DOI:
10.1039/C6RA11510A
(Paper)
RSC Adv., 2016,
6, 61777-61783
Acetyl shikonin induces IL-12, nitric oxide and ROS to kill intracellular parasite Leishmania donovani in infected hosts†
Received
4th May 2016
, Accepted 14th June 2016
First published on 15th June 2016
Abstract
Acetyl shikonin (AS), a naphthoquinone isolated from Arnebia nobilis, was tested against visceral leishmaniasis (VL) in this study. AS induced anti-VL activity was examined for a number of intracellular amastigotes in Giemsa-stained BALB/C derived macrophages in vitro and hepatic-splenic parasite burden by the stamp smear method in vivo. Mediators of AS induced anti-VL were studied by analysis of nitrites and reactive oxygen species (ROS) and were further confirmed using their inhibitors. The effect of AS on interleukin (IL)-12 and IL-10 was analyzed by ELISA and RT-PCR for infected macrophages. AS treatment caused a nitrite and ROS mediated decrease in the parasite load in murine infected macrophages. These events are associated with the up-regulation of IL-12 and down-regulation of IL-10. In addition, AS was efficient for decreasing the hepatic and splenic parasite load and modulated the IL-12 and IL-10 ratio in splenocytes. In summary, this study revealed that AS provides a chemo-immunotherapeutic strategy against visceral leishmaniasis.
1. Introduction
Visceral leishmaniasis is caused mainly by the protozoan intracellular parasite Leishmania donovani and is transmitted from one mammalian host to another via the infected female sandfly. There are 0.3 million new cases per year worldwide. Among them, more than 90% are reported in 6 countries i.e., Bangladesh, Brazil, Ethiopia, India, South Sudan and Sudan.1 The parasite resides and multiplies inside the host macrophages by evading the host’s immune response and also drug access.2 The parasite mediated immune suppression increases the risk of collateral deadly infections such as HIV.3 Thus, the host’s protective immune response should be induced to treat this disease and compounds having immunomodulatory activities could be a promising therapeutic choice.4,5
Our group recently reported that acetyl shikonin (AS), a natural derivative of shikonin – a naphthoquinone, which is a major component in the dried root bark of Arnebia nobilis among others.6,7 Shikonin has been used as a traditional medicine for many years to treat burn-wounds8 and inflammatory diseases.9 This has been extended to use as an antioxidant,10 antimicrobial,11–13 anti-fungal and 14 anti-lung cancer15,16 agent and we recently reported its use against human chronic myeloid leukemia.17 Besides that, naphthoquinones are well established antiprotozoals, specifically anti-malarial.18,19 Acetyl shikonin acts as an inhibitor of DNA topoisomerases,20 cysteine proteases,21 cyclooxygenase (COX)-2, and extracellular signal-regulated kinase 1/2 (ERK1/2),22 which are attractive targets for treating visceral leishmaniasis.23–26 Acetyl shikonin was also reported to have in vitro leishmanicidal activity against promastigotes and amastigotes of the L. major strain27 but the immunomodulatory effect in the context of host macrophage activation to kill the L. donovani parasite has not been studied elsewhere.
Nitric oxide generation is harmful for the survival of the leishmanial parasites inside the phagolysosome.28 Leishmanial parasites dampen the host’s immune response through up-regulation of IL-10 to suppress the inducible nitric oxide synthase 2 (iNOS2) activity followed by inhibition of nitrite generation and generate a haven for the parasite inside the macrophages.29 Previous reports indicate that orally administered acetyl shikonin induces nitric oxide levels in an ethanol-induced ulcer model in rats.30 Besides that, the opposite effect for a different concentration has been reported in microglial cells.21 Since nitric oxide generation is concentration dependent, we tried to find the concentration of acetyl shikonin that induces nitric oxide mediated killing of the parasite in L. donovani infected macrophages in vitro. Proinflammatory cytokines (IL-12), required for parasite clearance, and anti-inflammatory cytokines (IL-10), required for parasite survival, were also determined at the protein and mRNA levels. Finally, we have checked the in vivo liver and splenic parasite clearance ability of acetyl shikonin. Altogether, in this present study, we are reporting the mechanistic aspect of acetyl shikonin induced anti-visceral leishmanial activity.
2. Experimental
2.1 Chemicals and reagents
RPMI 1640 medium was obtained from Himedia, India. Penicillin, streptomycin and fetal bovine serum (FBS) were obtained from Gibco BRL (Grand Island, NY, USA). The Quantikine M ELISA Kit for interleukin (IL)-12 and IL-10 was obtained from R & D systems. Primers for semi-quantitative PCR were purchased from Sigma, India. All other chemicals were purchased from either Sigma (USA) or Merck.
