DOI:
10.1039/C6RA04669J
(Communication)
RSC Adv., 2016,
6, 49123-49126
Base-mediated synthesis of highly functionalized 2-aminonicotinonitriles from α-keto vinyl azides and α,α-dicyanoalkenes†
Received
22nd February 2016
, Accepted 26th April 2016
First published on 27th April 2016
Abstract
A novel access to highly functionalized 2-aminonicotinonitriles via efficient annulations of α-keto vinyl azides and α,α-dicyanoalkenes is described. This annulation was achieved via base-mediated ring-openings and intramolecular rearrangements. A possible mechanism is also proposed.
As the most popular N-heterocycles, pyridine scaffolds play an important role in numerous areas of chemistry, biology and pharmaceuticals.1 Among these, 2-aminonicotinonitrile derivatives attract much more attention over others because of their significant biological activities, such as anticancer,2a anti-inflammatory,2b and anti-prion disease,2c as well as serving as A2A adenosine receptor antagonists,2d IKK-β inhibitors,2e and HIV-1 integrase inhibitors.2f Moreover, 2-aminonicotinonitriles possess a nucleophilic nature from the amino group and electrophilic nature from the cyano group. Because of their unique chemical reactivity, 2-aminonicotinonitriles could be used to construct other complex heterocycles.3 In light of the pyridine scaffold's importance, a great deal of attention has been given to its organic synthesis.4 The classical strategy to afford multifunctionalized 2-aminonicotinonitriles was through the Chichibabin reactions of ketones with an aryl aldehyde, malononitrile, and ammonium5a (Scheme 1-A, eqn (1)) but with poor yields. During the previous decades, numerous methods have been reported,5b–f including using microwave irradiation5b or transition metal catalysts5c,d (Scheme 1-A, eqn (2)). Other methods have also been reported,5g–k such as reacting 2-(1-phenylethylidene)propanedinitriles with dimethyl N-cyanodithioiminocarbonate5g (Scheme 1-A, eqn (3)) or modifying pyridine precursors directly5h,i (Scheme 1-A, eqn (4)). However, the construction of complex 2-aminonicotinonitriles is still challenging. Therefore, an efficient and facile approach for the construction of highly functionalized 2-amino nicotinonitriles is in demand.
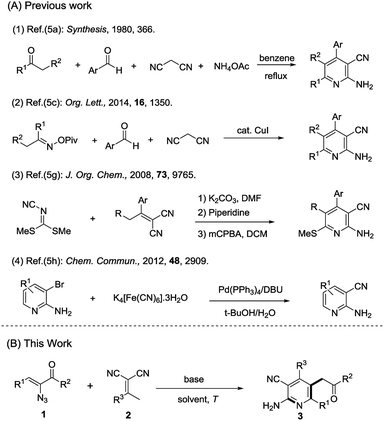 |
| Scheme 1 The synthesis of 2-aminonicotinonitriles. | |
The vinyl azides are reported to be versatile three-atom synthons for the synthesis of diverse N-heterocycles.6,7 α,α-Dicyanoalkenes bear specific functionalities that could be involved in a plurality of cascade reactions with Michael acceptors.8 The continued interest in the reactivity of vinyl azides motivates us to investigate the reaction between α-keto vinyl azides 1 and α,α-dicyanoalkenes 2 (Scheme 1-B).
Initially, we treated (Z)-2-azido-1-(4-chlorophenyl)-3-phenylprop-2-en-1-one 1a (1.0 equiv.) and 2-(1-phenylethylidene)-malononitrile 2a (1.0 equiv.) with Cs2CO3 (0.5 equiv.) in toluene at 90 °C for 3 h. This reaction interestingly led to the formation of the highly functionalized 2-aminonicotinonitrile 3a, which was confirmed by HRMS, 1H NMR, and 13C NMR, as well as X-ray crystal structure analysis (Fig. 1), in an acceptable yield (Table 1, entry 1).
