DOI:
10.1039/C6RA03188A
(Paper)
RSC Adv., 2016,
6, 27584-27589
Open-air oxidative Mizoroki–Heck reaction of arylsulfonyl hydrazides with alkenes†
Received
3rd February 2016
, Accepted 6th March 2016
First published on 15th March 2016
Abstract
A palladium(II)-catalyzed oxidative Mizoroki–Heck reaction of arylsulfonyl hydrazides with alkenes was developed employing atmospheric air as the sole oxidant in an open-vessel manner. By using palladium(II) acetate associating with inexpensive, air-stable and moisture stable pyridine ligand L9 as the catalyst system, the efficiency of the reaction could be significantly enhanced. A wide range of arylsulfonyl hydrazides underwent the oxidative Mizoroki–Heck reaction with alkenes smoothly. Good-to-excellent product yields and excellent regio- and stereoselectivity were achieved. Functional groups such as halo, ester etc. were well-tolerated under these optimized reaction conditions.
Introduction
The palladium-catalyzed Mizoroki–Heck reaction is one of the most powerful tools for incorporating a C–C double bond in organic synthesis1 and plays an important role in both synthetic laboratories and industrial applications.2 In the traditional Heck reaction, aryl halides act as electrophiles and couple with alkenes in the presence of palladium catalysts under basic conditions to produce higher substituted alkenes.3 Apart from aryl halides, aryl sulfonates,4 aryl diazonium salts,5 arylsulfonyl halides,6 and arylcarboxylic acid derivatives7 are also effective aryl sources in the Mizoroki–Heck type reactions.
The oxidative Heck reaction is also an excellent pathway to access substituted alkene products.8 The most striking difference between traditional Heck reaction and oxidative Heck reaction is the reaction mechanism. The former is that electrophiles act as self-oxidant for carrying out oxidative addition to palladium(0) species to form arylpalladium(II) intermediates. The latter is that extra oxidants such as oxygen or copper(II) salts are required to re-oxidize palladium(0) species to palladium(II) complexes. Aromatics,9 arylcarboxylic acids,10 arylboronic acid derivatives,11 arylphosphonic acids,12 arylsulfonic acids13 and its sodium salts,14 arylhydrazines,15 carbazates16 and aroyl hydrazides17 were shown to effectively couple with alkenes in the oxidative reactions (Scheme 1).
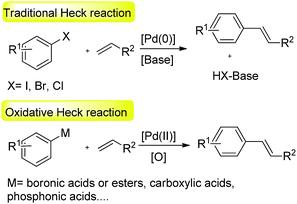 |
| Scheme 1 Traditional Heck reaction of aryl halides and oxidative Heck reaction using other aryl sources with alkenes. | |
Arylsulfonyl hydrazides are recently rising electrophiles which are readily accessible solids, stable in air and moisture conditions and can be easily prepared in one step from arylsulfonyl chlorides and hydrazine hydrates.18 Particular noteworthy is that they can serve as arylating agents, which are subjected to denitrogenation and desulfitation to generate the aryl source for the oxidative palladium-catalyzed coupling reactions.19 Oxidative Heck reaction,19a oxidative direct arylation of heteroarenes,19b-d oxidative conjugate addition reaction,19e oxidative homo-coupling reaction,19f oxidative Suzuki reaction19g and oxidative Hiyama reaction19h have been reported.
Currently, most of these reactions are using excess amount of Cu(OAc)2 or pure oxygen as oxidant. We envision that the use of the atmospheric air in open-vessel manner is one of the most attractive protocols for the oxidative Heck reaction because of the following advantages: (1) avoid the handling of dangerous and hazardous pressurized gas tube, (2) allow to release the extra gaseous pressure generates from denitrogenation (N2) and desulfitation (SO2) of arylsulfonyl hydrazides under high temperature, (3) can provide a constant oxygen concentration over the whole reaction period which may maximize the utility of this technology for genuine applications. However, in general, only inferior yields were obtained when air is used as the reaction atmosphere especially for the Heck-type reactions.19a,e Herein, we attempted to advance this oxidative Heck reaction by using atmospheric air in open air manner.