2.2 Animals, cells and parasites
This study was carried out in strict accordance with the recommendations in the Guide for the Care and Use of Laboratory Animals of the National Institutes of Health. All experimental animal protocols received prior approval from the Institutional Animal Ethical Committee (SASTRA University, Registration Number: 817/PO/ReRc/S/04/CPCSEA; dated: 20.11.2015). BALB/c mice (male, 4–6 weeks, 28–30 g) were procured from the Central Animal Facility, SASTRA University, Thanjavur. Peritoneal macrophages were isolated by 4% thioglycolate intraperitoneal injections followed by harvesting after 5 days. Peritoneal macrophages were cultured in RPM I1640 with 10% FBS and antibiotics for 48 h prior to all experiments. The L. donovani strain AG-83 (MHOM/IN/1983/AG-83) was maintained in vitro in RPMI-1640 (Himedia) containing 10% fetal bovine serum. The virulence of the parasite was maintained by regular passage through BALB/c mice.31
2.3 Isolation of acetyl shikonin
Acetyl shikonin was isolated from the dried root bark of Arnebia nobilis by the adsorption based elution method as described previously,6 and characterized by 1H-NMR, 13C-NMR and FT-IR (ESI; Fig. S1–S3†).6 Acetyl shikonin was dissolved in cell culture grade DMSO as a 10 mg mL−1 stock solution and further diluted in RPMI 1640 medium or PBS to obtain the required concentrations for the treatment in vitro and in vivo.
2.4 Cytotoxicity determination by MTT assay
Mouse derived peritoneal macrophages (1 × 105) were seeded in 96-well cell culture plates (Genetix), allowed to adhere and cultured for 48 h. Adherent macrophages were treated with acetyl shikonin (0–500 μg mL−1) for 48 h. Then an MTT assay was performed as described previously.32
2.5 Parasite burden in peritoneal macrophages
The parasitic burden was determined in 8-well cell culture slides (Genetix). Peritoneal macrophages (2 × 105) were infected with promastigotes (macrophage
:
promastigotes = 1
:
10) for 6 h followed by washing with fresh complete culture media to remove the non-ingested promastigotes. The cultures were incubated for the indicated time period (24 h to 72 h) with and without acetyl shikonin (0 to 25 μg mL−1). After that, the macrophages were fixed with methanol, stained with Giemsa and counted under a light microscope (E600, Nikon). The parasite burden was expressed as the number of amastigotes per hundred macrophages.33
2.6 Nitric oxide (NO) and reactive oxygen species (ROS) generation
Nitric oxide generation was estimated by measuring nitrite accumulation in the culture supernatant calorimetrically using Griess reagent (Sigma) and was expressed in micromoles of nitrite.32 ROS generation was estimated using H2DCFDA (Sigma) as described elsewhere.32,34 Peritoneal macrophages from different treatment sets were incubated with H2DCFDA (2 μg mL−1) at room temperature for 20 min in the dark. The relative fluorescence was measured in a Biotek Spectrofluorometer (Synergy H1) at λEx 485 nm and λEm 525 nm. Fluorometric measurements were expressed in mean fluorescence intensity units.
2.7 Estimation of cytokine release by sandwich ELISA
The concentration of interleukin (IL)-10 and IL-12 were analyzed in the cell culture supernatant using a sandwich ELISA kit (R & D systems) as per the manufacturer’s instructions.
2.8 Isolation of RNA and reverse transcription polymerase chain reaction
The total RNA was extracted from treated and untreated uninfected or infected macrophages (3 × 106) with the use of TRIZOL Reagent (Invitrogen), according to the manufacturer’s protocol. The isolated RNA (1 μg) was then reverse transcribed using Revert Aid M-MuLV Reverse Transcriptase (Thermo). The resulting complementary DNA was used for RT-PCR (Applied Biosystems) to detect iNOS2, IL-10 and IL-12. Glyceraldehyde 3-phosphate dehydrogenase (GAPDH) was used as a loading control. The forward and reverse specific primer sequences used were as follows: iNOS2 (forward 5′-CAG AGG ACC CAG AGA CAA GC-3′, reverse 5′-AAG ACC AGA GGC AGC ACA TC-3′); IL-10 (forward 5′-TCA CTC TTC ACC TGC TCC AC-3′, reverse 5′- CTA TGC TGC CTG CTC TTA CTC-3′); IL-12 (forward 5′-CAC GCC TGA AGA AGA TGA CA-3′, reverse 5′-GAC AGA GAC GCC ATT CCA CA-3′); GAPDH (forward 5′-GAG CCA AAC GGG TCA TCA TC-3′, reverse 5′-CCT GCT TCA CCA CCT TCT TG-3′).
2.9 In vivo anti-visceral leishmanial activity of acetyl shikonin
BALB/c mice (28–30 g) were divided into following groups: (a) uninfected control, (b) infected with L. donovani promastigotes (2 × 107) through i.v. injection and (c) acetyl shikonin (10 mg kg−1 BW) i.v. treated L. donovani infected groups. The infected and acetyl shikonin treated infected group mice were infected for 14 days to establish the infection. After that acetyl shikonin was administered intravenously through the tail vein for another 14 days. After a total of 28 days, all the mice were sacrificed by CO2 inhalation and the liver and spleen parasite loads in the BALB/c mice were assessed by the stamp-smear method under light microscope. The parasite load was expressed in Leishman–Donovan units as described elsewhere.34,35 Isolated splenocytes were cultured in RPMI 1640 medium plus 10% (FBS) for IL-10 and IL-12 cytokine profiling.34,35
2.10 Statistical analysis
The data are presented here as mean ± SD. All of the in vitro experiments were done in duplicate and repeated at least three times. In vivo experiments were done with 4 mice per group. Comparisons of the means of the control, infected group and infected treated group were done by ANOVA (using statistical software, Origin 6.1) to determine the significance level.
