DOI:
10.1039/C6RA05865E
(Paper)
RSC Adv., 2016,
6, 37006-37011
Synthesis, structure and conformational mobility of tetra-substituted cyanomethoxy p-tert-butylcalix[4]arenes†
Received
5th March 2016
, Accepted 31st March 2016
First published on 4th April 2016
Abstract
The syntheses and single crystal X-ray structure determinations of 5,11,17,23-tetra-tert-butyl-25,26,27,28-tetrakis(cyanomethoxy)calix[4]arene (partial-cone conformer), 5,11,17,23-tetra-tert-butyl-25,27-bis(diethylcarbamoylmethoxy)-26,28-bis(cyanomethoxy)calix[4]arene (cone and partial-cone conformers), 5,11,17,23-tetra-tert-butyl-25,26,27-tris(cyanomethoxy)-28-hydroxycalix[4]arene, and 5,11,17,23-tetra-tert-butyl-25-diethylcarbamoylmethoxy-26,27,28-tris(cyanomethoxy)calix[4]arene (cone conformer) are reported. The calixarenes are found to be conformationally mobile when heated in DMSO, favouring the partial-cone conformer at equilibrium. The tris-substituted cyanomethoxy derivative was found to assume the partial-cone conformation in the solid state. Attempts to convert nitrile groups to tetrazole moieties in derivatives containing both amide and nitrile groups were unsuccessful.
Introduction
Calixarenes are well established as useful and versatile macrocyclic frameworks upon which a range of functional groups can be added at both the upper and lower rims.1 Substitution at the lower rim with cyanomethoxy groups has attracted attention, both as a useful precursor for a range of functionalities,2 and because this group is one of the largest to still allow conformational mobility of the calix[4]arene.3 We have recently reported the conversion of the nitrile group to tetrazole moieties giving calix[4]arene ligands that support mononuclear and polynuclear lanthanide complexes.4 In an effort to extend this work, we have investigated the synthesis of some new cyanomethoxy-substituted p-tert-butylcalix[4]arenes. Here we report the synthesis and characterisation of some mixed amide/nitrile functionalised calix[4]arenes, as well as further investigation of the synthesis of the known tetra-cyanomethoxy derivative. Conformational mobility of the cyanomethoxy derivatives is also investigated.
Results and discussion
Synthesis and conformational properties
The starting material for all of the tetra-substituted calixarenes was the bis-cyanomethoxy calix[4]arene, 1, synthesised following the literature procedure (Scheme 1).5 The tetra-cyanomethoxy calix[4]arenes, 2, 3 were synthesised using a modification of the literature procedure;2c it was found that somewhat improved yields could be achieved by carrying out the reaction at room temperature, rather than at 75 °C in DMF. Tetrahydrofuran was also found to be a satisfactory substitute for DMF as a solvent for this reaction, which gave improved combined yields of 2 and 3 (increasing from 69% overall yield in DMF to 91% in THF) but with increased amounts of the partial-cone conformer, 3, being produced. The typical ratio of 2
:
3 found was 9
:
1. The conformers 2 and 3 were readily separated by column chromatography.
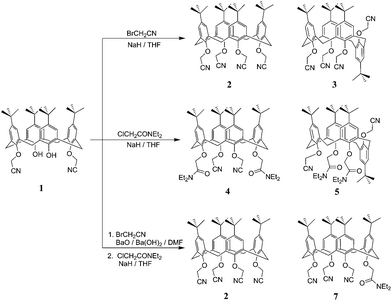 |
| Scheme 1 Reaction scheme for the syntheses of the cyanomethoxy-substituted calixarenes. | |
Amide functional groups were introduced by reacting 1 with N,N-diethylchloroacetamide, in the presence of sodium hydride in DMF. This reaction proceeded in good overall yield producing the bisamide cone 4 and partial-cone 5 conformers in an approximately 1
:
3 ratio. Again, these conformers were readily separated by column chromatography. The mono-amide tris-cyanomethoxy derivative 7 proved to be a little more challenging to access. Alkylation of 1 was carried out using BaO/Ba(OH)2 as the base, which has been reported to favour tris-substitution of calix[4]arenes.6 This was found to be the case, and the tris–cyanomethoxy calix[4]arene 6 was the dominant product of this alkylation, along with some tetra-substituted cone conformer, 2. Separation of 2 and 6 was not achieved by recrystallisation or chromatography (although a single crystal X-ray structure of 6 was obtained by selecting a crystal from the mixture, see below). Therefore, the mixture was further reacted to introduce the amide functional group to make 7, which was then readily separated from the tetra-cyanomethoxy 2, by chromatography.