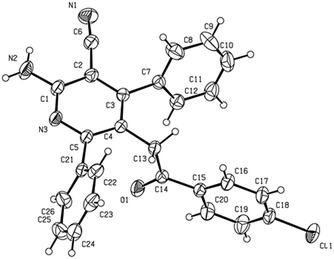 |
| Fig. 1 X-ray crystal structure of 3a.9 | |
Table 1 Optimization of the reaction conditionsa
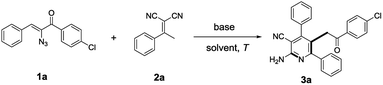
|
Entry |
Solvent |
Base (equiv.) |
T/°C |
Conversionb/% |
Reaction conditions: 1a (0.1 mmol, 1.0 equiv.) and 2a (0.1 mmol, 1.0 equiv.) in various solvents (3 mL, well-sealed tubes) at selected temperature in the presence of the base for 3 h. The conversion rate was determined by HPLC, based on the disappearance of the starting α-keto vinyl azides 1a. Isolated yield. n.r. = no reaction. |
1 |
Toluene |
Cs2CO3 (0.5) |
90 |
44 (40)c |
2 |
DCM |
Cs2CO3 (0.5) |
90 |
50 |
3 |
THF |
Cs2CO3 (0.5) |
90 |
31 |
4 |
EtOH |
Cs2CO3 (0.5) |
90 |
Trace |
5 |
DMF |
Cs2CO3 (0.5) |
90 |
Trace |
6 |
ACN |
Cs2CO3 (0.5) |
90 |
Trace |
7 |
DCE |
Cs2CO3 (0.5) |
90 |
56 |
8 |
1,4-Dioxane |
Cs2CO3 (0.5) |
90 |
24 |
9 |
DCE |
Et3N (0.5) |
90 |
n.r. |
10 |
DCE |
NaOH (0.5) |
90 |
19 |
11 |
DCE |
K2CO3 (0.5) |
90 |
20 |
12 |
DCE |
MeONa (0.5) |
90 |
61 (59)c |
13 |
DCE |
MeONa (0.5) |
rt |
n.r. |
14 |
DCE |
MeONa (0.5) |
60 |
n.r. |
15 |
DCE |
MeONa (0.5) |
120 |
83 (80)c |
16 |
DCE |
— |
120 |
n.r. |
17 |
DCE |
MeONa (0.1) |
120 |
17 |
18 |
DCE |
MeONa (1.0) |
120 |
25 |
19 |
DCE |
MeONa (2.0) |
120 |
26 |
Further studies found that the reaction solvent and base have a significant impact on the formation of 3a. When the reaction was performed in DCM or DCE, the conversion of 3a was slightly improved to 50% and 56%, respectively (Table 1, entry 2 and 7). Moreover, only a trace amount of 3a could be detected via LC-MS when performed in EtOH, DMF or ACN (Table 1, entry 4–6). The screening of bases showed that MeONa worked more efficiently, thus giving 3a in 59% yield (Table 1, entry 7 and entries 9–12). Optimization of temperature demonstrated that room temperature or 60 °C failed to obtain the desired product, whereas increasing to 120 °C could lead to an increased yield of 3a of 80% (Table 1, entries 12–15). Subsequent screening of the equivalents of MeONa showed that 0.5 equivalent was the best (Table 1, entries 15–19). As shown in Table 1, the optimal reaction condition was obtained in DCE with well-sealed tubes at 120 °C when 0.5 equivalent of MeONa was employed (Table 1, entry 15).
With the optimized reaction conditions in hand, the generality for the synthesis of highly functionalized 2-aminonicotinonitriles was explored. As shown in Table 2, α-keto vinyl azides 1 worked efficiently with α,α-dicyanoalkenes 2 to provide the desired products in moderate to good yields (3b–3i, 74–82%), regardless of whether an electron-withdrawing group (–Cl, –Br) or electron-donating group (–OMe) was borne at the R1 position on the aromatic ring or just an alkyl group. Moreover, the steric hindrance has a slightly effect on the reaction yields (3d–3f). Furthermore, most of the substituents R2 were found to be compatible in this reaction, including both the aromatic and heteroaromatic rings (3a, 3j–3o) and alkyl group (3y), with the exception being the hydrogen atom (3p). We failed to obtain the product 3p even after heating for 24 hours. In addition to these, the scope of various α,α-dicyanoalkenes 2 were also examined in this synthesis. Likewise, the installation of electron-deficient (3b, 3j–3k, 3o, 3w) and -rich benzene rings (3q, 3s–3t, 3x) or heteroaromatic rings (3r) as the substituent R3 were all well tolerated under the optimized reaction conditions. When R3 was replaced with alkyl groups (t-Bu- or Me-), the desired products were only detected via LC-MS in trace under the above-stated reaction condition. However, much higher isolated yields could be achieved when the reaction was performed for a longer period of time along with 1.5 equivalents of MeONa (3u and 3v), and the structure of 3v was further confirmed by 1H NMR, 13C NMR, HRMS and 13C–1H HMBC spectra (in ESI†).
