DOI:
10.1039/C6RA08749C
(Paper)
RSC Adv., 2016,
6, 58426-58433
Preparation of a phosphorous-free terpolymer as a decalcifying agent for removing calcium from crude oil
Received
5th April 2016
, Accepted 31st May 2016
First published on 2nd June 2016
Abstract
A novel phosphorous-free terpolymer, used as a decalcifying agent for removing calcium from crude oil, was prepared through a free-radical polymerization reaction of acrylic acid (AA), allylpolyethoxy amino carboxylate (APEA), and 2-hydroxyethyl acrylate (HEA) in water with ammonium persulfate as initiator. The structure of the synthesized AA–APEA–HEA terpolymer was characterized by Fourier transform infrared spectrometry and 1H nuclear magnetic resonance (1H-NMR). AA–APEA–HEA was used to remove calcium from Luning pipeline crude oil. The effects of several factors, such as molar ratio, dosage, and reaction temperature on calcium removal efficiencies from crude oil were evaluated. The results indicated that the removal of calcium from crude oil using AA–APEA–HEA was far more efficient than that using AA–APEA, PAA, HPMA, and PESA under the same conditions. The optimum conditions for calcium removal from crude oils were as follows: (a) molar ratio of AA–APEA–HEA, 2
:
1
:
0.5; (b) dosage of AA–APEA–HEA, 100 ppm; (c) reaction temperature, 100 °C. Under these conditions, the removal efficiency of calcium from Luning pipeline crude oils was approximately 98.63%. Furthermore, the proposed decalcification mechanism suggests surface complexation and chelation between the carboxylic (–COO−) and amino (–NH2) functional groups and Ca2+, with the polyethylene glycol segments increasing its solubility in water and the lipophilic ester groups increasing its affinity to oil.
Introduction
Considering the decreasing global crude oil resources, refiners are increasingly being forced to process opportunity crudes, such as DOBA, to be competitive. However, these opportunity crudes pose many problems, such as the fouling of heat exchangers, difficulties in effluent treatment, poisoning of the catalyst by certain metallic salts among other problems.1 Among the metals, calcium causes particular problems and is present in these feedstocks as organically-bond compounds such as calcium naphthenate, which are not easily dissociated or removed by conventional water washing or desalting processes.2,3 These calcium compounds can quickly be decomposed during typical catalytic operations, such as during hydroprocessing or during fluid catalytic cracking, causing rapid fouling or deactivation of the catalysts in the catalytic operation.4 Several treatment approaches have been made to reduce total metal levels and these all center on the removal of metals at the desalter unit. Normally, the desalter only removes water soluble inorganic salts, such as sodium or potassium chlorides. However, some crude oils contain water insoluble metal organic acid salts, such as calcium naphthenante and iron naphthenate, which are soluble or dispersed as fine particulate matter in the oil but not in water. Thus, it would be desirable to develop a composition and method that would cause most or all of the metals in the crude oil to transfer from the oil phase in a desalter operation, with little or no oil carry-under in the aqueous phase. Various agents, including carboxylic acids; nonyl phenol resins; phosphoric acid and its salts; a combination of mineral acid, alkyl phosphate ester, and an oxidant; hybrids of alkyl phosphate esters; alkyl carboxylic acid and mineral acids; and relatively high concentrations of sulfuric acid, are generally utilized for removing organically-bound calcium and other metal contaminants from crude oil.5–10 However, these materials have strong corrosive properties, low efficiency; and cause environmental pollution problems. In particular, phosphonates can create serious problems, such as due to the insolubility of phosphonates complexes, and also they are susceptible to breakdown to form orthophosphates under the influence of hydrolysis and/or chlorination, thereby increasing the potential of the formation of calcium-phosphate deposits in the presence of excess amounts of calcium.11–13 In addition, phosphonates, when reverted to orthophosphates, are potential nutrients for algae.14 Thus, there is an urgent need for developing a new phosphorus-free and effective agent for the removal of metal contaminants, particularly calcium, from crude oil.
Copolymers were developed in the late 1970s to take advantage of the strong complexation of multifunctional groups and superior dispersion characteristic of macromolecules.15 Several polymers have been used as a specific chemical additive agent in the crude oil desalting process, such as poly(acrylic acid) derivatives, poly-glycolic esters, poly thioglycolic acid, and poly hydroxyl-carboxylic acids.1,4,10 In particular, Subramaniyam et al. found that the use of any of the esters of poly carboxylic acids is very effective in the removal of metals like calcium and iron from hydrocarbon feedstock.1 These kinds of chemical additive agent are highly efficient and have low corrosiveness, which are attributed to their controllable pH range and multiple functional groups. In addition, some of them can perform as metal corrosion inhibitors.16,17 However, the required dosage is so large when these polymer derivatives are used as additive agents that it would greatly increase the cost of the water treatment, meanwhile, the heavy precipitation problem arising in the reaction also would lead to fouling of the processing equipment. In view of the above, there is a need to develop a new environmentally friendly “green” polymer comprising hydrophilic and lipophilic functional groups such as amino, carboxylic, and ester groups.