The debutylated analogue of the tetra-cyanomethoxy 2 was reported to be conformationally labile in solution at room temperature, with increasing concentration of the partial-cone conformer appearing over some days, with the time taken to achieve equilibrium greatly reduced if the solution was heated.3b The equilibrium ratio of partial-cone
:
cone in deuterated chloroform was found to be 3
:
2. Here, no change in the spectrum of 2 in d-chloroform was observed over the course of 3 days. Upon heating either 2 or 3 in deuterated DMSO to 150 °C, conformational mobility was observed, with both conformers converging to a partial-cone
:
cone ratio of 3
:
1 (see ESI†). These changes were accompanied by degradation of the substituted calixarenes, apparently to the unsubstituted p-tert-butylcalix[4]arene based on the NMR spectra. Given the degradation observed at temperatures required to achieve equilibrium in a reasonable timeframe, more detailed studies to extract thermodynamic or kinetic parameters were not undertaken. Under similar conditions, the ratio of 5
:
4 at equilibrium was 3
:
2. A similar partial-cone
:
cone ratio achieved for the mono-amide 7, but convergence to equilibrium could not be confirmed as the partial-cone conformer has not been isolated. It is interesting to note that attempts to convert the nitrile groups to tetrazole moieties in the calixarenes containing amide groups (4, 5, and 7) under the conditions reported previously for 1 (ref. 4a) and 2 (ref. 7) were unsuccessful, with only the starting materials being recovered. The origins of this lack of reactivity have yet to be determined.
Crystal structures
Crystals of compounds 3–7, suitable for single crystal X-ray structure determination were obtained by slow evaporation of 1
:
1 chloroform/ethanol solutions. The crystal structure of 2 has been reported previously as a dichloromethane solvate.2c The calixarene in that case exhibits the common “pinched cone” conformation, with two almost parallel phenyl rings having dihedral angles between the phenyl rings and the methylene C4 plane of 82.9 and 89.3°. Representations of the molecular structures found in this work are shown in Fig. 1.
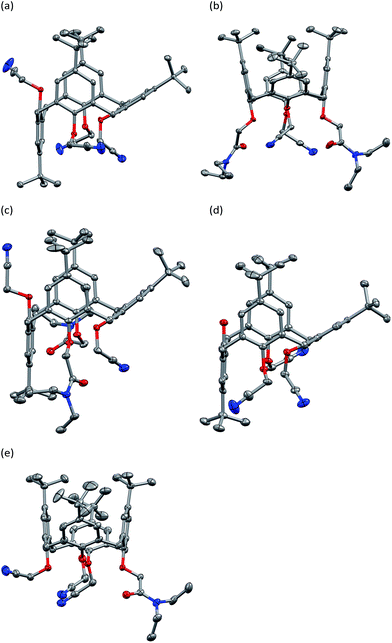 |
| Fig. 1 Representations of the molecular structures obtained by single crystal X-ray structure determinations for (a) 3, (b) 4, molecule 1 of two independent molecules, (c) 5·EtOH, (d) 6, and (e) 7·EtOH. Solvent molecules, hydrogen atoms and minor components of the disordered atoms have been omitted. Ellipsoids have been drawn at the 50% probability level. | |
Considering first the calixarenes in the cone conformation, 4 and 7, the “pinched” cone is observed in both cases. The bis amide 4 crystallised with two crystallographically independent, but very similar, molecules in the asymmetric unit which are related by a pseudo a/2 translation (see ESI†). The two opposing phenyl rings containing the amide groups are almost co-planar, the dihedral angle between the two planes being 15.98(4) and 15.13(4)° for molecule 1 and 2 respectively. This is also reflected in the dihedral angles between the phenyl rings planes and the methylene C4 plane, the dihedral angles for the planes of rings 1 and 3 are 98.67(4) and 82.70(4)° for molecule 1 and 90.63(3) and 75.50(3)° for rings 5 and 7 of molecule 2. The dihedral angles between the C4 plane and the planes of the remaining phenyl rings are 34.50(4) and 49.75(4)° for phenyl rings 2 and 4 of molecule 1 and 34.15(4) and 48.93(2)° for phenyl rings 6 and 8 of molecule 2. The mono amide tris(cyanomethoxy) calixarene 7 again has two opposing phenyl rings which are almost co-planar, the dihedral angle between the two planes being 8.56(7)°. The dihedral angles between the planes of rings 1 and 3 and the methylene C4 plane are 84.39(6) and 87.05(6). The dihedral angles between the C4 plane and the planes of the remaining phenyl rings, 2 and 4, are 46.43(6) and 41.11(6)° respectively. There is a hydrogen bond between an ethanol solvent molecule in the lattice and the amide oxygen atom (see ESI†).