Table 2 Scope of 2-aminonicotinonitrile from vinyl azides 1 and α,α-dicyanoalkenes 2a

|
Reaction conditions: α-keto vinyl azides 1 (1.0 mmol, 1.0 equiv.), α,α-dicyanoalkenes 2 (1.0 mmol, 1.0 equiv.), and MeONa (0.5 mmol, 0.5 equiv.) in DCE, heated in a well-sealed tube (120 °C). Yields shown are those of the isolated products. 1.5 equivalents of MeONa were used. |
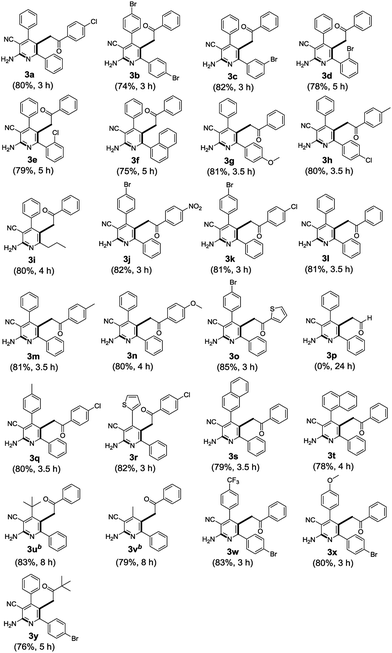 |
On the basis of the above mentioned investigation, a possible mechanism for this annulation is proposed in Scheme 2. First, the α-keto vinyl azide 1 could undergo thermolysis to give 2H-azirine A under the stated reaction conditions.6i In the presence of base, the α,α-dicyanoalkene 2 is converted to the vinylogous carbanion B via a facile deprotonation.8h Then, a nucleophilic attack would occur between B and 2H-azirine A, leading to the adduct product C, which then turned into D through an intramolecular nucleophilic attack between the nitrogen of the aziridine and a cyano group. A possible 4-endo-tet cyclization10 affording the adduct F may occur after deprotonation of D at the methylene. Then, F undergoes a facile ring opening of the strained four-membered ring to obtain G via a C–N bond cleavage. Finally, the desired product 3 is achieved through aromatization of G.
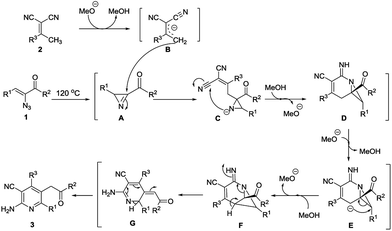 |
| Scheme 2 The possible reaction mechanism. | |
In summary, we have developed an efficient strategy for preparing highly functionalized 2-aminonicotinonitriles from readily available α-keto vinyl azides and α,α-dicyanoalkenes. These base-mediated annulations can be realized via intramolecular rearrangement and ring openings in good yields. This methodology also provides an attractive guidance for medicinal chemistry and pharmaceutical applications.
Acknowledgements
This study was supported by the National Natural Science Foundation of China (No. 81273356 and 81473074), National Science & Technology Major Projects for “Major New Drugs Innovation and Development” of China (2014ZX09304002-007), the Program for Zhejiang Leading Team of S&T Innovation Team (2011R50014), and Arthritis & Chronic Pain Research Institute, USA to Y. Y.; and National Natural Science Foundation of China (No. 81402778) to W. C.