In the present study, we developed an acrylic acid–allylpolyethoxy amino carboxylate–2-hydroxyethyl acrylate (AA–APEA–HEA) terpolymer containing hydrophilic and lipophilic functional groups with water as solvent and ammonium persulfate as initiator by a free-radical polymerization. This was then used to remove calcium from Luning pipeline crude oil, and several effect factors, such as molar ratio, dosage, and reaction temperature, were evaluated. The results showed that the AA–APEA–HEA terpolymer, in which an ester group was introduced, could be successfully employed as a high efficiency decalcifying agent for removing calcium from crude oil. Furthermore, herein, a possible decalcification mechanism of the AA–APEA–HEA terpolymer is illustrated and discussed.
Experimental section
Materials
Allyloxy polyethoxy ether (APEG, MW = 400 g mol−1), L-asparagine (MW = 132.12 g mol−1), acrylic acid (AA, MW = 72.06 g mol−1), 2-hydroxyethyl acrylate (HEA, MW = 116.12 g mol−1), and ammonium persulfate were analytically pure grades and purchased from Aladdin Chemical Regent Co., Ltd. (Shanghai, China). The polyacrylic acid (PAA, MW = 1800 g mol−1), hydrolyzed polymaleic acid (HPMA, MW = 600 g mol−1), and polyepoxysuccinic acid (PESA, MW = 1500 g mol−1) were technical grade and supplied by Jiangsu Jianghai Chemical Co., Ltd., (Changzhou, Jiangsu, P. R. China). Distilled water was used for all the studies.
Preparation of APEA
The carboxylic acid functionalization of the surface hydroxyl groups was realized with L-asparagine as shown in Fig. 1. Typically, 0.1 mol of L-asparagine was dissolved into 30 mL of HCl (3 wt%) solution, and then 0.1 mol of APEG was added into L-asparagine solution under continuous stirring. Subsequently, the reactant mixture was loaded into a Teflon autoclave, sealed, and placed in a microwave oven (MCR-3, 900 W). The autoclave was heated to 90 °C in 2 min and kept at this temperature for 10 min. After cooling to room temperature, the sample of APEA was obtained.
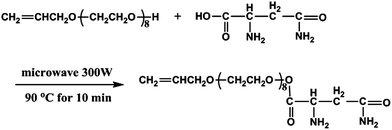 |
| Fig. 1 Preparation of APEA. | |
Preparation of AA–APEA–HEA
A typical synthetic process is as follows: 5 mol of distilled water and 0.1 mol of APEA were mixed together in a 250 mL five-neck round-bottom flask fitted with a thermometer and a magnetic stirrer. The mixture was heated to 70 °C with stirring under nitrogen atmosphere. Subsequently, 0.2 mol of AA and 0.05 mol of HEA in 20 mL distilled water (the molar ratio of AA, APEA, and HEA was 2
:
1
:
0.5) and the initiator solution (3.5 g of ammonium persulfate in 20 mL distilled water) were added separately at constant flow rates over a period of 1.0 h. The reaction was then heated to 85 °C and maintained at this temperature for another 2 h, ultimately affording an aqueous polymer solution containing approximately 27.6% solid. Finally, the product of AA–APEA–HEA was obtained. The AA–APEA–HEA product has a slight odor, is buff, and is a non-phosphorus liquid. Thus, it should not influence the air condition or result in potential nutrients for algae. Therefore, the AA–APEA–HEA terpolymer is an environmentally-friendly product. The preparation procedure of AA–APEA–HEA is shown in Fig. 2. Meanwhile, APEA–AA copolymer was also synthesized in the same condition without HEA.
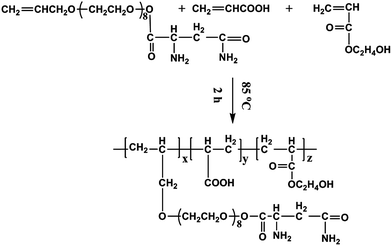 |
| Fig. 2 Preparation of AA–APEA–HEA. | |
Sample characterization
The samples were analyzed using FT-IR spectroscopy (VECTOR-22, Bruker Co., Germany) in the region of 4000–500 cm−1. Prior to the measurement, the samples were dried under vacuum until reaching a constant weight. The dried samples were pressed into the powder, mixed with KBr powder, and then compressed to make a pellet for FT-IR characterization. Structures of APEG, APEA, and AA–APEA were also explored with a Bruker NMR analyzer (AVANCE AV-500, Bruker, Switzerland) operating at 500 MHz. Molecular weight determinations were performed by gel permeation chromatography (GPC, Shodex KF-850 column) calibrated using polyethylene glycol (PEG) standards ranging in molecular mass from 1.32 × 102 to 1.34 × 106 g mol−1. Water was used as a mobile phase at a flow rate of 1.00 mL min−1. The calcium contents of the oil samples were analyzed by means of an inductively coupled plasma mass spectrometry (ICP-MS, Thermo Elemental X7 Series).