Results and discussion
In order to test the feasibility of palladium-catalyzed oxidative Mizoroki–Heck reaction of arylsulfonyl hydrazides with alkenes, a series of screenings was conducted (Table 1). 4-Methylbenzenesulfonyl hydrazide and styrene were used as the benchmark substrates. In surveying of common solvents, DMF gave the best result (Table 1, entry 2 and entries 1–11). The initial screening of palladium sources (Table 1, entries 12–18) indicated that Pd(OAc)2 was the suitable Pd source for this coupling reaction.
Table 1 Initial screening of palladium-catalyzed oxidative Mizoroki–Heck-type reaction of arylsulfonyl hydrazidesa
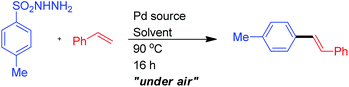
|
Entry |
Catalyst (mol%) |
Solvent |
% yieldb |
Reaction condition: 4-methylbenzenesulfonyl hydrazide (0.45 mmol), styrene (0.3 mmol), Pd source (10 mol%) and solvent (3.0 mL) were stirred at 90 °C for 16 h under air.
Calibrated GC yields were reported using dodecane as internal standard.
|
1 |
Pd(OAc)2 (10) |
Dioxane |
40 |
2 |
Pd(OAc)2 (10) |
DMF |
55 |
3 |
Pd(OAc)2 (10) |
DMA |
47 |
4 |
Pd(OAc)2 (10) |
NMP |
40 |
5 |
Pd(OAc)2 (10) |
DMSO |
14 |
6 |
Pd(OAc)2 (10) |
THF |
7 |
7 |
Pd(OAc)2 (10) |
t-BuOH |
20 |
8 |
Pd(OAc)2 (10) |
Toluene |
5 |
9 |
Pd(OAc)2 (10) |
MeCN |
13 |
10 |
Pd(OAc)2 (10) |
PhCN |
26 |
11 |
Pd(OAc)2 (10) |
AcOH |
17 |
12 |
Pd2(dba)3 (10) |
DMF |
15 |
13 |
Pd(COOCF3)2 (10) |
DMF |
41 |
14 |
Pd(acac)2 (10) |
DMF |
23 |
15 |
PdCl2(CH3CN)2 (10) |
DMF |
51 |
16 |
PdCl2(PPh3)2 (10) |
DMF |
32 |
17 |
PdCl2(COD)2 (10) |
DMF |
Trace |
18 |
PdCl2 (10) |
DMF |
40 |
After the detailed optimization of solvents and Pd sources, we still found that the product yield was unsatisfactory. Inspired by the poor result of Pd(OAc)2/DMSO system which is one of the well-known catalyst systems for the palladium-catalyzed oxidative reactions and also analogy to the aerobic alcohol oxidation reaction and oxidative amination of olefins reaction,20 we were intrigued that the inferior yield may result from the slow aerobic oxidation of Pd(0) species to Pd(II), especially under air atmosphere which is low oxygen concentration. Pd(0) species easily aggregate and agglomerate to unreactive palladium black and retard the coupling catalysis in the ligand-free Heck reactions. Oxidatively stable ligands have been identified to enhance the rate of Pd(0) oxidation particularly with respect to competing Pd(0) aggregation so as to promote catalyst turnover, improve catalyst stability and increases regio- and stereoselectivity of the reaction.20
Uemura's Pd(OAc)2/pyridine catalyst system were found to promote the oxidation of Pd(0) to Pd(II) in the aerobic alcohol oxidation reaction.20 Herein, we adopt the Pd(OAc)2/pyridine catalyst system for the oxidative Heck reaction of arylsulfonyl hydrazides with alkenes for the first time.
We found that when pyridine was added, the product yield improved satisfactorily from 55% to 77% (Table 2, L1). When the reaction temperature was lowered from 90 °C to 80 °C, the product yield remained the unaffected. An evaluation of different substituted pyridine ligands L2–L11 indicated that phenyl isonicotinate (L9) gave the best yield of product. Although the electronic influence of the pyridine-type ligands in this oxidative heck reaction is still unclear, sterically hindered pyridine type ligands L10–L11 which hinder the facial coordination to Pd center show minor enhancing effect of the product yield that imply the importance of the ligand coordination effect.