Calixarenes 3, 5, and 6 were found to be in the partial-cone conformation in the solid state. Considering first the tetra-cyanomethoxy derivative 3, the planes of phenyl rings 1, 3 and (inverted) 4 are virtually perpendicular to the methylene C4 plane, the dihedral angles being 91.42(3), 86.33(2), and 90.89(3)° (Fig. 1). The phenyl ring 2 lies at a much more acute angle with the dihedral angle between it and the (CH2)4 plane being 30.93(3)°. The conformation of the bisamide derivative 5 is similar. The two opposing phenyl rings with the amide groups attached are almost co-planar, the dihedral angle between the two planes being 8.92(6)°. The dihedral angles between these phenyl ring planes (ring 1 and 3) and the methylene C4 plane, are 93.23(5) and 84.32(5)° respectively. The inverted cyanomethoxy-substituted phenyl ring (4) is also close to perpendicular to the C4 plane at an angle of 87.16(5)°. The C4/Ph dihedral angle for the remaining phenyl (ring 2) is 36.15(5)°. An ethanol molecule in the lattice forms a hydrogen bond with one of the amide O atoms (see ESI†).
Intriguingly, the tris-cyanomethoxy calixarene 6 is also found in the partial-cone conformation, despite the inversion of the unsubstituted (and thus freely rotating) phenol ring preventing an intramolecular hydrogen bond interaction with a neighboring phenol O atom. The planes of phenyl rings 1, 3 and (inverted) 4 are again virtually perpendicular to the methylene C4 plane, the dihedral angles being 94.20(5), 88.66(5), and 87.06(5)°. The phenyl ring 2 lies at a more acute angle of 29.75(4)°. This observation has precedent, with a tripyridoxycalix[4]arene being found to crystallise in either the cone or partial-cone conformation, depending on the solvent from which crystallisation occurs.8 In that system, the partial-cone conformer formed hydrogen bonds between the unsubstituted phenol O atom and solvating methanol molecules. The cone conformer crystallized from ethyl acetate with an intramolecular hydrogen bond formed between phenol O atoms. In this tris(cyanomethoxy) derivative 6, the partial-cone conformer is observed, despite the unsubstituted phenol OH group being positioned without any apparent hydrogen bond interactions. This suggests that other weak interactions in the crystal are sufficient to overcome the single intramolecular hydrogen bond that can be formed by this molecule. Examination of the intermolecular interactions in this structure (and the others reported here) did not, however, reveal any specific interactions worthy of note.
Conclusions
A number of new cyanomethoxy lower-rim substituted p-tert-butylcalix[4]arenes have been synthesised and characterised. Consistent with previous reports, these calixarenes are found to be conformationally mobile in solution, favouring the partial-cone conformer at equilibrium. This was confirmed by approaching equilibrium from each direction, which was enabled by isolation of cone and partial-cone conformers for two systems. It was found that derivatives containing both nitrile and amide functional groups resisted attempts to convert the nitrile group to tetrazole moieties. The tris(cyanomethoxy) derivative was found to assume the partial-cone conformation in the crystalline state, presumably because weak intermolecular interactions in the crystal are more favourable than the formation of an intramolecular OH⋯O hydrogen bond.
Experimental
Synthesis
5,11,17,23-Tetra-tert-butyl-25,27-bis-cyanomethoxy-26,28-hydroxy-calix[4]arene, 1, was synthesised according to the literature method.5
5,11,17,23-Tetra-tert-butyl-25,26,27,28-tetrakis(cyanomethoxy)calix[4]arene (cone) 2
Sodium hydride (1.06 g, 44.0 mmol) and calixarene 4 (2.13 g, 2.93 mmol) were added to dry DMF (100 mL) and allowed to stir for 20 minutes. To this, bromoacetonitrile (0.96 mL, 13.7 mmol) was added and stirred overnight at room temperature under a nitrogen atmosphere. This resulted in a black completely opaque solution. Water (150 mL) was slowly added causing a pale brown precipitate to form, which was filtered off and washed several times with water. The solid, consisting of conformational isomers 2 and 3, was dissolved in a minimum amount of DCM and separated using a silica column with straight DCM as the eluent. The organic volatiles were evaporated leaving white solid calixarene 2 (1.62 g, 69%), which gave NMR spectra consistent with the literature.2c1H NMR (DMSO-d6, δ, ppm) 6.91 (s, 8H, Ar-H), 2.00 (s, 8H, Ar-O–CH2), 4.28 (d, 4H, axial Ar-CH2-Ar, J = 13.2 Hz), 3.32 (d, 4H, equatorial Ar-CH2-Ar, J = 13.3 Hz), 1.05 (s, 36H, Ar-CCH3). 13C NMR (DMSO-d6, δ, ppm) 151.5, 146.7, 133.8, 125.8, 118.0, 59.5, 34.2, 31.7, 31.4. IR (ATR): ν/cm−1 2959 (s), 2868 (m), 1604 (w), 1473 (s), 1362 (m), 1190 (s), 1024 (s), 878 (w).