Procedure for removing calcium from crude oil
Removal experiments were carried out in a DPY-2 electric desalter instrument. 40 g of the Luning pipeline oil sample (the properties, such as density, viscosity, and metal content, are shown in Table 1, which was supplied by the Sinopec Group) was placed in a constant temperature bath. After mixing uniformly, 2 mL of wash water and the desired dose of demulsifier were added to the mixture and stirred for 2 min at a high stirring rate (about 2000 rpm) to form a homogeneous emulsion and then the mixed crude oil was transferred into a sample bottle equipped with an electrode. Subsequently, the desired dose of AA–APEA–HEA terpolymer was added into the sample bottle and fixed in the oscillator, then shook for 5 min in order to make the terpolymer and oil mix completely. The mixture was placed in a constant temperature bath at 100 °C for 10 min (to make the AA–APEA–HEA sample react with Ca2+ completely), and then placed in an electric field for 20 min for effective oil–water separation. Finally, the mixture remained at a constant temperature for 15 min and the amounts of calcium in the oil were analyzed. Comparative experiments for removing calcium by using AA, APEA, PAA, HPMA, PESA, and AA–APEA samples were also carried out.
Table 1 Properties of the Luning pipeline oil sample
Item |
Value |
ρ20, g cm−3 |
0.8839 |
ν50, mm2 s−1 |
13.89 |
Water, wt% |
1.6 |
Ca, μg g−1 |
23.1 |
Fe, μg g−1 |
2.7 |
Ni, μg g−1 |
6.0 |
Na, μg g−1 |
6.4 |
Measuring the calcium content in crude oil
10.00 g of crude oil was weighed into a quartz crucible, slowly heated, and then ignited. After combustion, the crude oil was calcined in a muffle furnace at 525 ± 25 °C to remove carbon residues. After the ash was allowed to cool, a small volume of distilled water was added to wet the ash and nitric acid and the hydrochloric acid solutions were then added. The crucible was heated slowly to dissolve the ash and the solution was removed quantitatively into a volumetric flask. The residual calcium content of crude oil was analyzed with an inductively coupled plasma mass spectrometry (ICP-MS).
Results and discussion
FT-IR and 1H-NMR measurements
The FT-IR spectra of APEG (a), APEA (b), and AA–APEA–HEA (c) are exhibited in Fig. 3. Compared with curve (a), the emerging 1731 cm−1 strong intensity absorption peak (–C
O) in curve (b) indicates that APEA has been synthesized successfully. The fact that the (–C
C–) stretching vibration at 1638 cm−1 appears in curve (b) but disappears completely in curve (c) reveals that free radical copolymerization between APEA, AA, and HEA has happened.18
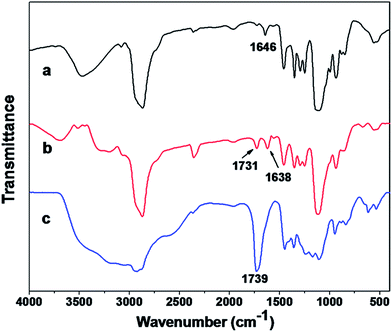 |
| Fig. 3 The FT-IR spectrums of (a) APEG (b) APEA, and (c) AA–APEA–HEA. | |
Fig. 4 shows the 1H-NMR spectra of (a) APEG, (b) APEA, and (c) AA–APEA–HEA. APEG (Fig. 4(a)) ((CD3)2SO, δ, ppm): 2.40–2.60 (solvent residual peak of (CD3)2SO),19 3.00–3.80 (–OCH2CH2–, ether groups), 3.80–6.00 (CH2
CH–CH2–, propenyl protons), and 4.40–4.60 (–OH, active hydrogen in APEG). APEA (Fig. 4(b)) ((CD3)2SO, δ, ppm): 2.40–2.60 (solvent residual peak of (CD3)2SO), 3.50–3.90 (–OCH2CH2–, ether groups), while the disappeared peak at 4.41–4.60 ppm in Fig. 4(b) reveals that the active hydroxyl group of APEG has reacted with L-asparagine, which confirms the FT-IR analysis of the emerging 1731 cm−1 strong intensity absorption peak (–C
O) in Fig. 3(b). AA–APEA–HEA (Fig. 4(c)) ((CD3)2SO, δ, ppm): 2.40–2.60 (solvent residual peak of (CD3)2SO), δ, 4.10–6.00 ppm in (b) double bond absorption peaks completely disappeared in (c). This reveals that free radical polymerization among APEA, AA, and HEA has happened. From the FT-IR and 1H-NMR analyses, it can be concluded that synthesized AA–APEA–HEA has the anticipated structure.