Table 2 Ligand screening for palladium-catalyzed oxidative Mizoroki–Heck-type reaction of arylsulfonyl hydrazidesa,b
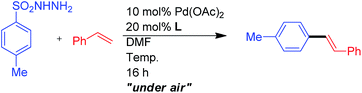
|
Reaction conditions: 4-methylbenzenesulfonyl hydrazide (0.45 mmol), styrene (0.3 mmol), Pd(OAc)2 (10 mol%), L (20 mol%), DMF (3.0 mL) were stirred at 80 °C for 16 h under air.
Calibrated GC yields were reported using dodecane as internal standard.
The reaction was conducted at 90 °C.
|
|
With the Pd(OAc)2/L9 system in hand, we next investigated the scope of Mizoroki–Heck reaction of arylsulfonyl hydrazides with alkenes. The results were summarized in Table 3. A wide range of arylsulfonylhydrazides carried out oxidative Mizoroki–Heck reaction with styrene smoothly to give 1,2-disubstituted alkenes in moderate-to-excellent yields under air atmosphere. ortho-Substituted arylsulfonyl hydrazides were good coupling partners for this reaction (Table 3, compounds 3bk and 3gk). Electron-rich arylsulfonyl hydrazide afforded moderate yield (Table 3, compound 3hk). The highly sterically hindered arylsulfonyl hydrazide coupled well with styrene (Table 3, compound 3ik). Apart from styrene, a variety of substituted styrenes and acrylates was examined and provided good to excellent product yields.21 To our delight, the highly sterically hindered arylsulfonyl hydrazide coupled with butyl acrylate in good yield (Table 3, compound 3in). Both of electron-deficient and electron-rich substituents on styrenes were feasible cross-coupling partners (Table 3, compounds 3ao, 3ap, 3aq, 3as, 3at and 3au). The highly sterically crowded 2,4,6-trimethylstyrenes proceeded well with 4-chlorobenzenesulfonyl hydrazide partner smoothly (Table 3, compound 3fv). Notably, bromo and chloro groups were compatible under these optimized reaction conditions that are useful moieties for further chemical transformations using other coupling technology (Table 3, compounds 3fk, 3aq, 3au and 3fv).
Table 3 Palladium-catalyzed oxidative Mizoroki–Heck-type reaction of arylsulfonyl hydrazides and olefinsa,b
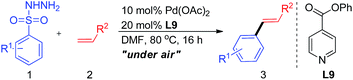
|
Reaction conditions: arylsulfonyl hydrazide (0.45 mmol), alkene (0.3 mmol), Pd(OAc)2 (10 mol%), L9 (20 mol%) and DMF (3 mL) were stirred at 80 °C for 16 h under air.
Isolated yield.
The reaction was conducted at 70 °C.
The reaction was conducted at 100 °C.
Close system was used.
The reaction was conducted for 24 h.
|
|
In order to have a better understanding of the effect of pyridine ligand towards the reaction rate of palladium-catalyzed oxidative Mizoroki–Heck reaction, 4-methylbenzenesulfonyl hydrazide and styrene were selected as substrates for the kinetic studies (Scheme 2). Two sets of parallel experiment in the presence of L9 and absence of L9 were conducted which was represented by
blue line and
red line in Scheme 2 respectively. The reaction rates in both cases were essentially the same within the first 30 min, which indicated the reaction rate was not obviously promoted by the presence of L9. At 45 min, on the wall of the reaction tube, a small amount of palladium black was observed in the absence of L9 while palladium black was not observed in the presence of L9. In the absence of the L9, the reaction rate decreased more significantly at 90 min and the reaction rate became steady after 180 min. In contrast, in the presence of L9, the reaction rate decreased relatively slower in the first 120 min and the reaction rate became steady after 240 min. Furthermore, in the presence of the L9, a higher turnover number could be obtained within the same reaction period. This kinetic result implied that the coordination of the pyridine ligand to the Pd center may reduce the possibility of the formation of inactive Pd black so that the reaction can be promoted by a higher concentration of the active Pd species during the course of the reaction.