Notes and references
-
(a) F. Gutiérrez-Nicolás, J. C. Oberti, Á. G. Ravelo and A. Estévez-Braun, J. Nat. Prod., 2014, 77, 1853 CrossRef PubMed;
(b) J. P. Machael, Nat. Prod. Rep., 2005, 22, 627 RSC;
(c) S. Zheng, Q. Zhong, M. Mottamal, Q. Zhang, C. Zhang, E. LeMelle, H. McFerrin and G. Wang, J. Med. Chem., 2014, 57, 3369 CrossRef CAS PubMed;
(d) F. Durola, J. P. Sauvage and O. S. Wenger, Chem. Commun., 2006, 171 RSC;
(e) T. Ishizuka, M. Kimoto, A. Sato and I. Hirao, Chem. Commun., 2012, 48, 10835 RSC;
(f) X.-Z. Luo, X.-J. Jia, J.-H. Deng, J.-L. Zhong, H.-J. Liu, K.-J. Wang and D.-C. Zhong, J. Am. Chem. Soc., 2013, 135, 11684 CrossRef CAS PubMed;
(g) C. W. Lee and J. Y. Lee, Chem. Commun., 2013, 49, 6185 RSC;
(h) T. Kobayashi, S. Sasaki, N. Tomita, S. Fukui, M. Nakayama, A. Kiba, M. Kusaka, S. I. Matsumoto, M. Yamaguchi, F. Itoh and A. Baba, Bioorg. Med. Chem., 2010, 18, 5157 CrossRef CAS PubMed;
(i) B. C. H. May, J. A. Zorn, J. Witkop, J. Sherrill, A. C. Wallace, G. Legname, S. B. Prudiner and F. E. Cohen, J. Med. Chem., 2007, 50, 65 CrossRef CAS PubMed.
-
(a) R. E. Khidre, A. A. Abu-Hashem and M. El-Shazly, Eur. J. Med. Chem., 2011, 46, 5057 CrossRef CAS PubMed;
(b) F. Zhang, Y. Zhao, L. Sun, L. Ding, Y. Gu and P. Gong, Eur. J. Med. Chem., 2011, 46, 3149 CrossRef CAS PubMed;
(c) N. Kumar, A. Chauhan and S. Drabu, Biomed. Pharmacother., 2011, 653, 75 Search PubMed;
(d) T. R. K. Reddy, R. Mutter, W. Heal, K. Guo, V. J. Gillet, S. Pratt and B. Chen, J. Med. Chem., 2006, 49, 607 CrossRef CAS PubMed;
(e) M. Mantri, O. de Graaf, J. van Veldhoven, A. Göblyös, J. K. von Frijtag Drabbe Künzel, T. Mulder-Krieger, R. Link, H. de Vries, M. W. Beukers, J. Brussee and A. P. Ijzerman, J. Med. Chem., 2008, 51, 4449 CrossRef CAS PubMed;
(f) T. Murata, M. Shimada, S. Sakakibara, T. Yoshino, H. Kadono, T. Masuda, M. Shimazaki, T. Shintani, K. Fuchikami, K. Sakai, H. Inbe, K. Takeshita, T. Niki, M. Umeda, K. B. Bacon, K. B. Ziegelbauer and T. B. Lowinger, Bioorg. Med. Chem. Lett., 2003, 13, 913 CrossRef CAS PubMed;
(g) J. Deng, T. Sanchez, L. Q. Al-Mawsawi, R. Dayam, R. A. Yunes, A. Garofalo, M. B. Bolger and N. Neamati, Bioorg. Med. Chem., 2007, 15, 4985 CrossRef CAS PubMed.
-
(a) L. Yang, D. Shi, S. Chen, H. Chai, D. Huang, Q. Zhang and J. Li, Green Chem., 2012, 14, 945 RSC;
(b) D. Sucunza, A. Samadi, M. Chioua, D. B. Silva, C. Yunta, L. Infantes, M. C. Carreiras, E. Sorianoa and J. Marco-Contelles, Chem. Commun., 2011, 47, 5043 RSC;
(c) A. M. Soliman, J. Heterocycl. Chem., 2011, 48, 592 CrossRef CAS;
(d) C. M. Lacbay, J. Mancuso, Y.-S. Lin, N. Bennett, M. Götte and Y. S. Tsantrizos, J. Med. Chem., 2014, 57, 7435 CrossRef CAS PubMed;
(e) B. Orlikova, W. Chaouni, M. Schumacher, M. Aadil, M. Diederich and G. Kirsch, Eur. J. Med. Chem., 2014, 85, 450 CrossRef CAS PubMed;
(f) M. A. Al-Haiza, M. S. Mostafa and M. Y. El-Kady, Molecules, 2003, 8, 275 CrossRef CAS.