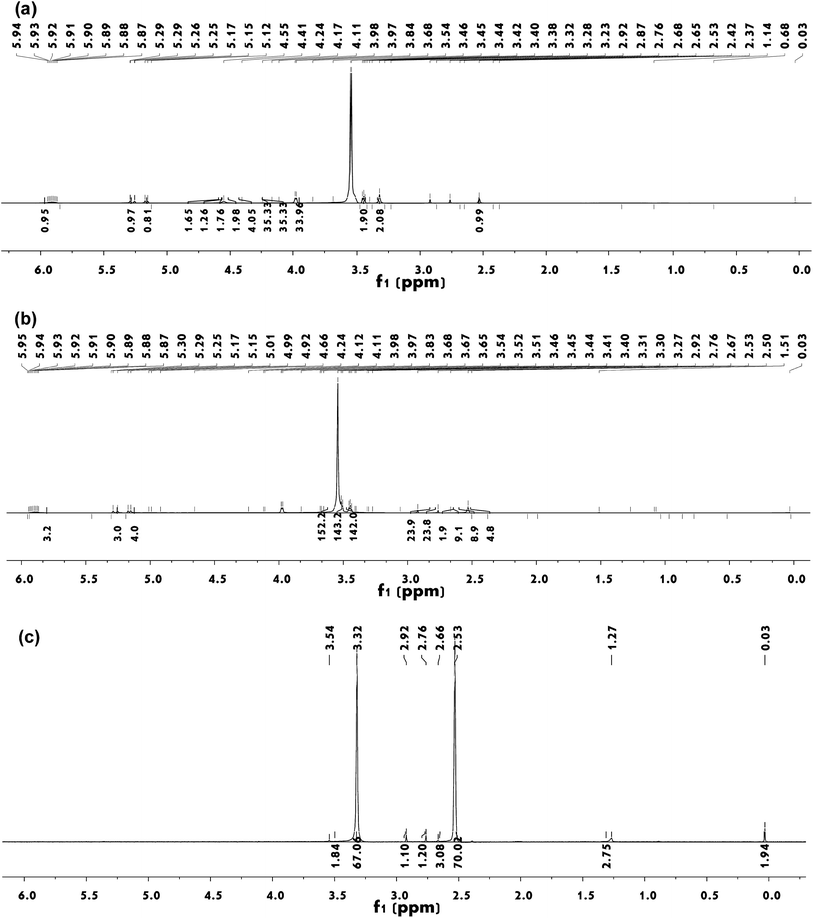 |
| Fig. 4 The 1H-NMR spectra of (a) APEG, (b) APEA, and (c) AA–APEA–HEA. | |
Influence of the molar ratio of AA–APEA and AA–APEA–HEA on the calcium removal efficiency
Data of the calcium removal efficiency of AA–APEA and AA–APEA–HEA prepared at different molar ratios at a dosage of 100 ppm and temperature of 100 °C are summarized in Fig. 5. Different monomer molar ratios can result in a different conversion rate, and different proportion of the functional groups and polymer sequence structure. Polymers have good scale performance only when the proportions of the functional groups in long-chain macromolecules are appropriate. As can be seen from Fig. 5(a), the molar ratio of AA
:
APEA has an impact on the properties of AA–APEA, and copolymer AA–APEA exhibits superior calcium removal efficiency when the molar ratio of AA
:
APEA is 2
:
1. The properties of AA–APEA–HEA prepared at different AA
:
APEA molar ratios are shown in Fig. 5(b). From Fig. 5(b), it can be found that the AA–APEA–HEA terpolymer shows a better decalcifying property for crude oil than the AA–APEA copolymer. This demonstrates that HEA can enhance the calcium removal efficiency of the AA–APEA copolymer prepared at different molar ratios. Fig. 5(c) illustrates the influence of different contents of HEA in the AA–APEA–HEA terpolymer, with the AA–APEA fixed at 2
:
1. As seen from Fig. 5(c), the calcium removal efficiency increases from 80.31% to 98.63% when increasing the molar ratio of AA–APEA–HEA from 2
:
1
:
0.1 to 2
:
1
:
0.5. This result may be attributed to the increasing content of HEA gives the terpolymer more opportunities to come into contact with oil, making the effective groups more active and enhancing its decalcifying properties. However, when the molar ratio of AA–APEA–HEA is raised from 2
:
1
:
0.5 to 2
:
1
:
3, the corresponding calcium removal efficiency decreases from 98.63% to 41.17%. This may be due to the high content of HEA making the solubility of the calcium complex decrease, such that the calcium complex cannot separate out the discharge water. Besides, the molecular weight and molecular weight distribution influence of the calcium removing ability. Thus the molecular mass distributions of the AA–APEA–HEA samples were investigated via GPC, and the results are illustrated in Table 2. As can be seen from Table 2, the weight-average molecular weight (Mw) ranged from 4200 Da to 21
700 Da with the variation of AA
:
APEA
:
HEA ratios. With the increase of the molecular weight of AA–APEA–HEA, a trend should not be ignored, which is that the Mw/Mn values were also increased and the molecular weight distribution was relatively broad (Mw/Mn = 1.37) when the molar ratio of AA
:
APEA
:
HEA reached 1
:
5
:
1. According to the calcium removal efficiency data of the AA–APEA–HEA terpolymer, a low or high molecular weight might have a negative impact on the decalcifying performance, which could be attributed to the low molecular weight samples containing less effective groups while the high molecular weight samples might wrap the active groups inside the molecular chains. In addition, the relatively broad molecular weight distribution could influence the stability of the AA–APEA–HEA terpolymer and thus reduce its decalcifying properties. These results manifest that AA–APEA–HEA terpolymer with a molar ratio of 2
:
1
:
0.5 shows superior efficacy in removing calcium from crude oil over the other molar ratios.