3. Results
3.1 Determination of non-cytotoxic dose of acetyl shikonin towards normal mouse macrophages
The test compound, acetyl shikonin, should not show toxicity to the host cells. Therefore, to determine the non-cytotoxic dose of acetyl shikonin against the host cells, we treated the peritoneal macrophages with acetyl shikonin from 0.01–500 μg mL−1 for 48 h. In the MTT assay, 88.42% viable macrophages were observed at 25 μg mL−1, whereas 82.2% viable cells were found at 50 μg mL−1 which was significantly (p < 0.05) different to the untreated control macrophages. Hence, we selected the highest dose as 25 μg mL−1 of acetyl shikonin to treat the infected macrophages.
3.2 Determination of effective dose of acetyl shikonin required for intracellular parasite clearance in L. donovani infected macrophages
The number of intracellular amastigotes indicates the extent of leishmanial infection.31 To determine the acetyl shikonin induced intracellular parasite killing, we performed concentration (0.5 to 25 μg mL−1) dependent and time dependent studies. Our results indicate that acetyl shikonin mediated decreased parasitemia were first significantly observed at 5 μg mL−1 concentration after 24 h, and 2.5 μg mL−1 after 48 h and 72 h of treatment. Maximum parasite elimination was observed at the highest studied concentration at all three time points. 32.4%, 58.3%, 75.01% and 84.81% inhibition of intracellular parasites was found after 48 h of treatment with 2.5 μg mL−1, 5 μg mL−1, 10 μg mL−1 and 25 μg mL−1 concentration of acetyl shikonin, respectively, whereas after 72 h treatment the parasite load inhibition was found to be 40.01%, 75.01%, 87.82% and 91.66%, respectively. There were no further significant differences observed for the 10 μg mL−1 and 25 μg mL−1 treated groups at both 48 h and 72 h. Hence, we selected the 10 μg mL−1 concentration of acetyl shikonin for the further study.
3.3 Acetyl shikonin enhanced nitric oxide (NO) and ROS generation in L. donovani infected macrophages
The generation of nitric oxide is unfavourable for parasite survival inside macrophages and its synthesis depends upon the enzyme iNOS2.36 Therefore, we determined the acetyl shikonin induced NO generation in L. donovani infected and uninfected macrophages. Initially we checked the concentration dependent NO generation with acetyl shikonin administration in uninfected and infected macrophages. This study clearly confirmed a concentration dependent nitrite generation, indicating that acetyl shikonin was proficient enough in NO generation (ESI Fig. S4†). Our results showed that acetyl shikonin (10 μg mL−1) was able to significantly (p < 0.01) elicit 2.84 fold and 3.87 fold increases of nitrite liberation in uninfected and infected macrophages, respectively, after 48 h (Fig. 3A). Since iNOS2 is responsible for NO generation, we checked the mRNA expression of iNOS2 by semi-quantitative PCR, confirming its role in acetyl shikonin induced nitrite generation (Fig. 3B and C). Besides that, ROS, specifically superoxide and hydrogen peroxide, caused the death of the parasite.37 Thus, we determined the acetyl shikonin-induced ROS generation in uninfected and L. donovani infected macrophages after 1 h. Our results indicate a gradual increase in ROS generation in treated macrophages in a concentration dependent manner (ESI Fig. S5†). Significant (p < 0.05) ROS generation was also observed at 10 μg mL−1 of acetyl shikonin administration in uninfected and infected macrophages (Fig. 3D).
To check the specific role of acetyl shikonin induced nitrite and ROS generation to reduce the L. donovani parasite load in macrophages, we administered L-NNMA (inhibitor of nitrite generation) and NAC (inhibitor of ROS generation) alone or together with acetyl shikonin and checked the acetyl shikonin induced parasite clearance after 48 h. We observed that acetyl shikonin induced parasite clearance was partially and significantly (p < 0.05) paralyzed when we administered L-NNMA and NAC individually in the presence of acetyl shikonin. Interestingly, acetyl shikonin induced parasite clearance was totally dampened, when these two inhibitors were administered together (Fig. 3E). Therefore, this experiment clearly indicates that acetyl shikonin induced parasite clearance in infected macrophages by both nitrite and ROS generation.