5,11,17,23-Tetra-tert-butyl-25,26,27,28-tetrakis(cyanomethoxy)calix[4]arene (partial-cone), 3
Sodium hydride (0.10 g, 4.13 mmol) and calixarene 1 (0.50 g, 0.69 mmol) were added to dry THF (60 mL) and allowed to stir for 30 minutes. To this, bromoacetonitrile (0.19 mL, 2.75 mmol) was added and stirred overnight at room temperature under a nitrogen atmosphere. This resulted in a dark brown solution. The solvent was removed under reduced pressure, and the brown solid redissolved in dichloromethane. The organic was washed with water (3 × 50 mL) which had a dark brown semi solid within the water layer. The organics were removed leaving a slight brown solid. The solid was purified via column chromatography using dichloromethane, resulting in the product eluting first as a white solid which upon characterisation proves to be partial-cone calixarene 3 (0.156 g, 28%), followed by 2 (yield, 63%) mp = 288 °C. 1H NMR (DMSO-d6, δ, ppm) 7.37 (s, 2H, Ar-H), 7.23 (s, 2H, Ar-H), 7.01 (d, 2H, Ar-H, J = 2.5 Hz), 6.60 (d, 2H, Ar-H, J = 2.4 Hz), 4.81 (dd, 4H, Ar-O–CH2, J = 11.2 Hz), 4.53 (s, 2H, Ar-O–CH2), 4.08 (d, 2H, Ar-CH2-Ar, J = 13.4 Hz), 3.86 (d, 2H, Ar-CH2-Ar, J = 14.4 Hz), 3.77 (d, 2H, Ar-CH2-Ar, J = 14.4 Hz), 3.25 (d, 2H, Ar-CH2-Ar, J = 13.6 Hz), 1.41 (s, 9H, Ar-CCH3), 1.29 (s, 9H, Ar-CCH3), 1.02 (s, 18H, Ar-CCH3). 13C NMR (CDCl3, δ, ppm) 153.5, 152.0, 151.2, 147.5, 146.0, 145.2, 135.4, 133.1, 132.5, 131.9, 128.2, 126.6, 126.4, 125.8, 118.3, 117.9, 117.7, 58.7, 56.9, 56.7, 67.7, 34.5, 34.3, 34.0, 31.8, 31.7, 31.6, 31.4. IR (ATR): ν/cm−1 2957 (s), 2868 (m), 1600 (w), 1474 (s), 1362 (m), 1188 (s), 1024 (s), 875 (m). Anal. calculated for C52H60O4N4: C, 77.58; H, 7.51; N, 6.96. Found C, 77.86; H, 7.62; N, 7.01%.
5,11,17,23-Tetra-tert-butyl-25,27-bis(diethylcarbamoylmethoxy)-26,28-bis(cyanomethoxy)calix[4]arene, cone 4, partial-cone 5
THF (50 mL) was placed into a round bottom flask containing calixarene 4 (0.44 g, 0.60 mmol) and sodium hydride (0.10 g, 2.52 mmol). This mixture was stirred for 30 minutes at room temperature. N,N-Diethylchloroacetamide (0.33 mL, 2.40 mmol) and potassium iodide (0.46 g, 2.80 mmol) was added to the pale yellow cloudy solution. The reaction was heated at reflux for 12 hours. The brown solution was reduced to dryness leaving a brown solid which was redissolved in DCM. The organic fraction was washed with hydrochloric acid (1 M, 3 × 50 mL). The organic layer was dried over magnesium sulphate and distilled under reduced pressure. The resulting solid was separated into its different components using column chromatography and a 5% methanol in DCM mixture. This resulted in three different fractions being collected: first, p-tert-butylcalix[4]arene; second, partial-cone 5 (0.38 g, 66%); and third, cone 4 (0.13 g, 22%).
Cone, 4.