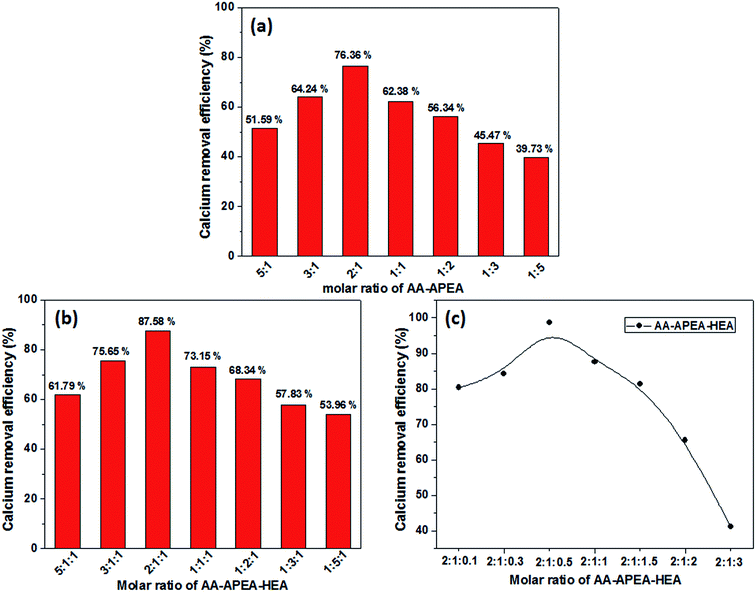 |
| Fig. 5 The influence of molar ratio of (a) AA–APEA and (b and c) AA–APEA–HEA on the calcium removal efficiency. | |
Table 2 Average molecular weights of the AA–APEA–HEA terpolymer
Entry |
AA : APEA : HEA |
Mwa (Da) |
Mnb (Da) |
Mw/Mnc |
Weight average molecular weight, determined by GPC with PEG standards. Number average molecular weight, determined by GPC with PEG standards. Molecular weight distribution, determined by GPC. |
1 |
5 : 1 : 1 |
4200 |
3900 |
1.08 |
2 |
3 : 1 : 1 |
7600 |
6800 |
1.12 |
3 |
2 : 1 : 1 |
10 200 |
9400 |
1.09 |
4 |
1 : 1 : 1 |
13 400 |
12 600 |
1.06 |
5 |
1 : 2 : 1 |
15 600 |
13 500 |
1.16 |
6 |
1 : 3 : 1 |
18 300 |
14 900 |
1.23 |
7 |
1 : 5 : 1 |
21 700 |
15 800 |
1.37 |
Influence of the dosage of AA–APEA–HEA on calcium removal efficiency
Data of the calcium removal efficiency under 100 °C in the presence of varying dosages of AA, APEA, AA–APEA (2
:
1), and AA–APEA–HEA (2
:
1
:
0.5) are shown in Fig. 6. As can be observed, the calcium removal efficiency is only 12.32% without the use of any chemical additive. However, the decalcification rate increases quite significantly when the terpolymer of AA–APEA–HEA (2
:
1
:
0.5) is added. The calcium removal efficiency can reach up to 98.63% when the dosage of AA–APEA–HEA (2
:
1
:
0.5) is at levels of up to 100 ppm, while the AA, APEA, and AA–APEA (2
:
1
:
0.5) can only approach 19.27%, 23.33%, and 76.36% for the same condition, respectively. However, the AA–APEA (2
:
1) copolymer shows a relatively high calcium removal efficiency of 95.69% when the dosage is up to 150 ppm. The phenomenon that AA–APEA–HEA (2
:
1
:
0.5) displays the best calcium removal efficiency can be ascribed to the AA–APEA–HEA (2
:
1
:
0.5) terpolymer containing more amino, carboxylic, and ester groups and the synergy between them.20,21 In addition, the decalcification rate does not obviously increase correspondingly when the concentration of AA–APEA–HEA (2
:
1
:
0.5) exceeds 100 ppm. Therefore, the terpolymer of AA–APEA–HEA (2
:
1
:
0.5) can be considered as an extremely effective decalcifying agent for removing calcium from crude oil and thus results in a much lower calcium content of the desalted oil.