3.4 In vitro IL-12 and IL-10 release and expression in acetyl shikonin treated L. donovani infected macrophages
IL-12 is a strong proinflammatory cytokine, responsible for the clearance of L. donovani infection in macrophages,38,39 but will be suppressed by the parasite in the process of infection.40 In contrast, IL-10 is a strong anti-inflammatory cytokine that will be induced by the parasite in order to evade the host immune response and to promote the parasite survival,41,42 and the disease progression or parasite clearance is dependent on the IL-12 to IL-10 ratio.34,41 Hence, we have estimated the IL-12 and IL-10 levels in the cell-free supernatant of the culture media of acetyl shikonin treated uninfected and infected macrophages. Treatment with a 10 μg mL−1 concentration of acetyl shikonin resulted in a significant (p < 0.01) increase of IL-12 release as well as significant (p < 0.01) suppression of IL-10 release in L. donovani infected macrophages (Fig. 4A). In addition to the cytokine release, the mRNA expression levels, determined by semi-quantitative PCR, were modulated significantly (p < 0.01) (Fig. 4B) in acetyl shikonin treated L. donovani-infected macrophages.
3.5 Acetyl shikonin mediated decrease in the parasite load and promoted Th1 cytokine in L. donovani infected BALB/c mice
As we have found that acetyl shikonin treatment of L. donovani infected macrophages induced the host’s protective responses, we further studied the capability of acetyl shikonin in curing visceral leishmaniasis in the BALB/c mouse model. After establishing L. donovani infection, the infected mice were administered with acetyl shikonin (10 mg kg−1 body weight) intravenously through the tail vein for 14 days of infection. Our results indicate that acetyl shikonin efficiently decreased the liver and spleen parasite burden by 68.75% and 72.6% respectively, which were significant (p < 0.01) differences compared with the untreated infected group (Fig. 5A). Because the spleen and liver are the major sites of parasite infection in the in vivo system, the presence of parasites in their respective histology section with hematoxylin and eosin (H&E) were determined. The analysis of the liver and spleen tissue histology indicated the presence of haloed small granules (protozoan bodies) in the cytoplasm of kupffer cells and splenocytes in the liver and spleen, respectively, in the infected BALB/c mice, which were reduced in acetyl shikonin treated BALB/c mice (Fig. 5D and E).
IL-12, an important Th1 cytokine is involved in the clearance of Leishmania parasites in mice,39 while IL-10, an important Th2 and immunosuppressive cytokine, promoted the survival of the L. donovani parasite in the host.41 After completion of the treatment schedule, we studied the Th1 and Th2 cytokine production in spleen cells from acetyl shikonin treated and infected mice by ELISA. The results showed that there was significant inhibition of IL-12 and high amounts of IL-10 in the splenocytes of infected mice, compared with the uninfected mice. As expected, acetyl shikonin not only reduced the parasite load but also significantly (p < 0.01) induced IL-12 (Fig. 5B), and significantly (p < 0.05) reduced IL-10 (Fig. 5C) release compared with the infected BALB/c mice.
4. Discussion
Visceral leishmaniasis, caused by L. donovani, is fatal if left untreated for a long time. Existing drugs such as miltefosine, amphotericin B and paromomycin were found to be toxic and there have been cases where drug resistance was reported against these drugs.43 Generally, the parasite enters in a motile promastigote form into the mammalian system through the bite of a female sandfly, which feeds on blood. When the promastigotes make contact with the tissue macrophages, they achieve silent entry into its phagosome, bypassing phagolysosome formation, and converting the phagosome into a parasitophorous vacuole,29 where the parasite is converted into the non-motile amastigotes by losing flagella and then multiplies. This L. donovani parasite evades the host’s immune response by inducing a strong anti-inflammatory response (i.e., up-regulation of IL-10 and TGF-β) and also by suppressing the pro-inflammatory response (i.e., down-regulation of IL-12 and IFN-γ).44 The generation of free radicals such as ROS and reactive nitrogen intermediates induces an oxidative burst response and causes the death of the parasite. In order to promote parasite survival inside the macrophages, ROS and NO generation should be blocked by suppressing the expression of NADPH oxidase and iNOS2 respectively.44 The parasite multiplies continuously as amastigotes; the number of amastigotes inside the macrophages corresponds to the extent of infection and the spleen and liver are the major sites of parasite residence in vivo.
In this study, we investigated the potential of using acetyl shikonin against visceral leishmaniasis, the most fatal form of leishmaniasis, as it causes the highest rate of mortality. Initially, we tested the cytotoxicity of acetyl shikonin towards peritoneal macrophages, selected for the in vitro model for the anti-VL study, to determine the non-cytotoxic dose. We have found that up to 25 μg mL−1, inducing a negligible 11.58% cell cytotoxicity in vitro, can be used to treat the peritoneal macrophages (Fig. 1). Interestingly, 87.82% and 91.66% maximum parasite clearances in infected peritoneal macrophages were observed with 10 μg mL−1 and 25 μg mL−1 of the acetyl shikonin treatment, respectively, after 72 h, while there were no significant differences in parasite clearance observed when the effects of these two concentrations are compared (Fig. 2). Hence, we selected the 10 μg mL−1 concentration of acetyl shikonin for further in vitro studies of ROS generation, NO generation and cytokine expression. To provide evidence of the possible effector mechanisms involved in acetyl shikonin induced L. donovani clearance in infected peritoneal macrophages, we studied the levels of ROS and NO (two of the most important effector molecules in macrophages leading to parasite clearance) generation in uninfected and infected macrophages (Fig. 3). We found that the acetyl shikonin mediated anti-VL response was solely mediated by the induction of iNOS2 followed by the production of NO and also ROS generation. We further confirmed the specific role of NO and ROS generation in acetyl shikonin mediated antileishmania by using their specific inhibitors, L-NNMA and NAC (Fig. 3E). Pretreatment with the individual inhibitors partially blocked the acetyl shikonin induced parasite killing and combined treatment of L-NNMA and NAC totally dampened the parasite killing ability of the macrophages.
