (a) and UV light (b, 365 nm). λex = 400 nm. Slits: 5 nm/5 nm. The concentrations of HSO3− were varied in the following order: 0, 4, 8, 12, 16, 20, 24, 28, 32, 36, 40, 44, 50 and 60 μM. | |
To further evaluate the specific nature of probe 1 towards HSO3−, the influence of other anions were examined under the same conditions. As shown in Fig. 2 and S7,† after adding all the other anions into the solution of probe 1 (20 μM), they did not induce any significant fluorescence change, and the I465/I571 values varied in a limited range between 0.12 and 0.25. By contrast, treatment of probe 1 with HSO3− resulted in an obvious ratiometric fluorescent response. Competition experiments of probe 1 were also determined by various species mentioned previously in the same condition. The signaling of probe 1 toward HSO3− was not affected by the presence of 60 μM of coexisting species (Fig. S8†). The phenomenon suggests that probe 1 could be used as an efficient signaling probe for HSO3− detection in aqueous media.
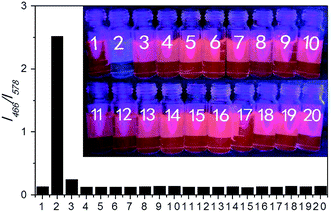 |
| Fig. 2 Emission ratio I466/I578 of 1 (20 μM) in PBS buffer (pH 7.4, 10 mM) in the presence of various species. λex = 400 nm. Slits: 5 nm/5 nm. (Inset) Corresponding fluorescent color changes under a handle UV lamp (365 nm): 1: probe 1 alone; 2–20: probe 1 + HSO3−, HS−, F−, Cl−, Br−, I−, AcO−, ClO4−, NO3−, N3−, SO42−, HSO4−, SCN−, PO43−, HPO42−, H2PO4−, Cys, Hcy and GSH (anions: 60 μM; Cys and Hcy: 200 μM; GSH: 10 mM). | |
3.2 Sensing mechanism
A well-known sensing process takes place between 1 and HSO3− with a Michael addition reaction. 1H NMR analysis was carried out to demonstrate the sensing mechanism. As anticipated, after addition of HSO3− into the solution of 1, the nucleophilic attack of HSO3− toward C-4 disturbed the π–π conjugation and caused all the proton signals shifted to up-field (Fig. 3). It was noticed that the proton of the methyl group (Ha) connected with N+ was dramatically shifted from δ 4.34 to 3.09 ppm. Another irrefutable evidence was the equivalent proton of the two methyl groups (Hb) at δ 2.03 ppm, then also shifted to up-field and splitted into two signals (Hb′, at δ 1.89 and 1.56 ppm). This adduct was further confirmed and characterized by HRMS analysis (Fig. S9†), where the peak at m/z 468.1398 (calc. = 468.1387) corresponding to [M − SO3]− was clearly observed.
 |
| Fig. 3 1H NMR spectral change of 1 (3 × 10−3 M) in the absence (A) and presence of 1 equiv. (B), of NaHSO3 in DMSO-d6 : D2O = 9 : 1 (v/v). | |
3.3 Real samples analysis
To investigate the applicability of the probe to real samples, the levels of HSO3− in granulated sugar, soft sugar and crystal sugar were examined by using the proposed method.13 The recoveries were good enough for practical use and determination values are listed in Table 1. The HSO3− levels in these samples were 16.96, 10.16, and 14.46 mg kg−1, respectively.
Table 1 Results for the determination of HSO3− in various samples
Samples |
HSO3− level/(μmol L−1) |
Added/(μmol L−1) |
Found/(μmol L−1) |
Recovery/% |
Granulated sugar |
4.07 |
4 |
7.93 |
98.26 |
6 |
10.02 |
99.50 |
Soft sugar |
2.44 |
4 |
6.61 |
102.64 |
6 |
8.76 |
103.79 |
Crystal sugar |
3.47 |
4 |
7.34 |
98.26 |
6 |
9.31 |
98.31 |
3.4 Test papers detection
A preliminary paper test strip system was constructed as shown in Fig. S10.† After dropping various anions to the prepared test papers, only HSO3− made the color changed from orange to blue under the UV light (365 nm). Moreover, the test papers could also detect the HSO3− in real sugar samples as shown in Fig. 4 and S11.† It can be seen that glucose without HSO3− did not cause any color change; while the sugar solution made the color changing at different concentrations.