Mp = 257 °C. 1H NMR (DMSO-d6, δ, ppm) 7.25 (s, 4H, Ar-H), 6.45 (s, 4H, Ar-H), 5.55 (s, 4H, Ar-O–CH2CON), 4.47 (s, 4H, Ar-O–CH2CN), 4.27 (d, 4H, axial Ar-CH2-Ar, J = 13.0 Hz), 3.40–3.30 (broad m, 4H, N–CH2–CH3), 3.28–3.10 (broad m, 4H, N–CH2–CH3 and 4H, equatorial Ar-CH2-Ar), 1.31 (s, 18H, Ar-CCH3), 1.16–1.04 (m, 12H, N–CH2–CH3), 0.77 (s, 18H, Ar-CCH3). 13C NMR (CDCl3, δ, ppm) 167.2, 152.2, 152.1, 147.0, 144.7, 136.2, 131.9, 126.0, 124.8, 118.5, 77.4, 58.8, 34.5, 33.7, 31.8, 31.1, 14.6, 13.5. IR (ATR): ν/cm−1 2957 (s), 2868 (m), 1600 (w), 1474 (s), 1362 (m), 1188 (s), 1024 (s), 875 (m). Anal. calculated for C60H80O6N4·[C2H6O]: C, 74.51; H, 8.67; N, 5.61. Found C, 74.20; H, 8.42; N, 5.63%.
Partial-cone, 5.
Mp = 256 °C with decomposition. 1H NMR (DMSO-d6, δ, ppm) 7.36 (s, 2H, Ar-H), 7.18 (s, 2H, Ar-H), 6.95 (d, 2H, Ar-H, J = 2.5 Hz), 6.50 (d, 2H, Ar-H, J = 2.5 Hz), 4.69 (s, 2H, Ar-O–CH2CN), 4.63 (d, 2H, Ar-O–CH2CON, J = 13.6 Hz), 4.52 (s, 2H, Ar-O–CH2CN), 4.27 (d, 2H, Ar-O–CH2CON, J = 13.6 Hz), 4.15 (d, 2H, Ar-CH2-Ar, J = 13.3 Hz), 3.94 (d, 2H, Ar-CH2-Ar, J = 13.8 Hz), 3.67 (d, 2H, Ar-CH2-Ar, J = 13.8 Hz), 3.45–3.23 (broad m, 4H, N–CH2–CH3), 3.17 (d, 2H, Ar-CH2-Ar, J = 13.4 Hz), 1.31 (s, 9H, Ar-CCH3), 1.30 (s, 9H, Ar-CCH3), 1.18–1.03 (t, 12H, N–CH2–CH3, J = 7.0 Hz), 1.01 (s, 18H, Ar-CCH3). 13C NMR (CDCl3, δ, ppm) 167.1, 154.4, 153.6, 151.2, 146.8, 144.6, 144.5, 136.2, 132.9, 131.9, 128.7, 126.2, 125.7, 12.54, 118.6, 118.1, 71.3, 56.9, 56.5, 36.8, 34.4, 34.2, 33.9, 31.8, 31.6, 19.0, 14.9, 13.5. IR (ATR): ν/cm−1 2954 (s), 2905 (m), 2870 (m), 1655 (s), 1480 (s), 1361 (m), 1197 (s), 1124 (m), 1029 (m), 870 (w). Anal. calculated for C60H80O6N4·2[C2H6O]: C, 73.53; H, 8.87; N, 5.36. Found C, 73.91; H, 8.43; N, 5.84%.
5,11,17,23-Tetra-tert-butyl-25,26,27-tris(cyanomethoxy)-28-hydroxycalix[4]arene, 6
A barium hydroxide (0.17 g, 0.55 mmol), barium oxide (0.08 g, 0.55 mmol) mixture was added to dry DMF (25 mL) and allowed to stir for 45 minutes at room temperature under a nitrogen atmosphere. To this, calix[4]arene 1 (0.51 g, 0.70 mmol) was allowed to stir for a further 30 minutes. Bromoacetonitrile (0.14 mL, 2.06 mmol) was then added and the resulting beige solution was stirred overnight at room temperature. This resulted in a black, completely opaque solution. Hydrochloric acid (50 mL, 1 M) was slowly added causing a pale brown precipitate to evolve, which was filtered off and washed several times with water. The cream coloured solid is a mixture of both calixarene 2 and 6 with the tris-cyanomethoxy calixarene 6 component calculated from NMR as (0.37 g, 69%) mp = 254 °C. 1H NMR (DMSO-d6, δ, ppm) 7.27 (s, 2H, Ar-H), 7.15 (s, 2H, Ar-H), 6.79 (d, 2H, Ar-H, J = 2.4 Hz), 6.63 (d, 2H, Ar-H, J = 2.4 Hz), 4.96 (d, 4H, Ar-O–CH2, J = 2.2 Hz), 4.83 (s, 2H, Ar-O–CH2), 4.26 (d, 2H, axial Ar-CH2-Ar, J = 13.2 Hz), 4.05 (d, 2H, axial Ar-CH2-Ar, J = 13.3 Hz), 3.45 (d, 2H, equatorial Ar-CH2-Ar, J = 13.5 Hz), 3.35 (d, 2H, equatorial Ar-CH2-Ar, J = 13.2 Hz), 1.28 (s, 9H, Ar-CCH3), 1.27 (s, 9H, Ar-CCH3), 0.89 (s, 18H, Ar-CCH3). 13C NMR (DMSO-d6, δ, ppm) 151.6, 150.7, 150.4, 147.6, 146.6, 135.3, 132.5, 132.3, 128.2, 126.6, 126.0, 125.6, 125.5, 118.4, 117.4, 60.15, 57.9, 34.5, 34.0, 33.9, 32.0, 31.9, 31.7, 31.1. IR (ATR): ν/cm−1 3525 (m), 3135 (w), 2959 (s), 2905 (m), 2868 (m), 1600 (w), 1479 (s), 1362 (m), 1190 (s), 1024 (m), 873 (m).