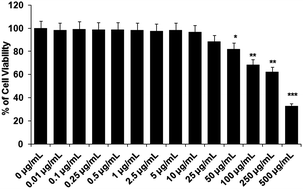 |
| Fig. 1 Determination of the noncytotoxic dose of acetyl shikonin (AS) towards macrophages. Cells were cultured in 96-well plates in complete 1640 media for 24 h. After that cells were treated with acetyl shikonin at specific doses (0.01–500 μg mL−1). After another 48 h of incubation, a cell viability assay was performed using the MTT method (spectrophotometric reading of the MTT-formazan formed was read at 570 nm and the data are expressed as the percentage of viable cells). The experiment was repeated 3 times, yielding similar results, and data were expressed as mean values ± standard deviation (SD); *p < 0.05, **p < 0.01, and ***p < 0.001 compared with the respective control. | |
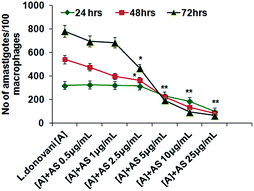 |
| Fig. 2 Determination of the effective concentration of AS required for intracellular parasite clearance. Macrophages plated in the chamber slide were infected with the L. donovani parasite for 6 h, then excess parasite was washed out and treated with acetyl shikonin (AS) at different concentrations. After 24, 48 and 72 h of treatment, cells were stained with Giemsa and the number of amastigotes/100 macrophages was counted. The experiments were repeated three times, yielding similar results, and data are expressed as mean ± SD; *p < 0.05 and **p < 0.01 compared with the respective infected control at defined time points. | |
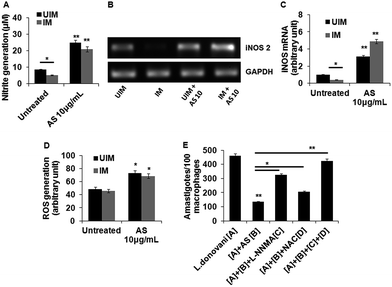 |
| Fig. 3 Acetyl shikonin enhanced nitric oxide (NO) generation, iNOS2 mRNA expression and ROS generation in L. donovani infected macrophages. (A) Macrophages were cultured and infected with L. donovani promastigotes. Infected and uninfected cells were treated with the indicated doses. After 48 h incubation nitrite generation was evaluated from the cell-free supernatants. (B and C) Uninfected and infected macrophages were treated with AS for 6 h, after which the cells were collected in TRIZOL. The RNA was extracted, followed by conventional RT-PCR using iNOS2-specific primers. The density units shown in the bar diagram are arbitrary units from densitometric analysis using Quantity-One software, Bio-Rad. (D) Acetyl shikonin enhanced reactive oxygen species (ROS) generation in L. donovani infected macrophages. Macrophages were cultured and infected with L. donovani promastigotes. Infected and uninfected cells were treated with AS at different concentrations for 1 h, then washed with PBS and treated with H2DCFDA (2 μg mL−1) for 30 min, after which the fluorescence was measured using a fluorimeter at λEx −485 and λEm −525 nm. (E) The effect of pretreatment with L-N-monomethyl arginine (L-NNMA; 0.4 mM) and N-acetylcysteine (NAC, 5 mM) on acetyl shikonin-induced parasite killing in peritoneal macrophages. All experiments were repeated 3 times, yielding similar results, and the data are expressed as mean values ± SD; *p < 0.05 and **p < 0.01 compared with the respective control. | |
The proinflammatory and anti-inflammatory cytokine levels not only determined the L. donovani parasite survival inside the host, but also regulated the induction of their effector molecules through complex regulation of different signaling pathways. In the case of L. donovani-induced visceral leishmaniasis, IL-10 and IL-12 have the most important roles in parasite survival and clearance, respectively.38,39,41 Acetyl shikonin modulated the IL-12 and IL-10 levels at the protein and mRNA levels in uninfected and infected macrophages (Fig. 4), providing direct evidence of acetyl shikonin induced parasite clearance inside the macrophages.