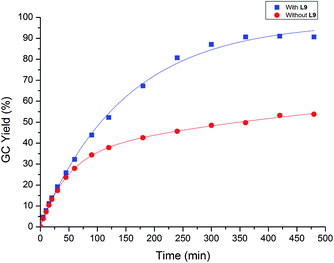 |
| Scheme 2 Kinetic study of the effect of the pyridine ligand L9 on the oxidative Mizoroki–Heck-type reaction of 4-methylbenzenesulfonyl hydrazide with styrene. | |
A proposed catalytic cycle, as adapted from the mechanistic studies performed on the Pd-catalyzed coupling reactions of arylsulfonyl hydrazides and Pd(OAc)2/pyridine oxidation reaction mechanism, is shown in Scheme 3.19,20 The coordination of the pyridine ligand to Pd(OAc)2 generate complex I which is subsequently converted to complex II and HOAc by deprotonation with arylsulfonyl hydrazide. Complex II undergoes β-hydride elimination to give complex III and sulfonyl diazene. Displacement of complex I with sulfonyl diazene gives complex IV. Liberation of N2 with complex IV provides the complex V, and successive extrusion of SO2 affords complex VI. The coordination of alkene to complex VI followed by alkene insertion generates complex VII. Complex VII undergoes β-hydride elimination to generate complex III. Reductive elimination of complex III generates complex VIII, and the complex I is regenerated by oxidation.
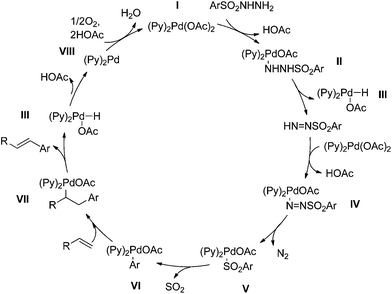 |
| Scheme 3 Proposed catalytic cycle. | |
Conclusions
In summary, we have reported the first examples of oxidative Mizoroki–Heck reaction of arylsulfonyl hydrazides with alkenes in an open-air manner using the stable and inexpensive pyridine ligand L9. The Pd(OAc)2/L9 catalyst system was effective for the coupling of a wide range of arylsulfonyl hydrazides and alkenes. It was noteworthy that the reaction was conducted under atmospheric air. The preliminary kinetic study result showed that pyridine ligand L9 may reduce the Pd(0) aggregation so as to promote catalyst turnover. We expect this convenient synthetic method will be useful in the organic synthesis.
Experimental section
General procedure for the oxidative heck reaction of arylsulfonyl hydrazides with alkenes
All reagents were weighted in air and the reactions were performed in an open vessel. Pd(OAc)2 (0.0068 g, 0.03 mmol) and phenyl isonicotinate (Pd
:
L = 1
:
2) were loaded into a 40 mL vial equipped with a Teflon-coated magnetic stir bar. Precomplexation was applied by adding DMF (1 mL) in to the vial. The palladium complex stock solution was stirred for 10 minutes. Arylsulfonyl hydrazide (0.45 mmol) and alkenes (0.3 mmol) were loaded into the vial. DMF (2 mL) was added with continuous stirring at room temperature. The vial was fitted with an air condenser as cooler and then placed into a preheated oil bath which the temperature was indicated in the table and vigorously stirred for 16 h. After the completion of reaction, the reaction vial was allowed to cool at room temperature. Ethyl acetate (∼10 mL) was added. The organic layer was subjected to GC analysis. After analyzing GC spectra, the crude product in the organic layer was extracted and the vial washed with ethyl acetate. The filtrate was concentrated under reduced pressure. The crude product was purified by flash column chromatography on silica gel (230–400 mesh) to afford the desired product.
Acknowledgements
We thank the Research Grants Council of Hong Kong, (CRF: C5023-14G), and General Research Fund (GRF: PolyU 153008/14P) and State Key Laboratory of Chirosciences for financial support. Grateful to Prof. Albert S. C. Chan's research group (PolyU Hong Kong) for sharing of GC-FID and GC-MS instruments.
Notes and references
-
(a) R. F. Heck, Acc. Chem. Res., 1979, 12, 146 CrossRef CAS
;
(b) G. D. Daves Jr and A. Hallberg, Chem. Rev., 1989, 89, 1433 CrossRef
;
(c) W. Cabri and I. Candiani, Acc. Chem. Res., 1995, 28, 2 CrossRef CAS
;
(d) G. T. Crisp, Chem. Soc. Rev., 1998, 27, 427 RSC
;
(e) I. P. Beletskaya and A. V. Cheprakov, Chem. Rev., 2000, 100, 3009 CrossRef CAS PubMed
;
(f) A. F. Littke and G. C. Fu, Angew. Chem., Int. Ed., 2002, 41, 4176 CrossRef CAS
.