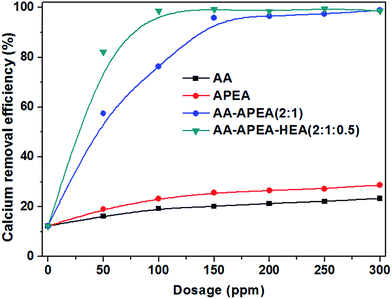 |
| Fig. 6 Calcium removal efficiency in the presence of varying dosages of AA, APEA, AA–APEA (2 : 1) and AA–APEA–HEA (2 : 1 : 0.5). | |
Effect of reaction temperature on the calcium removal efficiency
Experiments were conducted to study the patterns of how the reaction temperature affects the calcium removal efficiency when utilizing AA–APEA (2
:
1) and AA–APEA–HEA (2
:
1
:
0.5) as decalcifying agents, with the dosage of the AA–APEA and AA–APEA–HEA sample being 100 ppm, and the results are shown in Fig. 7. It can be found that the removal efficiency of calcium by AA–APEA (2
:
1) and AA–APEA–HEA (2
:
1
:
0.5) are 26.35% and 44.56%, respectively, when the reaction temperature is 60 °C. However, the removal efficiency of calcium by the AA–APEA–HEA (2
:
1
:
0.5) terpolymer increases to 98.63% when the temperature is increased up to 100 °C. Meanwhile, the result with AA–APEA (2
:
1) can only approach 76.36% for the same condition. This result may be attributed to the increasing temperature making the polymer gain much more energy, leading to increased stretching, making the effective groups more active and enhancing its decalcifying property. However, both the AA–APEA (2
:
1) and AA–APEA–HEA (2
:
1
:
0.5) samples exhibit a significant decrease in calcium removal efficiency when the temperature rises from 120 °C to 180 °C. This can be ascribed to the high temperature not being beneficial for the coalescence of water droplets and thereby the dissolved calcium compounds are difficult to separate from the discharge water promptly. In addition, it is worth noting that AA–APEA (2
:
1) and AA–APEA–HEA (2
:
1
:
0.5) exhibited similar calcium removing efficiency at temperatures above 150 °C. This phenomenon may be attributed to the high reaction temperature reducing the viscosity of the crude oil and supplying more energy for the polymer macromolecule at the same time, and thus both the AA–APEA and AA–APEA–HEA polymers could gain more opportunities to come into contact with the oil. However, the purpose of introducing HEA is to use the lipophilic ester group of the terpolymer to gain more opportunities to come into contact with the oil and to have some synergy effects on the decalcifying performance. However, the advantage of the lipophilic ester group of HEA could be ignored in this high temperature condition. On the other hand, the electric desalting temperature of a refinery typically ranges from 90 °C to 130 °C. Therefore, the optimum temperature was set at 100 °C and the resulting calcium removal efficiency from the crude oil was 98.63%.
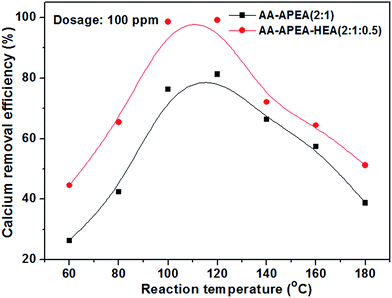 |
| Fig. 7 Effect of reaction temperature on the calcium removal efficiency from crude oil. | |
Comparison with other polymers
The effect of the terpolymer (AA–APEA–HEA (2
:
1
:
0.5)) as an agent for removing calcium from crude oil was compared with that of other polymer decalcifying agents, namely HPMA, PAA, PESA, and AA–APEA (2
:
1), at a reaction temperature of 100 °C, and the results are shown in Fig. 8. All the data in Fig. 8 show that the ability to remove calcium from crude oil followed the order: AA–APEA–HEA (2
:
1
:
0.5) > AA–APEA (2
:
1) > HPMA > PAA > PESA (under 250 ppm). As can be seen, the calcium removal efficiency for the copolymer AA–APEA–HEA (2
:
1
:
0.5) could reach 98.63% at a dosage of 100 ppm, while AA–APEA (2
:
1), HPMA, PAA, and PESA could only approach 76.36%, 26.53%, 21.66%, and 18.27%, respectively. In addition, the calcium removal efficiency did not obviously increase with the increasing concentrations when the concentration of AA–APEA–HEA (2
:
1
:
0.5) exceeded 100 ppm. However, the calcium removal efficiency for AA–APEA (2
:
1) copolymer could reach 95.69% at a dosage of 150 ppm. Furthermore, the HPMA, PAA, and PESA polymers also had relatively superior ability for the removal of calcium from crude oil, with 58.25%, 56.15%, and 47.23% removal at a level of 250 ppm, respectively. It is also worth mentioning that PESA displays better calcium removal efficiency than both HPMA and PAA when the dosage is over 300 ppm. These results demonstrated that the amino groups existing in the AA–APEA–HEA (2
:
1
:
0.5) terpolymer generate more active sites, while the lipophilic ester group of HEA could make the terpolymer gain more opportunities to come into contact with oil and could have some synergy effects on the decalcifying performance. Therefore, the AA–APEA–HEA (2
:
1
:
0.5) terpolymer displayed the best ability to remove calcium from crude oil among the polymers investigated, i.e., AA–APEA (2
:
1), HPMA, PAA, and PESA.