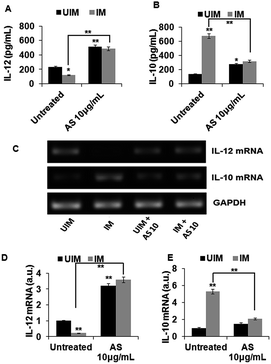 |
| Fig. 4 Differential mRNA expression and production of IL-10 and IL-12 in acetyl shikonin treated L. donovani infected macrophages. (A and B) Macrophages were cultured and infected with L. donovani promastigotes. Infected and uninfected cells were treated with AS at the indicated dose for 24 h and then the cell-free supernatants were collected and ELISA was performed to estimate the cytokine release. (C–E) Uninfected and infected macrophages were treated with AS at the indicated dose for 6 h, after which the cells were collected in TRIZOL. The RNA was extracted, followed by conventional RT-PCR using IL-10 and IL-12 specific primers. The density units shown in the bar diagram are arbitrary units obtained from the densitometry analysis using the Quantity-One software, Bio-Rad. The data represent the mean ± SD of data from three independent experiments; *p < 0.05 and **p < 0.01 compared with the respective control. | |
Quinones are highly reactive entities and are vital links in biochemical-electron transport, thus promoting pro-inflammatory and pro-oxidant behaviour. Acetyl shikonin is a naphthoquinone and naphthoquinones exert their action as either pro-oxidants, reducing oxygen to reactive oxygen species and also inducing nitrite generation, or as electrophiles (radical anion/dianion), forming covalent bonds with biological nucleophiles.45,46 In addition, shikonins are known to inhibit ERK1/2 which might lead to the up-regulation of IL-12 release, and down-regulation of IL-10, indicating the activation of iNOS2 to produce nitric oxide in the L. donovani infected peritoneal macrophages.22,47,48 Thus, the presence of this chemical structural feature (naphthoquinone) in acetyl shikonin can be plausibly linked to the leishmanicidal effect of acetyl shikonin on L. donovani.
In vivo experiments were conducted to study the potential of acetyl shikonin in defending a host suffering from visceral leishmaniasis. A significant (p < 0.01) decrease in the hepatic and splenic parasite burden was proof of disease progression control by acetyl shikonin (10 mg kg−1 BW) (Fig. 5A). The observation of much fewer protozoan bodies in the cytoplasm of Kupffer cells and splenic macrophages in H&E stained liver and spleen tissue sections also supported the decreased hepatic and splenic parasite burden (Fig. 5D and E). Besides this, the induction of Th1 (IL-12) and suppression of Th2 (IL-10) in cytokines in splenocytes indicate the immunotherapeutic potential of acetyl shikonin in restricting visceral leishmaniasis (Fig. 5B and C). Importantly, toxicity related changes in the tissue architecture were not found in acetyl shikonin treated L. donovani-infected BALB/c mice.
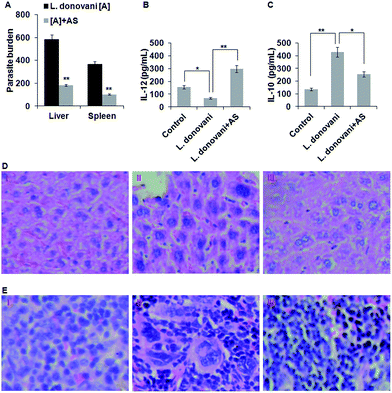 |
| Fig. 5 (A) Liver and spleen parasite load in LDU assessed by the stamp-smear method by light microscopy in BALB/c mice. Bar diagram indicates the mean ± SD of LDU data in the liver and spleen with 4 mice per group. (B and C) Splenocytes from each group were isolated and stimulated with SLA (5 μg mL−1) for 48 h. The cell-free supernatant was used to estimate the Th1 (IL-12) and Th2 (IL-10) cytokines by ELISA. The data represent the mean ± SD of the different groups (4 mice/group). (D and E) Histology photographs of liver (D) and spleen (E) of control (I), infected (II) and acetyl shikonin treated infected (III) BALB/c mice, observed under 400× magnification by light microscopy, for the best representative of the four mice per group; *p < 0.05 and **p < 0.01 compared with the respective control. | |
5. Conclusions
Altogether, our findings provide evidence for the mechanism of acetyl shikonin, isolated from the root bark of A. nobilis, against visceral leishmaniasis. Acetyl shikonin (10 μg mL−1) treatment efficiently induced parasite clearance in L. donovani infected peritoneal macrophages in vitro through the up-regulation of IL-12, iNOS2, and nitric oxide and ROS generation and the down-regulation of the anti-inflammatory cytokine, IL-10. This phytochemical (10 mg kg−1 BW) also effectively decreased the parasite burden and modulated the Th1 and Th2 cytokines in the BALB/c mouse model of visceral leishmaniasis. Hence, acetyl shikonin can be taken as an immunotherapeutic phytochemical to fight against visceral leishmaniasis.
Acknowledgements
The authors would like to thank the Management, SASTRA UNIVERSITY, for providing the necessary facilities and the TRR funding to Dr S. Kar Mahapatra. The financial support of SERB, Government of India (SB/YS/LS-285/2013) is sincerely acknowledged. Authors are thankful to Dr Davidraj C. and Mrs Parimaladevi ST, Central Animal Facility, SASTRA University for their kind help during Animal study.
References
- Visceral leishmaniasis: Report of a WHO bi-regional consultation Addis Ababa, Ethiopia, 2015 Search PubMed.