- For reviews of pyridine synthesis:
(a) J. A. Bull, J. J. Mousseau, G. Pelletier and A. B. Charette, Chem. Rev., 2012, 112, 2642 CrossRef CAS PubMed;
(b) B. Heller and M. Hapke, Chem. Soc. Rev., 2007, 36, 1085 RSC;
(c) C. Allais, J.-M. Grassot, J. Rodriguez and T. Constantieux, Chem. Rev., 2014, 114, 10829 CrossRef CAS PubMed. For recent examples of pyridine synthesis:
(d) G. Cheng, Y. Weng, X. Yang and X. Cui, Org. Lett., 2015, 17, 3790 CrossRef CAS PubMed;
(e) Y. Jiang, C.-M. Park and T.-P. Loh, Org. Lett., 2014, 16, 3432 CrossRef CAS PubMed;
(f) X. He, Y. Shang, Z. Yu, M. Fang, Y. Zhou, G. Han and F. Wu, J. Org. Chem., 2014, 79, 8882 CrossRef CAS PubMed;
(g) J. M. Neely and T. Rovis, J. Am. Chem. Soc., 2014, 136, 2735 CrossRef CAS PubMed;
(h) M. Yoshida, T. Mizuguchi and K. Namba, Angew. Chem., Int. Ed., 2014, 53, 14550 CrossRef CAS PubMed;
(i) Y. Wei and N. Yoshikai, J. Am. Chem. Soc., 2013, 135, 3756 CrossRef CAS PubMed;
(j) M.-N. Zhao, Z.-H. Ren, Y.-Y. Wang and Z.-H. Guan, Chem. Commun., 2012, 48, 8105 RSC.
-
(a) S. Kambe, K. Saito, A. Sakurai and H. Midorikawa, Synthesis, 1980, 366 CrossRef CAS;
(b) F. Shi, S. J. Tu, F. Fang and T. J. Li, ARKIVOC, 2005, 137 CAS;
(c) Q. Wu, Y. Zhang and S. Cui, Org. Lett., 2014, 16, 1350 CrossRef CAS PubMed;
(d) J. Tang, L. Wang, Y. Yao, L. Zhang and W. Wang, Tetrahedron Lett., 2011, 52, 509 CrossRef CAS;
(e) F. Jemmezi, S. Chtiba and J. Khiari, J. Heterocycl. Chem., 2013, 50, 206 CrossRef CAS;
(f) T. Shintani, H. Kadono, T. Kikuchi, T. Schubert, Y. Shogase and M. Shimazaki, Tetrahedron Lett., 2003, 44, 6567 CrossRef CAS. For other examples of 2-aminonicotinonitrile derivatives synthesis:
(g) S. J. Teague, J. Org. Chem., 2008, 73, 9765 CrossRef CAS PubMed;
(h) D. Zhang, H. Sun, L. Zhang, Y. Zhou, C. Li, H. Jiang, K. Chen and H. Liu, Chem. Commun., 2012, 48, 2909 RSC;
(i) S. Guo, Y. Wang, C. Sun, J. Li, D. Zou, Y. Wu and Y. Wu, Tetrahedron Lett., 2013, 54, 3233 CrossRef CAS;
(j) P. Manna and P. K. Maiti, Tetrahedron Lett., 2015, 56, 5094 CrossRef CAS;
(k) B. Jiang, X. Wang, F. Shi, S.-J. Tu and G. Li, Org. Biomol. Chem., 2011, 9, 4025 RSC;
(l) Farhanullah, N. Agarwal, A. Goel and V. J. Ram, J. Org. Chem., 2003, 68, 2983 CrossRef CAS PubMed.
- The review of the reactivities of vinyl azides:
(a) B. Hu and S. G. DiMagno, Org. Biomol. Chem., 2015, 13, 3844 RSC. Selected reactions with vinyl azides:
(b) L. Xiang, Y. Niu, X. Pang and R. Yan, Chem. Commun., 2015, 51, 6598 RSC;
(c) P. R. Adiyala, G. S. Mani, J. B. Nanubolu, K. C. Shekar and R. A. Maurya, Org. Lett., 2015, 17, 4308 CrossRef CAS PubMed;
(d) Y.-F. Wang, G. H. Lonca and S. Chiba, Angew. Chem., Int. Ed., 2014, 53, 1067 CrossRef CAS PubMed;
(e) R. R. Donthiri, V. Pappula, N. N. Reddy, D. Bairagi and S. Adimurthy, J. Org. Chem., 2014, 79, 11277 CrossRef CAS PubMed;
(f) N. Jung and S. Bräse, Angew. Chem., Int. Ed., 2012, 51, 12169 CrossRef CAS PubMed;
(g) K. V. Sajna and K. C. Kumara Swamy, J. Org. Chem., 2012, 77, 8712 CrossRef CAS PubMed;
(h) Y. F. Wang, K. K. Toh, E. P. J. Ng and S. Chiba, J. Am. Chem. Soc., 2011, 133, 6411 CrossRef CAS PubMed;
(i) S. Chiba, Y.-F. Wang, G. Lapointe and K. Narasaka, Org. Lett., 2008, 10, 313 CrossRef CAS PubMed.