 |
| Fig. 4 The photos of the filter paper containing 1 treated with real sugar samples under UV light (365 nm, bottom): probe 1, HSO3−, glucose, soft sugar of 1 g mL−1, soft sugar of 0.7 g mL−1, soft sugar of 0.4 g mL−1, and soft sugar of 0.1 g mL−1. | |
3.5 Cellular imaging
The potential application of probe 1 for living cell detection of HSO3− was investigated. Confocal fluorescence imaging in living HepG2 cells was carried out under selective excitation at 405 nm. First, the MTT assay for probe 1 showed that the probe with a concentration range between 5–15 μM has only minimal cytotoxicity after 12 h (Fig. S12†), thus the probe at 10 μM was selected for imaging experiments. The cells incubated with probe 1 (10 μM) for 15 min displayed strong fluorescence in the red channel and weak fluorescence in the green channel, confirming that probe 1 is cell-permeable (Fig. 5). Since a certain concentration of HSO3− (15 μM) has been indicated in cells, the observed strong fluorescence in the green channel is probably a result of the fact that probe 1 binds HSO3− in cells. These cell experiments indicated that probe 1 could competent for imaging HSO3− in living cells.
 |
| Fig. 5 Confocal fluorescence images in Hala cells. (A) Bright field imaging of the cells with adding probe 1 (10 μM) for 15 min; (B) fluorescent imaging of (A) from the green channel; (C) fluorescent imaging of (A) from the red channel; (D) overlap of (A) and (C). (E) Bright field imaging of the cells were treated with probe 1 (10 μM) for 15 min and subsequently treated with HSO3− (15 μM) for another 15 min; (F) fluorescent imaging of (E) from the green channel; (G) fluorescent imaging of (E) from the red channel; (H) overlap of (E) and (F). λex = 405 nm. | |
4. Conclusion
In summary, we have developed a new ratiometric fluorescent probe bearing a benzo[e]indolium group for HSO3− detection in PBS buffer under physiology conditions. The probe displayed a dramatic fluorescent change and a color change after exposure to HSO3− with a very short response time (within 30 s). Probe 1 allowed the detection of HSO3− with high sensitivity at a very low concentration. These advantages made the probe a potential sensor for HSO3− detection in real sugar samples and in living cells.
Acknowledgements
This work was supported by the National Natural Science Foundation of china (No. 21301126).
References
- T. Fazio and C. R. Warner, Food Addit. Contam., 1990, 7, 433–454 CrossRef CAS PubMed.
- S. L. Taylor, N. A. Higley and R. K. Bush, Adv. Food Res., 1986, 30, 1–76 CAS.
- H. Vally, N. L. Misso and V. Madan, Clin. Exp. Allergy, 2009, 39, 1643–1651 CrossRef CAS PubMed.
- White granulated sugar GB317-2006, General administration of quality supervision, Inspection and Quarantine of the People's Republic of China, 2006.
- J. Chan, S. Dodani and C. Chang, Nat. Chem., 2012, 4, 973–984 CrossRef CAS PubMed.
- M. Schäferling, Angew. Chem., Int. Ed., 2012, 15, 3532–3554 CrossRef PubMed.
- Y. Sun, C. Zhong, R. Gong, H. Mu and E. Fu, J. Org. Chem., 2009, 74, 7943–7946 CrossRef CAS PubMed.
- C. Wang, S. Feng, L. Wu, S. Yan, C. Zhong, P. Guo, R. Huang, X. Weng and X. Zhou, Sens. Actuators, B, 2014, 190, 792–799 CrossRef CAS.
- M. G. Choi, J. Hwang, S. Eor and S.-K. Chang, Org. Lett., 2010, 12, 5624–5627 CrossRef CAS PubMed.
- X. Gu, C. Liu, Y.-C. Zhu and Y.-Z. Zhu, J. Agric. Food Chem., 2011, 59, 11935–11939 CrossRef CAS PubMed.
- S. Chen, P. Hou, J. Wang and X. Song, RSC Adv., 2012, 2, 10869–10873 RSC.
- H. Zhang, S. Xue and G. Feng, Sens. Actuators, B, 2016, 231, 752–758 CrossRef CAS.
- K. Chen, Y. Guo, Z. Lu, B. Yang and Z. Shi, Chin. J. Chem., 2010, 28, 55–60 CrossRef CAS.
- X.-F. Yang, M. Zhao and G. Wang, Sens. Actuators, B, 2011, 152, 8–13 CrossRef CAS.
- Y. Yang, F. Huo, J. Zhang, Z. Xie, J. Chao, C. Yin, H. Tong, D. Liu, S. Jin, F. Cheng and X. Yan, Sens. Actuators, B, 2012, 166–167, 665–670 CrossRef CAS.
- Y.-Q. Sun, P. Wang, J. Liu, J. Zhang and W. Guo, Analyst, 2012, 137, 3430–3433 RSC.
- G. Wang, H. Qi and X.-F. Yang, Luminescence, 2013, 28, 97–101 CrossRef CAS PubMed.