-
N. J. Lawrence, in Preparation of Alkenes: a Practical Approach, ed. J. M. J. Williams, Oxford University Press, Oxford, UK, 1995 Search PubMed
.
-
(a) T. Mizoroki, K. Mori and A. Ozaki, Bull. Chem. Soc. Jpn., 1971, 44, 581 CrossRef CAS
;
(b) R. F. Heck and J. P. Nolley, J. Org. Chem., 1972, 37, 2320 CrossRef CAS
;
(c) X.-F. Wu, P. Anbarasan, H. Neumann and M. Beller, Angew. Chem., Int. Ed., 2010, 49, 9047 CrossRef CAS PubMed
.
-
(a) Q.-Y. Chen and Z.-Y. Yang, Tetrahedron Lett., 1986, 27, 1171 CrossRef CAS
;
(b) T. M. Gøgsig, A. T. Lindhardt, M. Dekhane, J. Grouleff and T. Skrydstrup, Chem.–Eur. J., 2009, 15, 5950 CrossRef PubMed
.
- For reviews, see:
(a) J. G. Taylor, A. M. Moro and C. R. D. Correia, Eur. J. Org. Chem., 2011, 1403 CrossRef CAS
;
(b) A. Roglans, A. Pla-Quintana and M. Moreno-Maňas, Chem. Rev., 2006, 106, 4622 CrossRef CAS PubMed
. For recent examples, see:
(c) F. Le Callonnec, E. Fouquet and F.-X. Felpin, Org. Lett., 2011, 13, 2646 CrossRef CAS PubMed
;
(d) E. W. Werner and M. S. Sigman, J. Am. Chem. Soc., 2011, 133, 9692 CrossRef CAS PubMed
.
-
(a) M. Miura, H. Hashimoto, K. Itoh and M. Nomura, Tetrahedron Lett., 1989, 30, 975 CrossRef CAS
;
(b) S. R. Dubbaka and P. Vogel, Chem.–Eur. J., 2005, 11, 2633 CrossRef CAS PubMed
.
-
(a) C.-M. Andersson and A. Hallberg, J. Org. Chem., 1988, 53, 235 CrossRef CAS
;
(b) M. S. Stephan, A. J. J. M. Teunissen, G. K. M. Verzijl and J. G. de Vries, Angew. Chem., Int. Ed., 1998, 37, 662 CrossRef CAS
;
(c) L. J. Gooßen, J. Paetzold and L. Winkel, Synlett, 2002, 1721 CrossRef
;
(d) L. J. Gooßen and J. Paetzold, Angew. Chem., Int. Ed., 2002, 41, 1237 CrossRef
;
(e) L. J. Gooßen and J. Paetzold, Angew. Chem., Int. Ed., 2004, 43, 1095 CrossRef PubMed
;
(f) T. Sugihara, T. Satoh and M. Miura, Tetrahedron Lett., 2005, 46, 8269 CrossRef CAS
.
- For reviews, see:
(a) B. Karimi, H. Behzadnia, D. Elhamifar, P. F. Akhavan, F. K. Esfahani and A. Zamani, Synthesis, 2010, 1399 CrossRef CAS
;
(b) R. Rossi, F. Bellina and M. Lessi, Synthesis, 2010, 4131 CrossRef CAS
;
(c) J. Le Bras and J. Muzart, Chem. Rev., 2011, 111, 1170 CrossRef CAS PubMed
.