5,11,17,23-Tetra-tert-butyl-25-diethylcarbamoylmethoxy-26,27,28-tris(cyanomethoxy)calix[4]arene, 7
THF (50 mL) was placed into a round bottom flask containing a mixture of predominantly tris–cyanomethoxy calixarene 6 along with a minor component of tetra-cyanomethoxy 2 (0.53 g, ∼0.69 mmol) and sodium hydride (0.06 g, 2.63 mmol). This mixture was stirred for 30 minutes at room temperature. A solution of N,N-diethylchloroacetamide (0.36 mL, 2.63 mmol) and potassium iodide (0.33 g, 1.97 mmol) in THF was added dropwise to the cloudy solution. The reaction was then heated at reflux for 12 hours. The yellow solution was reduced to dryness leaving a yellow oil which was redissolved in DCM. The organic fraction was washed with hydrochloric acid (1 M, 3 × 50 mL), dried over magnesium sulphate and distilled under reduced pressure. The resulting solid was purified by column chromatography, using a 70/30 petroleum spirit/ethyl acetate mixture. The product eluted as the first spot, which upon characterisation proved to be pure calixarene 7 (0.39 g, 65%) mp = 248 °C with decomposition. 1H NMR (DMSO-d6, δ, ppm) 7.28 (d, 2H, Ar-H, J = 2.5 Hz), 7.26 (d, 2H, Ar-H, J = 2.5 Hz), 6.53 (s, 2H, Ar-H), 6.49 (s, 2H, Ar-H), 5.58 (d, 2H, Ar-O–CH2CN, J = 16.8 Hz), 5.07 (d, 2H, Ar-O–CH2CN, J = 16.8 Hz), 4.91 (s, 2H, Ar-O–CH2CON), 4.47 (s, 2H, Ar-O–CH2CN), 4.39 (d, 2H, Ar-CH2-Ar, J = 12.8 Hz), 4.19 (d, 2H, Ar-CH2-Ar, J = 12.9 Hz), 3.46–3.38 (m, 4H, N–CH2–CH3), 3.34–3.18 (m, 4H, Ar-CH2-Ar), 1.31 (s, 18H, Ar-CCH3), 1.14–1.08 (m, 6H, N–CH2–CH3), 0.80 (s, 9H, Ar-CCH3), 0.79 (s, 9H, Ar-CCH3). 13C NMR (CDCl3, δ, ppm) 167.3, 152.6, 151.9, 150.5, 147.2, 145.8, 144.9, 135.8, 135.7, 132.3, 131.8, 126.4, 126.0, 125.1, 125.0, 118.7, 117.5, 70.5, 60.3, 58.5, 56.5, 34.5, 33.8, 33.7, 31.8, 31.4, 31.1, 19.0, 14.5, 13.5. IR (ATR): ν/cm−1 2961 (s), 2908 (m), 2868 (m), 1648 (m), 1480 (s), 1362 (w), 1196 (s), 1030 (m), 871 (w). Anal. calculated for C56H70O5N4·[C2H6O]: C, 75.29; H, 8.28; N, 6.06. Found C, 75.40; H, 8.67; N, 5.71%.