- R. Hadighi, M. Mohebali, P. Boucher, H. Hajjaran, A. Khamesipour and M. Ouellette, PLoS Med., 2006, 3(5), e162 CrossRef PubMed.
- J. Alvar, P. Aparicio, A. Aseffa, M. Den Boer, C. Cañavate, J. P. Dedet, L. Gradoni, R. Ter Horst, R. López-Vélez and J. Moreno, Clin. Microbiol. Rev., 2008, 21(2), 334 CrossRef CAS PubMed.
- L. Kedzierski, A. Sakthianandeswaren, J. M. Curtis, P. C. Andrews, P. C. Junk and K. Kedzierska, Curr. Med. Chem., 2009, 16(5), 599 CrossRef CAS PubMed.
- A. M. Musa, S. Noazin, E. A. Khalil and F. Modabber, Trans. R. Soc. Trop. Med. Hyg., 2010, 104(1), 1 CrossRef CAS PubMed.
- S. Subramaniam, A. Palanisamy and A. Sivasubramanian, RSC Adv., 2015, 5, 6265 RSC.
- V. P. Papageorgiou, A. N. Assimopoulou, E. A. Couladouros, D. Hepworth and K. C. Nicolaou, Angew. Chem., Int. Ed. Engl., 1999, 38, 270 CrossRef.
- X. Chen, L. Yang, J. J. Oppenheim and O. M. Z. Howard, Phytother. Res., 2002, 16, 199 CrossRef CAS PubMed.
- L. Lu, A. Qin, H. Huang, P. Zhou, C. Zhang, N. Liu, S. Li, G. Wen, C. Zhang, W. Dong, X. Wang, Q. P. Dou and J. Liu, Eur. J. Pharmacol., 2011, 658, 242 CrossRef CAS PubMed.
- Z. Wang, T. Liu, L. Gan, T. Wang, X. Yuan, B. Zhang, H. Chen and Q. Zheng, Eur. J. Pharmacol., 2010, 643, 211 CrossRef CAS PubMed.
- C. C. Shen, W. J. Syu, S. Y. Li, C. H. Lin, G. H. Lee and C. M. Sun, J. Nat. Prod., 2002, 65, 1857 CrossRef CAS PubMed.
- X. Ding, B. Yin, L. Qian, Z. Zeng, Z. Yang, H. Li, Y. Lu and S. Zhou, J. Med. Microbiol., 2011, 60, 1827 CrossRef CAS PubMed.
- S. Subramaniam, N. Rajendran, S. B. Muralidharan, G. Subramaniam, R. Raju and A. Sivasubramanian, RSC Adv., 2015, 5, 77168 RSC.
- H. Miao, L. Zhao, C. Li, Q. Shang, H. Lu, Z. Fu, L. Wang, Y. Jiang and Y. Cao, Biol. Pharm. Bull., 2012, 35, 1956 Search PubMed.
- X. P. Guo, X. Y. Zhang and S. D. Zhang, Zhongguo Zhongxiyi Jiehe Jijiu Zazhi, 1991, 11(10), 598 Search PubMed.
- W. Lan, S. Wan, W. Gu, H. Wang and S. Zhou, Cell Biochem. Biophys., 2014, 70(2), 1459 CrossRef CAS PubMed.
- K. B. A. Ahmed, S. Kar Mahapatra, M. R. Charan Raja, S. Subramaniam, M. Sengan, N. Rajendran, S. K. Das, K. Haldar, S. Roy, S. Sivasubramanian and V. Anbazhagan, RSC Adv., 2016, 6, 18980 RSC.
- A. T. Hudson, A. W. Randall, M. Fry, C. D. Ginger, B. Hill, V. S. Latter, N. McHardy and R. B. Williams, Parasitology, 1985, 90, 45 CrossRef PubMed.
- S. L. Croft, A. T. Evans and R. A. Neal, Ann. Trop. Med. Parasitol., 1985, 79, 651 CrossRef CAS PubMed.
- Z. F. Plyta, T. Li, V. P. Papageorgiou, A. S. Mellidis, A. N. Assimopoulou, E. N. Pitsinos and E. A. Couladouros, Bioorg. Med. Chem. Lett., 1998, 8, 3385 CrossRef PubMed.
- R. Wang, R. Yin, W. Zhou, D. Xu and S. Li, Expert Opin. Ther. Pat., 2012, 22(9), 977 CrossRef CAS PubMed.
- K. Subbaramaiah, P. Bulic, Y. Lin, A. J. Dannenberg and D. S. Pasco, J. Biomol. Screening, 2001, 6(2), 101 Search PubMed.
- B. B. Das, A. Ganguly and H. K. Majumder, Adv. Exp. Med. Biol., 2008, 625, 103 CrossRef PubMed.
- E. Svensjö, P. R. Batista, C. I. Brodskyn, R. Silva, A. P. Lima, V. Schmitz, E. Saraiva, J. B. Pesquero, M. A. Mori, W. Müller-Esterl and J. Scharfstein, Microbes Infect., 2006, 8(1), 206–220 CrossRef PubMed.