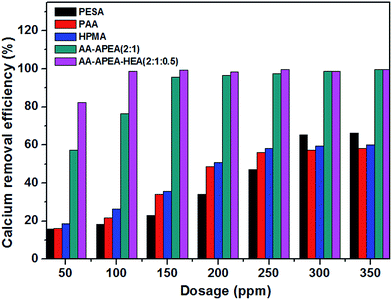 |
| Fig. 8 Comparison of the calcium removal efficiencies between different samples. | |
Proposed mechanism for decalcification
It was previously discovered that the addition of water-soluble AA–APEA–HEA terpolymer to crude oil can significantly reduce the amount of calcium when it is run through an electric desalter instrument. Based on the above results, a possible mechanism for removing calcium from crude oil was proposed and is illustrated in Fig. 9. As can be seen, the AA–APEA–HEA terpolymer has a large number of carboxyl, amino, and ester groups. It can be considered as a member of a broad class of multidentate chelating ligands that complex or coordinate metal ions, with these metal complexes being very stable.22–24 Herein, crude oil was mixed with an aqueous solution of AA–APEA–HEA and produced an aqueous/organic multi-phase mixture. With vigorous mixing of the oil and water, the ester groups of HEA make the terpolymer adsorb on the surface of olesome. Then, the acids formed after hydrolysis of the chemical compound and the calcium ions of calcium naphthenate were substituted for H+ by an acid displacement reaction. Once confronted with calcium ions, the carboxyl and amino groups of AA–APEA can recognize and react with the positively charged ions, which leads to the spontaneous formation of AA–APEA–HEA–Ca complexes. The PAA and APEA blocks are hydrophilic chain segments, thus the AA–APEA–HEA–Ca complexes are also water soluble, and the calcium in the organic phase is transported across the interface between the two phases and dissolves in the aqueous phase, and therefore it could be washed and removed from the crude oil by an electric desalting process. In this case, the calcium removal efficiency is more pronounced with more carboxyl- and amino-containing groups.
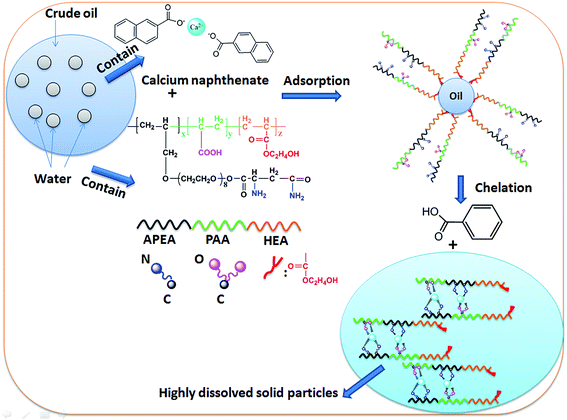 |
| Fig. 9 Schematic of the decalcification mechanism. | |
Conclusions
In summary, the water-soluble monomer APEA was produced from APEG and L-asparagine by esterification via the microwave technique route. A phosphorus-free terpolymer of AA–APEA–HEA was synthesized with different molar ratios in this study and the synthesized samples were characterized by FT-IR and further confirmed by 1H-NMR. The majority of inorganic metals in crude oil can be removed with an electric desalting process. However, organically-bond compounds, such as calcium naphthenate, are only oil soluble and thus they cannot be removed by the electric desalting process. After the AA–APEA–AA terpolymer was introduced to crude oil, the removal efficiencies of calcium from Luning pipeline crude oil samples could reach approximately 98.63% at the optimum conditions as follows: (a) molar ratio of AA–APEA–HEA, 2
:
1
:
0.5; (b) dosage of AA–APEA–HEA, 100 ppm; (c) reaction temperature, 100 °C. Compared to the nonphosphorus polymer of PAA, HPMA, PESA, and AA–APEA, the AA–APEA–HEA terpolymer possessing carboxyl, amino, and ester groups showed superior calcium removal efficiency from crude oil. The decalcification mechanism is proposed whereby the lipophilic ester group of HEA could make the terpolymer gain more opportunities to come into contact with oil and have some synergy effects on the adsorption, displacement, and chelation between AA–APEA–HEA and calcium ions. Also, water soluble AA–APEA–HEA–Ca complexes can be formed; hence, the calcium is removed via the water phase. Therefore, the AA–APEA–HEA terpolymer is believed to represent a potentially environmental decalcifying agent of crude oil.