Crystallography
Crystallographic data for the structures were collected at 100(2) K on an Oxford Diffraction Gemini or Xcalibur diffractometer using Mo Kα radiation. Following multi-scan absorption corrections and solution by direct methods, the structures were refined against F2 with full-matrix least-squares using the program SHELXL-97.9 All hydrogen atoms were added at calculated positions and refined by use of riding models with isotropic displacement parameters based on those of the parent atoms. Anisotropic displacement parameters were employed throughout for the non-hydrogen atoms. Crystallographic data for the structures reported in this paper have been deposited at the Cambridge Crystallographic Data Centre with supplementary publication numbers CCDC 1456439–1456443.†
Refinement details
3: empirical formula C52H60N4O4; formula weight 805.04; crystal system: monoclinic; space group C2/c; unit cell dimensions a = 14.6327(2), b = 16.7338(2), c = 37.5113(4) Å, β = 93.8360(10)°; volume 9164.46(19) Å3; Z = 8; density (calculated) 1.167 Mg m−3; absorption coefficient 0.074 mm−1; crystal size 0.51 × 0.37 × 0.32 mm3; θ range for data collection 3.70 to 38.00°; reflections collected 95
516; independent reflections 24
922 [R(int) = 0.0341]; completeness to θ = 38.00° 99.9%; data/restraints/parameters 24
922/6/569, goodness-of-fit on F2 0.985; final R indices [I > 2σ(I)] R1 = 0.0504, wR2 = 0.1410; R indices (all data) R1 = 0.0774, wR2 = 0.1488. One tertiary butyl group was modelled as being disordered with site occupancies refined to 0.880(2) and its complement. Atoms of the minor component were refined with isotropic displacement parameters and with geometries restrained to ideal values.
4: empirical formula C60H80N4O6; formula weight 953.28; crystal system: monoclinic; space group P21/n; unit cell dimensions a = 21.0623(5), b = 21.8364(3), c = 25.8796(5) Å, β = 109.896(2)°; volume 11
192.2(4) Å3; Z = 8; density (calculated) 1.131 Mg m−3; absorption coefficient 0.072 mm−1; crystal size 0.37 × 0.28 × 0.26 mm3; θ range for data collection 2.81 to 32.00°; reflections collected 145 617; independent reflections 38
570 [R(int) = 0.0439]; completeness to θ = 32.00° 99.2%; data/restraints/parameters 38
570/76/1432, goodness-of-fit on F2 1.102; final R indices [I > 2σ(I)] R1 = 0.0700, wR2 = 0.1463; R indices (all data) R1 = 0.0950, wR2 = 0.1582. Three tertiary butyl groups and one of the amide groups were modelled as being disordered over two sets of sites. Geometries of these groups were restrained to ideal values.
5·EtOH: empirical formula C62H86N4O7; formula weight 999.35; crystal system: triclinic; space group P
; unit cell dimensions a = 11.3151(8), b = 15.0871(7), c = 17.7550(12) Å, α = 86.040(5), β = 80.157(6), γ = 76.643(5)°; volume 2904.2(3) Å3; Z = 2; density (calculated) 1.143 Mg m−3; absorption coefficient 0.074 mm−1; crystal size 0.57 × 0.24 × 0.17 mm3; θ range for data collection 3.54 to 29.00°; reflections collected 29
154; independent reflections 15
430 [R(int) = 0.0626]; completeness to θ = 29.00° 99.8%; data/restraints/parameters 15
430/1/676, goodness-of-fit on F2 1.014; final R indices [I > 2σ(I)] R1 = 0.0638, wR2 = 0.1408; R indices (all data) R1 = 0.1040, wR2 = 0.1626.
6: empirical formula C50H59N3O4; formula weight 766.00; crystal system: triclinic; space group P
; unit cell dimensions a = 11.2306(5), b = 12.9547(5), c = 16.8293(7) Å, α = 78.456(3), β = 71.355(4), γ = 75.486(4)°; volume 2226.64(16) Å3; Z = 2; density (calculated) 1.143 Mg m−3; absorption coefficient 0.072 mm−1; crystal size 0.34 × 0.30 × 0.14 mm3; θ range for data collection 2.81 to 29.00°; reflections collected 20
351; independent reflections 11
503 [R(int) = 0.0300]; completeness to θ = 29.00° 99.4%; data/restraints/parameters 11
503/9/588, goodness-of-fit on F2 1.023; final R indices [I > 2σ(I)] R1 = 0.0641, wR2 = 0.1368; R indices (all data) R1 = 0.0910, wR2 = 0.1496. Two nitrile groups were modelled as being disordered over two sets of sites with site occupancies constrained to 0.5 after trial refinement. One tertiary butyl group was modelled as being slightly disordered with site occupancies refined to 0.903(3) and its complement. Geometries of the minor component were restrained to ideal values.