-
(a) J. Shao, X. Liu, K. Shu, P. Tang, J. Luo, W. Chen and Y. Yu, Org. Lett., 2015, 17, 4502 CrossRef CAS PubMed;
(b) B. Chen, S. Guo, X. Guo, G. Zhang and Y. Yu, Org. Lett., 2015, 17, 4698 CrossRef CAS PubMed;
(c) G. Zhang, B. Chen, X. Guo, S. Guo and Y. Yu, Adv. Synth. Catal., 2015, 357, 1065 CrossRef CAS;
(d) S. Liu, W. Chen, J. Luo and Y. Yu, Chem. Commun., 2014, 50, 8539 RSC;
(e) G. Zhang, H. Ni, W. Chen, J. Shao, H. Liu, B. Chen and Y. Yu, Org. Lett., 2013, 15, 5967 CrossRef CAS PubMed;
(f) J. Shao, W. Yu, Z. Shao and Y. Yu, Chem. Commun., 2012, 48, 2785 RSC;
(g) W. Chen, M. Hu, J. Wu, H. Zou and Y. Yu, Org. Lett., 2010, 12, 3863 CrossRef CAS PubMed.
- Selected examples involving α,α-dicyanoalkenes:
(a) Y. Xu, J. Han, Y. Lv, R. Pan, J. Chen, H. Deng, M. Shao, H. Zhang and W. Cao, Tetrahedron, 2015, 71, 820 CrossRef CAS;
(b) S. L. Broman, M. Jevric, A. D. Bond and M. B. Nielsen, J. Org. Chem., 2014, 79, 41 CrossRef CAS PubMed;
(c) E. Gopi and I. N. N. Namboothir, J. Org. Chem., 2014, 79, 7468 CrossRef CAS PubMed;
(d) X. Wang, G. Liu, X.-H. Xu, N. Shibata, E. Tokunaga and N. Shibata, Angew. Chem., Int. Ed., 2014, 53, 1827 CrossRef PubMed;
(e) S. Rout, S. K. Ray, R. A. Unhale and V. K. Singh, Org. Lett., 2014, 16, 5568 CrossRef CAS PubMed;
(f) A. R. Longstreet, B. S. Campbell, B. F. Gupton and D. T. McQuade, Org. Lett., 2013, 15, 5298 CrossRef CAS PubMed;
(g) P. Das, S. Ray and C. Mukhopadhyay, Org. Lett., 2013, 15, 5622 CrossRef CAS PubMed;
(h) O. A. Attanasi, G. Favi, A. Geronikaki, F. Mantellini, G. Moscatelli and A. Paparisva, Org. Lett., 2013, 15, 2624 CrossRef CAS PubMed;
(i) C. Ma, Z.-J. Jia, J.-X. Liu, Q.-Q. Zhou, L. Dong and Y.-C. Chen, Angew. Chem., Int. Ed., 2013, 52, 948 CrossRef CAS PubMed;
(j) Z. Lu, S. Zheng, X. Zhang and X. Lu, Org. Lett., 2008, 10, 3267 CrossRef CAS PubMed;
(k) D. Xue, J. Li, Z.-T. Zhang and J.-G. Deng, J. Org. Chem., 2007, 72, 5443 CrossRef CAS PubMed;
(l) J.-W. Xie, W. Chen, R. Li, M. Zeng, W. Du, L. Yue, Y.-C. Chen, Y. Wu, J. Zhu and J.-G. Deng, Angew. Chem., Int. Ed., 2007, 46, 389 CrossRef CAS PubMed.
- ESI.†.
- This possible mechanism contains an unfavorable 4-endo-tet cyclization and further investigation on the reaction mechanism is underway.
Footnote |
† Electronic supplementary information (ESI) available: Experimental procedures, characterization, spectral data of the final products, 13C–1H HMBC spectra of 3v and crystallographic data for compound 3a. CCDC 1431902. For ESI and crystallographic data in CIF or other electronic format see DOI: 10.1039/c6ra04669j |
|
This journal is © The Royal Society of Chemistry 2016 |