Acknowledgements
The authors are grateful to the financial supports of the National Natural Science Foundation of China (Grant No. 21376051, 21306023, 21106017), the Natural Science Foundation of Jiangsu (Grant No. BK20131288), Project Funded by the Priority Academic Program Development of Jiangsu Higher Education Institutions (PAPD, 1107047002), Fund Project for Transformation of Scientific and Technological Achievements of Jiangsu Province of China (Grant No. BA2014100) and the Fundamental Research Funds for the Central Universities (3207045301, T15192014, KYLX_0161).
References
- M. Subramaniyam, US Pat. 8440072, 2013
. - L. N. Kremer, J. J. Weers and C. Sandu, US Pat. 8425765, 2013
. - D. L. Kuehne, L. P. Hawker and D. C. Kramer, US Pat. 6905593, 2005
. - M. Subramaniyam, US Pat. 8685233, 2014
. - R. B. Duke, US Pat. 4439345, 1984
. - A. Goliaszewski, D. Engel and R. May, US Pat. 6905593, 2005
. - F. J. Krambeck, C. T. Lam and P. H. Schipper, US Pat. 4645589, 1987
. - G. R. Norman, US Pat. 4432865, 1984
. - R. Powell, US Pat. 2778777, 1957
. - M. Subramaniyam, US Pat. 8840781, 2014
. - B. R. Zhang, L. Zhang, F. T. Li, W. Hu and P. M. Hannam, Corros. Sci., 2010, 52, 3883–3890 CrossRef CAS
. - A. Tsortos and G. H. Nancollas, J. Colloid Interface Sci., 2002, 250, 159–167 CrossRef CAS PubMed
. - Z. Amjad, Langmuir, 1989, 5, 1222–1225 CrossRef CAS
. - A. A. Koelmans, A. Van der Heijde, L. M. Knijff and R. H. Aalderink, Water Res., 2001, 35, 3517–3536 CrossRef CAS PubMed
. - M. M. Reddy and G. H. Nancolla, Desalination, 1973, 12, 61–73 CrossRef CAS
. - Y. Y. Chen, Y. M. Zhou, Q. Z. Yao, Y. Y. Bu, H. C. Wang, W. D. Wu and W. Sun, Desalin. Water Treat., 2015, 55, 945–955 CrossRef CAS
. - Y. Y. Chen, Y. M. Zhou, Q. Z. Yao, Y. Y. Bu, H. C. Wang, W. D. Wu and W. Sun, J. Appl. Polym. Sci., 2015, 132, 41447 Search PubMed
. - H. C. Wang, Y. M. Zhou, G. Q. Liu, J. Y. Huang, Q. Z. Yao, S. S. Ma, K. Cao, Y. H. Liu, W. D. Wu, W. Sun and Z. J. Hu, Desalin. Water Treat., 2015, 53, 3491–3498 CrossRef CAS
. - G. Q. Liu, J. Y. Huang, Y. M. Zhou, Q. Z. Yao, L. Ling, P. X. Zhang, H. C. Wang, K. Cao, Y. H. Liu, W. D. Wu, W. Sun and Z. J. Hu, Tenside, Surfactants, Deterg., 2012, 49, 404–412 CrossRef CAS
. - S. C. Wang, J. Y. Yang and X. R. Xu, Fuel, 2011, 90, 987–991 CrossRef CAS
. - S. C. Wang, X. R. Xu, J. Y. Yang and J. S. Gao, Fuel Process. Technol., 2011, 92, 486–492 CrossRef CAS
. - F. Dwyer, Chelating agents and metal chelates, Elsevier, 2012 Search PubMed
. - A. E. Martell, J. Am. Chem. Soc., 1965, 87, 2527–2528 CrossRef
. - G. Leaf, Nature, 1965, 207, 564–565 CrossRef
.
Footnote |
† S. S. Ma and Z. L. Cai contributed equally to this work. |
|
This journal is © The Royal Society of Chemistry 2016 |
Click here to see how this site uses Cookies. View our privacy policy here.