7·EtOH: empirical formula C58H76N4O6; formula weight 925.23; crystal system: triclinic; space group P
; unit cell dimensions a = 10.6671(5), b = 12.4238(5), c = 21.0581(9) Å, α = 81.960(4), β = 79.731(4), γ = 84.962(4)°; volume 2713.4(2) Å3; Z = 2; density (calculated) 1.132 Mg m−3; absorption coefficient 0.073 mm−1; crystal size 0.39 × 0.27 × 0.06 mm3; θ range for data collection 2.81 to 29.00°; reflections collected 32
902; independent reflections 14
427 [R(int) = 0.0342]; completeness to θ = 29.00° 99.9%; data/restraints/parameters 14
427/21/689, goodness-of-fit on F2 1.088; final R indices [I > 2σ(I)] R1 = 0.0856, wR2 = 0.1905; R indices (all data) R1 = 0.1144, wR2 = 0.2064. The atoms of one tertiary butyl group and those of the solvent ethanol molecule were modelled as being disordered over two sets of sites. The occupancies of the tertiary butyl group were refined to 0.765(5) and its complement with those of the solvent molecule constrained to 0.5 after trial refinement. The geometries of the solvent were restrained to ideal values.
Acknowledgements
We acknowledge use of the facilities and scientific and technical assistance of the Australian Microscopy and Microanalysis Research Facility at the Centre for Microscopy, Characterisation, and Analysis, The University of Western Australia, a facility funded by the University, State and Commonwealth Governments. D. D. gratefully acknowledges The Australian Institute of Nuclear Scientists and Engineers (AINSE) Ltd for provision of a post-graduate research award (AINSE PGRA 2012).
Notes and references
-
C. D. Gutsche, Calixarenes: An Introduction, RSC Publishing, Cambridge, 2nd edn, 2008 Search PubMed.
-
(a) M. Kumar, V. Bhalla, A. Dhir and J. N. Babu, Dalton Trans., 2010, 39, 10116–10121 RSC;
(b) J. Scheerder, M. Fochi, J. F. J. Engbersen and D. N. Reinhoudt, J. Org. Chem., 1994, 59, 7815–7820 CrossRef CAS;
(c) H. Halouani, I. Dumazet-Bonnamour, M. Perrin and R. Lamartine, J. Org. Chem., 2004, 69, 6521–6527 CrossRef CAS PubMed.
-
(a) J. B. Czirok, A. Tarcsay, P. D. Mezei, A. Simon, L. Balazs and I. Bitter, Supramol. Chem., 2014, 26, 722–728 CrossRef CAS;
(b) K. C. Nam, S. O. Kang and Y. J. Ki, Supramol. Chem., 2002, 14, 503–509 CrossRef CAS;
(c) C. Danila, V. Böhmer and M. Bolte, Org. Biomol. Chem., 2005, 3, 3508–3513 RSC.
-
(a) D. D'Alessio, S. Muzzioli, B. W. Skelton, S. Stagni, M. Massi and M. I. Ogden, Dalton Trans., 2012, 41, 4736–4739 RSC;
(b) D. D'Alessio, A. N. Sobolev, B. W. Skelton, R. O. Fuller, R. C. Woodward, N. A. Lengkeek, B. H. Fraser, M. Massi and M. I. Ogden, J. Am. Chem. Soc., 2014, 136, 15122–15125 CrossRef PubMed.
- E. Collins, A. McKervey, S. J. Harris, M. Owens, G. Ferguson and M. Moran, J. Chem. Soc., Perkin Trans. 1, 1991, 3, 3137–3142 RSC.
- C. D. Gutsche, B. Dhawan, J. A. Levine, K. H. No and L. J. Bauer, Tetrahedron, 1983, 39, 409–426 CrossRef CAS.
- D. D'Alessio, B. W. Skelton, N. A. Lengkeek, B. H. Fraser, A. M. Krause-Heuer, S. Muzzioli, S. Stagni, M. Massi and M. I. Ogden, Supramol. Chem., 2015, 27, 787–791 CrossRef.
- G. Dyker, M. Mastalerz, I. M. Muller, K. Merz and K. Koppe, Eur. J. Org. Chem., 2005, 4963–4966 CrossRef CAS.
- G. M. Sheldrick, Acta Crystallogr., Sect. C: Struct. Chem., 2015, 71, 3–8 CrossRef PubMed.
Footnote |
† Electronic supplementary information (ESI) available: Plots of conformer concentrations as a function of time, molecular diagrams, hydrogen bond geometries. CCDC 1456439–1456443. For ESI and crystallographic data in CIF or other electronic format see DOI: 10.1039/c6ra05865e |
|
This journal is © The Royal Society of Chemistry 2016 |
Click here to see how this site uses Cookies. View our privacy policy here.