- For reviews, see:
(a) J. Le Bras and J. Muzart, Chem. Rev., 2011, 111, 1170 CrossRef CAS PubMed
;
(b) C. Jia, T. Kitamura and Y. Fujiwara, Acc. Chem. Res., 2001, 34, 633 CrossRef CAS PubMed
;
(c) I. Moritani and Y. Fujiwara, Synthesis, 1973, 524 CrossRef CAS
. For recent examples, see:
(d) K. M. Engle, D.-H. Wang and J.-Q. Yu, Angew. Chem., Int. Ed., 2010, 49, 6169 CrossRef CAS PubMed
;
(e) Y. Lu, D.-H. Wang, K. M. Engle and J.-Q. Yu, J. Am. Chem. Soc., 2010, 132, 5916 CrossRef CAS PubMed
;
(f) K. M. Engle, D.-H. Wang and J.-Q. Yu, J. Am. Chem. Soc., 2010, 132, 14137 CrossRef CAS PubMed
;
(g) D.-H. Wang, K. M. Engle, B.-F. Shi and J.-Q. Yu, Science, 2010, 327, 315 CrossRef CAS PubMed
;
(h) M. Ye, G.-L. Gao and J.-Q. Yu, J. Am. Chem. Soc., 2011, 133, 6964 CrossRef CAS PubMed
;
(i) T. Nishikata and B. H. Lipshutz, Org. Lett., 2010, 12, 1972 CrossRef CAS PubMed
;
(j) H.-X. Dai, A. F. Stepan, M. S. Plummer, Y.-H. Zhang and J.-Q. Yu, J. Am. Chem. Soc., 2011, 133, 7222 CrossRef CAS PubMed
;
(k) C. Huang, B. Chattopadhyay and V. Gevorgyan, J. Am. Chem. Soc., 2011, 133, 12406 CrossRef CAS PubMed
;
(l) S. Cui, L. Wojtas and J. C. Antilla, Org. Lett., 2011, 13, 5040 CrossRef CAS PubMed
;
(m) M. Yu, Z. Liang, Y. Wang and Y. Zhang, J. Org. Chem., 2011, 76, 4987 CrossRef CAS PubMed
;
(n) X. Liu and K. K. Hii, J. Org. Chem., 2011, 76, 8022 CrossRef CAS PubMed
;
(o) A. García-Rubia, M. A. Fernández-Ibáñez, R. G. Arrayás and J. C. Carretero, Chem.–Eur. J., 2011, 17, 3567 CrossRef PubMed
;
(p) A. García-Rubia, B. Urones, R. G. Arrayás and J. C. Carretero, Angew. Chem., Int. Ed., 2011, 50, 10927 CrossRef PubMed
.
-
(a) A. G. Myers, D. Tanaka and M. R. Mannion, J. Am. Chem. Soc., 2002, 124, 11250 CrossRef CAS PubMed
;
(b) D. Tanaka and A. G. Myers, Org. Lett., 2004, 6, 433 CrossRef CAS PubMed
;
(c) D. Tanaka, S. P. Romeril and A. G. Myers, J. Am. Chem. Soc., 2005, 127, 10323 CrossRef CAS PubMed
;
(d) P. Hu, J. Kan, W. Su and M. Hong, Org. Lett., 2009, 11, 2341 CrossRef CAS PubMed
;
(e) S.-L. Zhang, Y. Fu, R. Shang, Q.-X. Guo and L. Liu, J. Am. Chem. Soc., 2010, 132, 638 CrossRef CAS PubMed
;
(f) Z. Fu, S. Huang, W. Su and M. Hong, Org. Lett., 2010, 12, 4992 CrossRef CAS PubMed
.
- For selected references:
(a) C. S. Cho and S. Uemura, J. Organomet. Chem., 1994, 465, 85 CrossRef CAS
;
(b) X. Du, M. Suguro, K. Hirabayashi and A. Mori, Org. Lett., 2001, 3, 3313 CrossRef CAS PubMed
;
(c) Y. C. Jung, R. K. Mishra, C. H. Yoon and K. W. Jung, Org. Lett., 2003, 5, 2231 CrossRef CAS PubMed
;
(d) M. M. S. Andappan, P. Nilsson and M. Larhed, Chem. Commun., 2004, 218 RSC
;
(e) K. S. Yoo, C. H. Yoon and K. W. Jung, J. Am. Chem. Soc., 2006, 128, 16384 CrossRef CAS PubMed
;
(f) J. Ruan, X. Li, O. Saidi and J. Xiao, J. Am. Chem. Soc., 2008, 130, 2424 CrossRef CAS PubMed