- X. Cheng, H. Jia, J. Feng, J. Qin and Z. Li, Sens. Actuators, B, 2013, 184, 274–280 CrossRef CAS.
- H. Xie, X. Jiang, F. Zeng, C. Yu and S. Wu, Sens. Actuators, B, 2014, 203, 504–510 CrossRef CAS.
- X. Su, X. Li, T. Ding, G. Zheng and Z. Liu, J. Organomet. Chem., 2015, 781, 59–64 CrossRef CAS.
- G. Li, Y. Chen, J. Wang, Q. Lin, J. Zhao, L. Ji and H. Chao, Chem. Sci., 2013, 4, 4426–4433 RSC.
- G. Li, Y. Chen, J. Wang, J. Wu, G. Gasser, L. Ji and H. Chao, Biomaterials, 2015, 63, 128–136 CrossRef CAS PubMed.
- Y.-Q. Sun, J. Liu, J. Zhang, T. Yang and W. Guo, Chem. Commun., 2013, 49, 2637–2639 RSC.
- H. Tian, J. Qian, Q. Sun, H. Bai and W. Zhang, Anal. Chim. Acta, 2013, 788, 165–170 CrossRef CAS PubMed.
- M.-Y. Wu, T. He, K. Li, M.-B. Wu, Z. Huang and X.-Q. Yu, Analyst, 2013, 138, 3018–3025 RSC.
- M.-Y. Wu, K. Li, C.-Y. Li, J.-T. Hou and X.-Q. Yu, Chem. Commun., 2014, 50, 183–185 RSC.
- L. Zhu, J. Xu, Z. Sun, B. Fu, C. Qin, L. Zeng and X. Hu, Chem. Commun., 2015, 51, 1154–1156 RSC.
- Q. Zhang, Y. Zhang, S. Ding, H. Zhang and G. Feng, Sens. Actuators, B, 2015, 211, 377–384 CrossRef CAS.
- X. Dai, T. Zhang, Z.-F. Du, X.-J. Cao, M.-Y. Chen, S.-W. Hu, J.-Y. Miao and B.-X. Zhao, Anal. Chim. Acta, 2015, 888, 138–145 CrossRef CAS PubMed.
- G. Xu, H. Wu, X. Liu, R. Feng and Z. Liu, Dyes Pigm., 2015, 120, 322–327 CrossRef CAS.
- W. Chen, Q. Fang, D. Yang, H. Zhang, X. Song and J. Foley, Anal. Chem., 2015, 87, 609–616 CrossRef CAS PubMed.
- W. Chen, X. Liu, S. Chen, X. Song and J. Kang, RSC Adv., 2015, 5, 25409–25415 RSC.
- Y. Chen, X. Wang, X.-F. Yang, Y. Zhong, Z. Li and H. Li, Sens. Actuators, B, 2015, 206, 268–275 CrossRef CAS.
- Y. Liu, K. Li, M.-Y. Wu, Y.-H. Liu, Y.-M. Xie and X.-Q. Yu, Chem. Commun., 2015, 51, 10236–10239 RSC.
- Y. Liu, K. Li, K.-X. Xie, L.-L. Li, K.-K. Yu, X. Wang and X.-Q. Yu, Chem. Commun., 2016, 52, 3430–3433 RSC.
- H. Zhang, Z. Huang and G. Feng, Anal. Chim. Acta, 2016, 920, 72–79 CrossRef CAS PubMed.
- Y. Zhang, L. Guan, H. Yu, Y. Yan, L. Du, Y. Liu, M. Sun, D. Huang and S. Wang, Anal. Chem., 2016, 88, 4426–4431 CrossRef CAS PubMed.
- D.-P. Li, Z.-Y. Wang, X.-J. Cao, J. Cui, X. Wang, H.-Z. Cui, J.-Y. Miao and B.-X. Zhao, Chem. Commun., 2016, 52, 2760–2763 RSC.
- J. Chao, Z. Li, Y. Zhang, F. Huo, C. Yin, Y. Liu, Y. Li and J. Wang, J. Mater. Chem., 2016, 4, 3703–3712 RSC.
- L. Y. Niu, Y. S. Guan, Y. Z. Chen, L. Z. Wu, C. H. Tung and Q. Z. Yang, J. Am. Chem. Soc., 2012, 134, 18928–18931 CrossRef CAS PubMed.
- M. Shimizu, R. Kaki, Y. Takeda, T. Hiyama, N. Nagai, H. Yamagishi and H. Furutani, Angew. Chem., Int. Ed., 2012, 51, 4095–4099 CrossRef CAS PubMed.
Footnote |
† Electronic supplementary information (ESI) available. See DOI: 10.1039/c6ra15605c |
|
This journal is © The Royal Society of Chemistry 2016 |