- A. Awasthi, R. Mathur, A. Khan, B. N. Joshi, N. Jain, S. Sawant, R. Boppana, D. Mitra and B. Saha, J. Exp. Med., 2003, 197(8), 1037 CrossRef PubMed.
- S. Bhattacharjee, A. Bhattacharjee, S. Majumder, S. B. Majumdar and S. Majumdar, J. Antimicrob. Chemother., 2012, 67(8), 1905–1914 CrossRef CAS PubMed.
- A. Ali, A. N. Assimopoulou, V. P. Papageorgiou and H. Kolodziej, Planta Med., 2011, 77(18), 2003 CrossRef CAS PubMed.
- J. B. Hibbs Jr, R. R. Taintor, Z. Vavrin and E. M. Rachlin, Biochem. Biophys. Res. Commun., 1988, 157, 87 CrossRef CAS erratum: Biochem. Biophys. Res. Commun, 1989, 158, 624.
- G. Gupta, S. Oghumu and A. R. Satoskar, Adv. Appl. Microbiol., 2013, 82, 155 CAS.
- A. Toker, F. Akcay, H. Aksoy, H. Suleman, U. Özgen and H. Erdem, Turk. J. Med. Sci., 2013, 43, 315 CAS.
- P. Wadhone, M. Maiti, R. Agarwal, V. Kamat, S. Martin and B. Saha, J. Immunol., 2009, 182(11), 7146 CrossRef CAS PubMed.
- S. Kar Mahapatra, S. Bhattacharjee, S. P. Chakraborty, S. Majumdar and S. Roy, Int. Immunopharmacol., 2011, 11, 485 CrossRef PubMed.
- B. Saha, A. Saini, R. Germond, P. J. Perrin, D. M. Harlan and T. A. Davis, Eur. J. Immunol., 1999, 29(7), 2319 CrossRef CAS PubMed.
- G. Gupta, S. Bhattacharjee, S. Bhattacharyya, P. Bhattacharya, A. Adhikari, A. Mukherjee, S. B. Majumdar and S. Majumdar, J. Infect. Dis., 2009, 200(8), 1300 CrossRef CAS PubMed.
- B. Saha, H. N. Roy, A. Pakrashi, R. N. Chakraborty and S. Roy, Eur. J. Immunol., 1991, 21, 577 CrossRef CAS PubMed.
- F. Y. Liew, S. Millott, C. Parkinson, R. M. Palmer and S. Moncada, J. Immunol., 1990, 144, 4794 CAS.
- H. W. Murray, J. Immunol., 1982, 129, 351 CAS.
- A. R. Satoskar, S. Rodig, S. R. Telford 3rd, A. A. Satoskar, S. K. Ghosh, F. von Lichtenberg and J. R. David, Eur. J. Immunol., 2000, 30(3), 834 CrossRef CAS PubMed.
- A. Adhikari, G. Gupta, S. Majumder, S. Banerjee, S. Bhattacharjee, P. Bhattacharya, S. Kumari, S. Haldar, S. B. Majumdar, B. Saha and S. Majumdar, PLoS One, 2012, 7(7), e40265 CrossRef CAS PubMed.
- M. A. McDowell and D. L. Sacks, Curr. Opin. Microbiol., 1999, 2(4), 438 CrossRef CAS PubMed.
- D. Chandra and S. Naik, Clin. Exp. Immunol., 2008, 154(2), 224 CrossRef CAS PubMed.
- A. Meddeb-Garnaoui, H. Zrelli and K. Dellagi, Clin. Exp. Immunol., 2009, 155(2), 199 CrossRef CAS PubMed.
- S. Mohapatra, Trop. Parasitol., 2014, 4(1), 4 CrossRef PubMed.
- M. Olivier, D. J. Gregory and G. Forget, Clin. Microbiol. Rev., 2005, 18(2), 293 CrossRef CAS PubMed.
- O. Ramirez, L. B. Motta-Mena, A. Cordova and K. M. Garza, PLoS One, 2014, 9(9), e106828 CrossRef PubMed.
- Y. Kumagai, Y. Shinkai, T. Miura and A. K. Cho, Annu. Rev. Pharmacol. Toxicol., 2012, 52, 221 CrossRef CAS PubMed.
- P. Bhattacharya, G. Gupta, S. Majumder, A. Adhikari, S. Banerjee, K. Halder, S. B. Majumdar, M. Ghosh, S. Chaudhuri, S. Roy and S. Majumdar, PLoS One, 2011, 6, e24141 CrossRef CAS PubMed.
- A. R. Satoskar, S. Rodig, S. R. Telford, A. A. Satoskar, S. K. Ghosh, F. von Lichtenberg and J. R. David, Eur. J. Immunol., 2000, 30, 834 CrossRef CAS PubMed.
Footnote |
† Electronic supplementary information (ESI) available: Isolation of acetyl shikonin; 1H-NMR, 13C-NMR and FT-IR of acetyl shikonin; concentration dependent NO and ROS generation. See DOI: 10.1039/c6ra11510a |
|
This journal is © The Royal Society of Chemistry 2016 |