;
(g) J. Limdh, J. Sävmarker, P. Nilsson, P. J. R. Sjçberg and M. Larhed, Chem.–Eur. J., 2009, 15, 4630 CrossRef PubMed
;
(h) Y. Leng, F. Yang, K. Wei and Y. Wu, Tetrahedron, 2010, 66, 1244 CrossRef CAS
;
(i) E. W. Werner and M. S. Sigman, J. Am. Chem. Soc., 2010, 132, 13981 CrossRef CAS PubMed
;
(j) Y. Liu, D. Li and C.-M. Park, Angew. Chem., Int. Ed., 2011, 50, 7333 CrossRef CAS PubMed
;
(k) A. L. Gottumukkala, J. F. Teichert, D. Heijneen, N. Eeisink, S. V. Dijk, C. Ferrer, A. V. D. Hoogenband and A. J. Minnaard, J. Org. Chem., 2011, 76, 3498 CrossRef CAS PubMed
;
(l) M. Khoobi, M. Alipour, S. Zareei, F. Jafarpour and A. Shafiee, Chem. Commun., 2012, 48, 2985 RSC
;
(m) Z. He, S. Kirchberg, R. Fröhlich and A. Studer, Angew. Chem., Int. Ed., 2012, 51, 3699 CrossRef CAS PubMed
;
(n) L. Zhang, C. Dong, C. Ding, J. Chen, W. Tang, H. Li, L. Xu and J. Xiao, Adv. Synth. Catal., 2013, 355, 1570 CrossRef CAS
;
(o) L. Meng, C. Liu, W. Zhang, C. Zhou and A. Lei, Chem. Commun., 2014, 50, 1110 RSC
;
(p) J. Zheng, L. Huang, C. Huang, W. Wu and H. Jiang, J. Org. Chem., 2015, 80, 1235 CrossRef CAS PubMed
.
- A. Inoue, H. Shinokubo and K. Oshima, J. Am. Chem. Soc., 2003, 125, 1484 CrossRef CAS PubMed
.
-
(a) K. Garves, J. Org. Chem., 1970, 35, 3273 CrossRef CAS
;
(b) G.-W. Wang and T. Miao, Chem.–Eur. J., 2011, 17, 5787 CrossRef CAS PubMed
.
- X. Zhou, J. Luo, J. Liu, S. Peng and G.-J. Deng, Org. Lett., 2011, 13, 1432 CrossRef CAS PubMed
.
- M.-K. Zhu, J.-F. Zhao and T.-P. Loh, Org. Lett., 2011, 13, 6308 CrossRef CAS PubMed
.
- Y.-H. Su, Z. Wu and S.-K. Tian, Chem. Commun., 2013, 49, 6528 RSC
.
- Y.-G. Zhang, X.-L. Liu, Z.-Y. He, X.-M. Li, H.-J. Kang and S.-K. Tian, Chem.–Eur. J., 2014, 20, 2765 CrossRef CAS PubMed
.
-
(a) T. Taniguchi, A. Idota and H. Ishibashi, Org. Biomol. Chem., 2011, 9, 3151 RSC
;
(b) X. Li, X. Xu and C. Zhou, Chem. Commun., 2012, 48, 12240 RSC
;
(c) F.-L. Yang, X.-T. Ma and S.-K. Tian, Chem.–Eur. J., 2012, 18, 1582 CrossRef CAS PubMed
;
(d) R. Singh, D. S. Raghuvanshi and K. N. Singh, Org. Lett., 2013, 15, 4202 CrossRef CAS PubMed
;
(e) F.-L. Yang and S.-K. Tian, Angew. Chem., Int. Ed., 2013, 52, 4929 CrossRef CAS PubMed
;
(f) N. Singh, R. Singh, D. S. Raghuvanshi and K. N. Singh, Org. Lett., 2013, 15, 5874 CrossRef CAS PubMed
;
(g) W. Wei, C. Liu, D. Yang, J. Wen, J. You, Y. Suo and H. Wang, Chem. Commun., 2013, 49, 10239 RSC
;
(h) X. Li, X. Xu, P. Hu, X. Xiao and C. Zhou, J. Org. Chem., 2013, 78, 7343 CrossRef CAS PubMed
;
(i) X. Li, X. Xu and Y. Tang, Org. Biomol. Chem., 2013, 11, 1739 RSC
;
(j) S.-R. Guo, W.-M. He, J.-N. Xiang and Y.-Q. Yuan, Chem. Commun., 2014, 50, 8578 RSC
;
(k) T.-T. Wang, F.-X. Wang, F.-L. Yang and S.-K. Tian, Chem. Commun., 2014, 50, 3802 RSC
;
(l) F.-L. Yang, F.-X. Wang, T.-T. Wang, Y.-J. Wang and S.-K. Tian, Chem. Commun., 2014, 50, 2111 RSC
;
(m) X. Kang, R. Yan, G. Yu, X. Pang, X. Liu, X. Li and L. Xiang, J. Org. Chem., 2014, 79, 10605 CrossRef CAS PubMed
;
(n) X. Zhao, L. Zhang, T. Li, G. Liu, H. Wang and K. Lu, Chem. Commun., 2014, 50, 13121 RSC
;
(o) Y. Yang, L. Tang, S. Zhang, X. Guo, Z. Zha and Z. Wang, Green Chem., 2014, 16, 4106 RSC
;
(p) Q. Tian, P. He and C. Kuang, Org. Biomol. Chem., 2014, 12, 6349 RSC
;
(q) X. Li, Y. Xu, W. Wu, C. Jiang, C. Qi and H. Jiang, Chem.–Eur. J., 2014, 20, 7911 CrossRef CAS PubMed
;
(r) S. Tang, Y. Wu, W. Liao, R. Bai, C. Liu and A. Lei, Chem. Commun., 2014, 50, 4496 RSC
;
(s) X. Li, X. Shi, M. Fang and X. Xu, J. Org. Chem., 2013, 78, 9499 CrossRef CAS PubMed
;
(t) W. Zhang, K. Li and B. Zhao, J. Chem. Res., 2014, 38, 269 CrossRef CAS
;
(u) J. Wang, C. Xu, S. Wei, B. Zhao and K. Cheng, Chin. J. Org. Chem., 2014, 34, 767 CrossRef CAS
.
-
(a) F.-L. Yang, X.-T. Ma and S.-K. Tian, Chem.–Eur. J., 2012, 18, 1582 CrossRef CAS PubMed
;
(b) B. Liu, J. Li, F. Song and J. You, Chem.–Eur. J., 2012, 18, 10830 CrossRef CAS PubMed
;
(c) X. Yu, X. Li and B. Wan, Org. Biomol. Chem., 2012, 10, 7479 RSC
;
(d) O. Y. Yuen, C. M. So, W. T. Wong and F. Y. Kwong, Synlett, 2012, 23, 2714 CrossRef CAS
;
(e) W. Chen, H. Chen, F. Xiao and G.-J. Deng, Org. Biomol. Chem., 2013, 11, 4295 RSC
;
(f) W. Zhang, B. Zhao and K. Li, J. Chem. Res., 2013, 37, 674 CrossRef CAS
;
(g) H. Miao, F. Wang, S. Zhou, G. Zhang and Y. Li, Org. Biomol. Chem., 2015, 13, 4647 RSC
;
(h) S. Zhong, C. Sun, S. Dou and W. Liu, RSC Adv., 2015, 5, 27029 RSC
.
- For oxidation of reduced palladium by molecular oxygen is the rate limiting step for the Pd(OAc)2/DMSO system catalyzed oxidative amination of olefins, see:
(a) S. R. Fix, J. L. Brice and S. S. Stahl, Angew. Chem., Int. Ed., 2002, 41, 164 CrossRef CAS
; for Pd(OAc)2/pyridine catalyst for aerobic alcohol oxidation, see:
(b) B. A. Steinhoff and S. S. Stahl, Org. Lett., 2002, 4, 4179 CrossRef CAS PubMed
;
(c) B. A. Steinhoff, S. R. Fix and S. S. Stahl, J. Am. Chem. Soc., 2002, 124, 766 CrossRef CAS PubMed
;
(d) B. A. Steinhoff, L. A. Guzei and S. S. Stahl, J. Am. Chem. Soc., 2004, 126, 11268 CrossRef CAS PubMed
, and reference therein.
- Only poor to fair yields could be observed when non-terminal and aliphatic alkenes were used as substrates in this oxidative Heck reaction. The isolation of the desired products from the complicated reaction mixtures containing the homo-coupling by-products and the regioisomers were unsuccessful.
Footnote |
† Electronic supplementary information (ESI) available. See DOI: 10.1039/c6ra03188a |
|
This journal is © The Royal Society of Chemistry 2016 |
Click here to see how this site uses Cookies. View our privacy policy here.